Abstract
Background
Malaria in pregnancy is associated with considerable morbidity and mortality. Regular surveillance of artemisinin-based combination therapy tolerance, or molecular makers of resistance, is vital for effective malaria treatment, control and eradication programmes. Plasmodium falciparum multiple drug resistance-1 gene (Pfmdr1) N86Y mutation is associated with reduced susceptibility to lumefantrine. This study assessed the prevalence of Pfmdr1 N86Y in Brazzaville, Republic of Congo.
Methods
A total 1001 of P. falciparum-infected blood samples obtained from asymptomatic malaria pregnant women having a normal child delivery at the Madibou Integrated Health Centre were analysed. Pfmdr1 N86Y genotyping was conducted using PCR-restriction fragment length polymorphism.
Results
The wild type Pfmdr1 N86 allele was predominant (> 68%) in this study, whereas a few isolates carrying the either the mutant allele (Pfmdr1 86Y) alone or both alleles (mixed genotype). The dominance of the wildtype allele (pfmdr1 N86) indicates the plausible decline P. falciparum susceptibility to lumefantrine.
Conclusion
This study gives an update on the prevalence of Pfmdr1 N86Y alleles in Brazzaville, Republic of Congo. It also raises concern on the imminent emergence of resistance against artemether–lumefantrine in this setting. This study underscores the importance to regular artemether–lumefantrine efficacy monitoring to inform the malaria control programme of the Republic of Congo.
Similar content being viewed by others
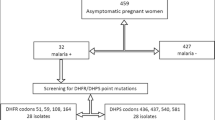
Background
Plasmodium falciparum malaria among pregnant women is a major public health concern in sub-Saharan Africa. Pregnant women have substantial risks and malaria in pregnant women are related to preterm delivery, intrauterine growth restriction, low birth weight and maternal anaemia. The World Health Organization (WHO) recommends the use of intermittent preventive treatment (IPTp) with sulfadoxine-pyrimethamine (SP) for pregnant women and also for infants (IPTi) [1]. Currently, artemisinin-based combination therapy (ACT) is the first-line treatment for P. falciparum uncomplicated malaria. Resistance against chloroquine (CQ) and its successor, sulfadoxine-pyrimethamine, had devasting consequences in sub-Saharan Africa in 1990s and 2000, particularly among children below 5 years [2].
After the introduction of ACT, malaria mortality and morbidity has globally declined until 2015. Even though ACT is still efficacious, there is sensitive concern on the potential spread of artemisinin-resistant P. falciparum parasites from Southeast Asia to sub-Saharan Africa, reminiscent of the spread of CQ and SP resistance [3,4,5,6,7]. The WHO recommends routine surveillance of anti-malarial drug efficacy once every 2 years. However, the efforts to monitor the emergence and spread of anti-malarial drug resistance in resource-limited settings are hampered due to high clinical trial costs. Molecular surveillance of distinct point mutation(s) in P. falciparum genes linked to anti-malarial treatment failure offers a cost-effective tool to monitor spatial and temporal emergence and spread of resistant parasites. High prevalence of gene mutations associated with CQ (P. falciparum chloroquine transporter, Pfcrt) and SP (P. falciparum dihydrofolate reductase gene; Pfdhfr, and P. falciparum dihydropteroate synthase; Pfdhps) resistance informed, in part, the decision to replace these anti-malarial drugs with ACT, including in the Republic of Congo [8,9,10,11].
The P. falciparum multidrug resistance 1 protein (PfMDR1), also known as P-glycoprotein homologue, is a transmembrane protein of the P. falciparum digestive vacuole (DV) [12]. It is involved in the transport of substrates into digestive vacuole of the parasite, including anti-malarial drugs [13]. Distinct changes in the sequence and/or amplification of the copy number of the Pfmdr1 gene alters P. falciparum susceptibility to several anti-malarial drugs [14].
In particular, the Pfmdr1 N86Y single nucleotide polymorphism (SNP) has been implicated in P. falciparum resistance to chloroquine and amodiaquine [15]. Pfmdr1 N86Y is mostly abundant in African settings. High prevalence of Pfmdr1 86 N and 86Y alleles is currently being driven by ACT-linked P. falciparum selection. Previous studies have shown that parasites carrying Pfmdr1 N86 are less susceptible to lumefantrine [16, 17], artemether-lumefantrine (AL) selects for Pfmdr1 86 N, whereas artesunate-amodiaquine (ASAQ) and piperaquine is selective for Pfmdr1 86Y [16, 18,19,20]. Since this phenomenon indicates potential decline of malaria parasite sensitivity or increased tolerance to ACT partner drugs, Pfmdr1 N86Y genotyping has been proposed as a useful marker to guide rotation of ACT medicines in a given geographical area [20, 21].
The present study aimed to genotype and to determine the prevalence of Pfmdr1 N86Y in Brazzaville, Republic of Congo among pregnant women using maternal peripheral, placental, and cord blood. The study aims to provide factual data as a useful measure for the refinement and adaption of the current malaria treatment policy with the long-term goal of reducing malaria in the Republic of Congo.
Methods
Sample collection
This study analysed a total of 101 matched blood samples (maternal peripheral, placenta, and cord blood) collected from pregnant women with asymptomatic malaria who had a normal child delivery at the Madibou Integrated Health Center, Brazzaville, between March 2014 and April 2015 [21]. The study was conducted in Brazzaville, the capital of the Republic of Congo with 1.8 million inhabitants [22]. Malaria transmission in this area is perennial with P. falciparum being the predominant Plasmodium species [22, 23]. AL and ASAQ are the first-line and second-line anti-malarial drugs for uncomplicated P. falciparum malaria in the Republic of Congo, respectively [24].
Pfmdr1 genotyping
Total genomic DNA was isolated using QIAamp DNA Mini Kit (Qiagen, Hilden, Germany) according to the manufacturer’s instructions. Amplification of P. falciparum merozoite surface protein 2 gene (Pfmsp2) was used to determine P. falciparum multiplicity of infection (MOI), as described previously [25]. Nested-PCR followed by a restriction fragment length polymorphism (PCR–RFLP) were used to genotype Pfmd1 N86Y, as described earlier [26]. In brief, Pfmdr1 primary and nested PCRs were amplified by adding 2 µl DNA template into a PCR master mix (50 µl) containing 1X PCR buffer, 2.8 mM MgCl2, 200 µM dNTPs, 5 pM of each primer, 1UTaq DNA polymerases (Qiagen, Hilden, Germany). The primer pairs for the primary PCR were A1 (5′-CGGAGTGACCAAATCTGGGA-3′) and A3 (5′-GGGAATCTGGTGGTAACAGC-3′) and for the secondary PCR were A2 (5′-TTGAAGAACAGAAATTACATGATGA-3′) and A4 (5′-AAAGATGGTAACCTCAGTATCAAAGAAGAG-3′). The thermal cycler conditions were as follows: initial denaturation at 94 °C for 2 min, followed by 40 cycles at 94 °C for 1 min, 45 °C for 1 min, 72 °C for 1 min and a final extension at 72 °C for 5 min. The secondary reaction was amplified using the product of the primary reaction as a template. DNA extracted P. falciparum laboratory strains (3D7 and Dd2) and PCR grade water were used as positive and negative controls, respectively.
The Pfmdr1 N86Y mutation was identified by digesting Pfmdr1 A2/A4 secondary PCR products (10 µl) using ApoI (New England Biolabs Inc., Ipswich, Massachusetts, USA) restriction enzyme for 15 min at 50 °C following manufacturer’s instructions. The resulting DNA fragments were separated and resolved by gel electrophoresis on a 2% agarose gel stained with SYBR green at 100 V for 45 min. ApoI digests Pfmdr1PCR product when Pfmdr1N86 (wild type allele) is present. The PCR amplification was performed three consecutive times for a given sample in order to get a successful amplification. Also, the nested PCR products were subjected to RFLP using ApoI twice (with independent PCR products) to reconfirm the Pfmdr1 N86Y alleles. Additionally, few random samples were chosen and were subjected to sanger sequencing.
Data analyses
Chi square and Fisher exact tests were applied to compare the proportions of Pfmdr1 N86Y alleles in this study. The statistical significance was set at p-value< 0.05.
Ethical considerations
This study was approved by the Institutional Ethics Committee of Fondation Congolaise pour la Recherche Médicale, FCRM, Brazzaville, Republic of Congo. Written informed consent was obtained from all participants before samples collection. The objectives of the study including the study procedures, sample to be taken, study benefits, potential risks and discomforts were explained. Newly opened needle and syringe were used for each subject.
Results
The baseline characteristics of participants recruited in this study is summarized in Table 1. The mean age of participants was 23.7 ± 5.75 years. Overall, 24% of the pregnant women did not take intermittent preventive treatment during pregnancy and most of the participants (70%) had > 1 parity. Of the 101 matched samples analysed in this study, Pfmdr1 was successfully amplified in 59 (58%), 38 (38%) and 21 (21%) maternal peripheral blood, placental blood and cord blood samples, respectively. Figure 1 shows an electrophoresis gel of PCR products before and after digestion with specific enzyme restriction. Pfmsp2 genotyping showed mean multiplicity of infection (MOI) was 1.06 ± 0.24. High prevalence of Pfmdr1 wild type allele (N86) was observed among the different sample types. Pfmdr1 N86 was present in 70% (41/59), 58% (22/38) and 86% (18/21) of the peripheral blood, placenta blood and cord blood samples, respectively. The remaining samples had the mutant allele (Pfmdr186Y). Three peripheral blood samples, two placental blood samples and one cord blood sample had both the wild type and mutant Pfmdr1 N86Y alleles. The Pfmdr1 N86 allele prevalence was similar (p-value> 0.05) among different sample types, parity and the number of intermittent preventive treatment during pregnancy (Table 2).
Discussion
Anti-malarial drug resistance is a major obstacle in malaria reduction/eradication globally. Molecular surveillance is important to identify resistant phenotypes and to constantly monitor for any anti-malarial drug resistance. This study was set out to determine the prevalence of Pfmdr1 N86Y mutation among pregnant women having a normal child delivery at the Madibou Integrated Health Centre, Brazzaville Republic of Congo. The Pfmsp2 gene used to determine MOI showed mean multiplicity of infection as 1.06 ± 0.24. In areas with constant transmission of malaria, MOI may increase as immunity develops. MOI in pregnant women is a factor for the acquisition and maintenance of immunity against malaria. In this study, only using msp2 genotyping, only one set of parasite clones were predominantly present among the pregnant women investigated. However, there are possibilities that these individuals may harbour more than one parasite, and this could be explained only by additional msp1 genotyping for K1, MAD20, and RO33 alleles.
The frequency of Pfmdr1 86Y (mutated allele) in this study was lower than previously estimated in this setting in 2010 (73%) and in 2015 (27%) [27, 28]. These findings are also comparable to Pfmdr1 86Y allele (23%) global frequency and most parts of Africa (17 to 24%), except Central Africa, where high resistant allele frequency (44%) has been observed [29]. In Southeast Asia, however, the frequency of Pfmdr1 86Y is much lower than observed in this study whereas it has almost reached fixation in Papua New Guinea [29].
Pfmdr1 N86Y mutation is known to modulate P. falciparum susceptibility to various anti-malarial drugs by regulating the influx of the drugs into the parasite’s digestive vacuole. Previous studies have shown that parasite carrying this mutation are less susceptible to 4-aminoquinolines, namely chloroquine, amodiaquine and piperaquine, in vitro [30] and increase the risk of chloroquine or amodiaquine therapeutic failure [15]. On the other hand, the Pfmdr1 86Y mutation enhance malaria parasite susceptibility to lumefantrine, mefloquine and the active derivative of artemisinin, dihydroartemisinin [30]. The converse impact of Pfmdr1 N86Y on P. falciparum response to longer-acting partner drugs of ACT implies that wide spread use of AL and ASAQ, particularly in Africa, exert opposite selection pressure on P. falciparum populations and allele frequency [31, 32].
Changes in malaria treatment policies greatly influence the frequency of mutations that modulate P. falciparum susceptibility to anti-malarial drugs, including Pfmdr1 N86Y [31]. The introduction of ACT in the early 2000s and cessation of chloroquine use in the 1990s led to drastic changes in Pfmdr1 N86Y allele frequency in various malaria-endemic settings [27, 33, 34]. For instance, the frequency of Pfmdr186Y has declined dramatically, in favour of Pfmdr1 N86, in countries, where AL is used as the first − line treatment for malaria. The increase in Pfmdr1 N86 allele frequency is faster when AL is used compared to ASAQ usage [31]. In areas where ASAQ is the primary treatment for malaria, the decline of Pfmdr1 86Y allele frequency is slow owing to the reduced susceptibility of parasites carrying this mutation to amodiaquine.
Previous studies demonstrate that parasites carrying Pfmdr1 N86 tolerate higher lumefantrine levels and have short-time to reinfection or recrudescence in patients with high lumefantrine concentration following AL treatment [16, 17]. Even though there is no evidence directly linking Pfmdr1 N86 to AL treatment failure and AL is still highly efficacious, parasite tolerance to lumefantrine is a clear warning sign for plausible emergence of resistance against AL. In this context, Pfmdr1 86 N can be used to track lumefantrine selective pressure in a given area [17]. The findings show that Pfmdr1N 86 allele is approaching fixation in the Republic of Congo and could provide the genetic background needed for the emergence of resistance against lumefantrine threatening AL usefulness in this setting. However, this possibility could be averted by concurrent use of AL and ASAQ as first-line treatment for uncomplicated P. falciparum malaria. Such a strategy is supported by evidence showing the opposite effect of both Pfmdr1 N86Y alleles on P. falciparum susceptibility to AL and ASAQ [18].
Conclusion
This study offers an update on the frequency of Pfmdr1 N86Y alleles in Brazzaville, Republic of Congo and provides evidence supporting the concomitant deployment or rotation of AL and ASAQ as the primary treatment for uncomplicated P. falciparum malaria. This will be helpful to halt any further selection of Pfmdr1 alleles that dampen parasite susceptibility and safeguard AL efficacy.
Availability of data and materials
All raw data provided in this work are available upon request to the corresponding author.
Abbreviations
- ACT:
-
Artemisinin-based combined therapy
- AL:
-
Artemether–lumefantrine
- ASAQ:
-
Artesunate–amodiaquine
- CQ:
-
Chloroquine
- FCRM:
-
Fondation Congolaise pour la Recherche Médicale
- IPTp:
-
Intermittent preventive treatment for pregnant women
- IPTi:
-
Intermittent preventive treatment for infants
- SNP:
-
Single nucleotide polymorphism
- SP:
-
Sulfadoxine-pyrimethamine
References
WHO. Guidelines for the treatment of malaria. 3rd ed. Geneva: World Health Organization; 2015.
Trape JF. The public health impact of chloroquine resistance in Africa. Am J Trop Med Hyg. 2001;64:12–7.
Conrad MD, Rosenthal PJ. Antimalarial drug resistance in Africa: the calm before the storm? Lancet Infect Dis. 2019;19:338–51.
Hamilton WL, Amato R, van der Pluijm RW, Jacob CG, Quang HH, Thuy-Nhien NT, et al. Evolution and expansion of multidrug-resistant malaria in southeast Asia: a genomic epidemiology study. Lancet Infect Dis. 2019;19:943–51.
Ariey F, Witkowski B, Amaratunga C, Beghain J, Langlois AC, Khim N, et al. A molecular marker of artemisinin-resistant Plasmodium falciparum malaria. Nature. 2014;505:50–5.
Ashley EA, Dhorda M, Fairhurst RM, Amaratunga C, Lim P, Suon S, et al. Spread of artemisinin resistance in Plasmodium falciparum malaria. N Engl J Med. 2014;371:411–23.
Mita T, Tanabe K, Kita K. Spread and evolution of Plasmodium falciparum drug resistance. Parasitol Int. 2009;58:201–9.
Nsimba B, Malonga DA, Mouata AM, Louya F, Kiori J, Malanda M, et al. Efficacy of sulfadoxine/pyrimethamine in the treatment of uncomplicated Plasmodium falciparum malaria in Republic of Congo. Am J Trop Med Hyg. 2004;70:133–8.
Mayengue PI, Ndounga M, Davy MM, Tandou N, Ntoumi F. In vivo chloroquine resistance and prevalence of the pfcrt codon 76 mutation in Plasmodium falciparum isolates from the Republic of Congo. Acta Trop. 2005;95:219–25.
Ndounga M, Tahar R, Basco LK, Casimiro PN, Malonga DA, Ntoumi F. Therapeutic efficacy of sulfadoxine-pyrimethamine and the prevalence of molecular markers of resistance in under 5-year olds in Brazzaville, Congo. Trop Med Int Health. 2007;12:1164–71.
Koukouikila-Koussounda F, Bakoua D, Fesser A, Nkombo M, Vouvoungui C, Ntoumi F. High prevalence of sulphadoxine-pyrimethamine resistance-associated mutations in Plasmodium falciparum field isolates from pregnant women in Brazzaville, Republic of Congo. Infect Genet Evol. 2015;33:32–6.
Cowman AF, Karcz S, Galatis D, Culvenor JG. A P-glycoprotein homologue of Plasmodium falciparum is localized on the digestive vacuole. J Cell Biol. 1991;113:1033–42.
Reiling SJ, Rohrbach P. Monitoring PfMDR1 transport in Plasmodium falciparum. Malar J. 2015;14:270.
Price RN, Uhlemann AC, Brockman A, McGready R, Ashley E, Phaipun L, et al. Mefloquine resistance in Plasmodium falciparum and increased pfmdr1 gene copy number. Lancet. 2004;364:438–47.
Picot S, Olliaro P, de Monbrison F, Bienvenu AL, Price RN, Ringwald P. A systematic review and meta-analysis of evidence for correlation between molecular markers of parasite resistance and treatment outcome in falciparum malaria. Malar J. 2009;8:89.
Otienoburu SD, Maiga-Ascofare O, Schramm B, Jullien V, Jones JJ, Zolia YM, et al. Selection of Plasmodium falciparum pfcrt and pfmdr1 polymorphisms after treatment with artesunate-amodiaquine fixed dose combination or artemether-lumefantrine in Liberia. Malar J. 2016;15:452.
Sisowath C, Stromberg J, Mårtensson A, Msellem M, Obondo C, Bjorkman A, et al. In vivo selection of Plasmodium falciparum pfmdr1 86 N coding alleles by artemether-lumefantrine (Coartem). J Infect Dis. 2005;191:1014–7.
Sondo P, Derra K, Diallo Nakanabo S, Tarnagda Z, Kazienga A, Zampa O, et al. Artesunate-amodiaquine and artemether-lumefantrine therapies and selection of Pfcrt and Pfmdr1 alleles in Nanoro, Burkina Faso. PLoS One. 2016;11:e0151565.
Malmberg M, Ferreira PE, Tarning J, Ursing J, Ngasala B, Bjorkman A, et al. Plasmodium falciparum drug resistance phenotype as assessed by patient antimalarial drug levels and its association with pfmdr1 polymorphisms. J Infect Dis. 2013;207:842–7.
Taylor AR, Flegg JA, Holmes CC, Guérin PJ, Sibley CH, Conrad MD, et al. Artemether-lumefantrine and dihydroartemisinin-piperaquine exert inverse selective pressure on Plasmodium falciparum drug sensitivity-associated haplotypes in Uganda. Open Forum Infect Dis. 2017;4:ofwo229.
Gil JP, Krishna S. Pfmdr1 (Plasmodium falciparum multidrug drug resistance gene 1): a pivotal factor in malaria resistance to artemisinin combination therapies. Expert Rev Anti Infect Ther. 2017;15:527–43.
Trape JF, Peelman P, Morault-Peelman B. Criteria for diagnosing clinical malaria among a semi-immune population exposed to intense and perennial transmission. Trans R Soc Trop Med Hyg. 1985;79:435–42.
Trape JF, Zoulani A. Malaria and urbanization in central Africa: the example of Brazzaville. Part II: results of entomological surveys and epidemiological analysis. Trans R Soc Trop Med Hyg. 1987;81(Suppl 2):10–8.
Ministère de la Santé et de la Population. Politique nationale de lutte contre le paludisme. Brazzaville: Republic of Congo; 2006.
Gueye NSG, Ntoumi F, Vouvoungui C, Kobawila SC, Nkombo M, Mouanga AM, et al. Plasmodium falciparum merozoite protein-1 genetic diversity and multiplicity of infection in isolates from Congolese children consulting in a pediatric hospital in Brazzaville. Acta Trop. 2018;183:78–83.
Mungthin M, Khositnithikul R, Sitthichot N, Suwandittakul N, Wattanaveeradej V, Ward SA, et al. Association between the pfmdr1 gene and in vitro artemether and lumefantrine sensitivity in Thai isolates of Plasmodium falciparum. Am J Trop Med Hyg. 2010;83:1005–9.
Koukouikila-Koussounda F, Jeyaraj S, Nguetse CN, Nkonganyi CN, Kokou KC, Etoka-Beka MK, et al. Molecular surveillance of Plasmodium falciparum drug resistance in the Republic of Congo: four and nine years after the introduction of artemisinin-based combination therapy. Malar J. 2017;16:155.
ACT Partner Drug Molecular Surveyor. (https://www.wwarn.org/tracking-resistance/act-partner-drug-molecular-surveyor).
Plasmodium falciparum Community Project. (www.malariagen.net/projects/p-falciparum-community-project).
Veiga MI, Dhingra SK, Henrich PP, Straimer J, Gnadig N, Uhlemann AC, et al. Globally prevalent PfMDR1 mutations modulate Plasmodium falciparum susceptibility to artemisinin-based combination therapies. Nat Commun. 2016;7:11553.
Okell LC, Reiter LM, Ebbe LS, Baraka V, Bisanzio D, Watson OJ, et al. Emerging implications of policies on malaria treatment: genetic changes in the Pfmdr-1 gene affecting susceptibility to artemether-lumefantrine and artesunate-amodiaquine in Africa. BMJ Glob Health. 2018;3:e000999.
Venkatesan M, Gadalla NB, Stepniewska K, Dahal P, Nsanzabana C, Moriera C, et al. Polymorphisms in Plasmodium falciparum chloroquine resistance transporter and multidrug resistance 1 genes: parasite risk factors that affect treatment outcomes for P. falciparum malaria after artemether-lumefantrine and artesunate-amodiaquine. Am J Trop Med Hyg. 2014;91:833–43.
Hayward R, Saliba KJ, Kirk K. Pfmdr1 mutations associated with chloroquine resistance incur a fitness cost in Plasmodium falciparum. Mol Microbiol. 2005;55:1285–95.
Moyeh MN, Njimoh DL, Evehe MS, Ali IM, Nji AM, Nkafu DN, et al. Effects of drug policy changes on evolution of molecular markers of Plasmodium falciparum resistance to chloroquine, amodiaquine, and sulphadoxine-pyrimethamine in the South West Region of Cameroon. Malar Res Treat. 2018;2018:7071383.
Acknowledgements
We thank the study participants and their parents. We thank Dr Mandingha Kosso Etoka-Beka, Dr. Nerly Gampio Gueye for her contribution to sample collection and the staff of the Madibou integrated health center. FN, TPV, FKK are members of CANTAM (EDCTP-RegNet2015-1045) and PANDORA-ID-Net (Grant Agreement RIA2016E-1609) networks.
Disclaimer
The findings and conclusions in this paper are those of the authors and do not necessarily represent the views of the funding agency.
Funding
This study was funded by CANTAM (EDCTP-RegNet 2015-1045) and TOTAL E&P Congo.
Author information
Authors and Affiliations
Contributions
LRD-Y performed all the lab analyses, FK-K supervised the lab work, JCV was responsible of statistical analysis, AA and AL participated in the interpretation of the results, DN and TPV reviewed and edited the manuscript. FN supervised the overall work. All the authors contributed in drafting the paper. All authors read and approved the final manuscript.
Corresponding author
Ethics declarations
Ethical approval and consent to participate
This study was approved by the Institutional Ethics Committee of Fondation Congolaise pour la Recherche Médicale, FCRM, Brazzaville, Republic of Congo. Written informed consent was obtained from all participants before samples collection.
Consent for publication
Not applicable.
Competing interests
Authors declare they have no competing interests.
Additional information
Publisher's Note
Springer Nature remains neutral with regard to jurisdictional claims in published maps and institutional affiliations.
Rights and permissions
Open Access This article is licensed under a Creative Commons Attribution 4.0 International License, which permits use, sharing, adaptation, distribution and reproduction in any medium or format, as long as you give appropriate credit to the original author(s) and the source, provide a link to the Creative Commons licence, and indicate if changes were made. The images or other third party material in this article are included in the article's Creative Commons licence, unless indicated otherwise in a credit line to the material. If material is not included in the article's Creative Commons licence and your intended use is not permitted by statutory regulation or exceeds the permitted use, you will need to obtain permission directly from the copyright holder. To view a copy of this licence, visit http://creativecommons.org/licenses/by/4.0/. The Creative Commons Public Domain Dedication waiver (http://creativecommons.org/publicdomain/zero/1.0/) applies to the data made available in this article, unless otherwise stated in a credit line to the data.
About this article
Cite this article
Dossou-Yovo, L.R., Ntoumi, F., Koukouikila-Koussounda, F. et al. Molecular surveillance of the Pfmdr1 N86Y allele among Congolese pregnant women with asymptomatic malaria. Malar J 19, 178 (2020). https://doi.org/10.1186/s12936-020-03246-0
Received:
Accepted:
Published:
DOI: https://doi.org/10.1186/s12936-020-03246-0