Abstract
To what extent is the relative biodiversity of some flagship conservation sites a result of differential attention? Knysna estuarine bay is the topmost ranked South African estuary for conservation importance and biodiversity. It is also one of the most intensively studied, and hence differential sampling effort could partly be responsible for its apparent relative richness. To assess the extent to which this might be true, identical sampling area, effort and methodology were employed to compare the benthic macrofauna of one specific major Knysna habitat (Zostera capensis seagrass beds) with equivalent ones in two nearby lesser-studied estuaries, the Keurbooms/Bitou and Swartvlei. Investigation showed all three localities to share a common species pool, but different elements of it dominated the shared habitat type in each. The seagrass and adjacent sandflat macrobenthos proved just as biodiverse in unprotected Keurbooms/Bitou as in the Protected Area of Knysna, but that in Swartvlei (also a Protected Area) was impoverished in comparison, presumably consequent on mouth closure and the prevailing lower salinity. Despite marked geomorphological and hydrological differences, all three estuaries share a suite of unusual faunal elements and such particularly close faunal similarity suggests the importance of historical biogeographic processes. The analysis emphasises the need for caution when assessing the relative conservation importance or other merits of different individual systems in a data-limited environment.
Similar content being viewed by others
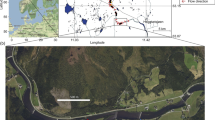
Avoid common mistakes on your manuscript.
Introduction
The Swartvlei, Knysna and Keurbooms/Bitou estuaries discharge into the Indian Ocean along a 50 km warm-temperate stretch of the south coast of South Africa’s Western Cape between 34° 02′ S, 22° 48′ E and 34° 03′ S, 23° 23′ E (Fig. 1). They share the distinction of being the only known localities of the rare and endangered fully-estuarine seahorse, Hippocampus capensis (Teske et al. 2003). Not unrelatedly, all three also support important beds of Cape dwarf-eelgrass, Zostera (Zosterella) capensis. Globally this seagrass is classified as vulnerable but in South Africa it too is endangered (Skowno et al. 2019), and between them these estuaries currently support 60% of country’s acreage of Z. capensis, with Knysna in particular being its South African stronghold (Adams 2016). For this and other reasons, all three estuaries are in the top twenty most ecologically significant South African ones (out of 290+), Knysna being ranked 1st, Swartvlei 6/7th, and Keurbooms 16-18th (Turpie et al. 2002; Turpie 2004; Turpie and Clark 2007). Swartvlei and Knysna lie within the Garden Route National Park and hence have Protected Area status; the joint Keurbooms/Bitou estuary, however, receives no formal protection, although it is managed under the prescriptions of the National Estuarine Management Protocol (Western Cape Government 2018).
Part of the south coast of the Western Cape, South Africa, showing the location of the three estuaries under study (Google Earth Pro satellite image © 2020 Landsat/Copernicus), and within each estuary detail of its mouth region with the sites investigated marked (Google Earth Pro satellite images © 2023 Maxar Technologies). Scale lines on the individual estuaries are all 2 km
Although geographically adjacent, their present nature, size, shape and hydrography differ quite markedly. Knysna is a large estuarine bay (sensu van Niekerk et al. 2020a) with a water surface area of some 19 km2 and a narrow but permanently open connection to the ocean through a sandstone gorge (Russell et al. 2010; Whitfield et al. 2023). Like most South African estuaries (Whitfield 2005), freshwater inflow is relatively small, and the whole system is dominated by sea water, with a very large tidal prism, although it is a drowned river-valley estuary in the terminology of Whitfield and Elliott (2011). Swartvlei is a small (2 km2), narrow channel linking a large estuarine lake to the ocean (Hill 1975), and its mouth is often closed as a result of longshore sand-movement at times of low freshwater discharge (Whitfield et al. 1983; Whitfield 1989). The system is essentially the mouth of a bahira lagoon (Tagliapietra et al. 2009) formed when rising sea levels broke through the 200 m high pre-existing coastal dune system to flood part of the hinterland (Illenberger 1996; Bateman et al. 2011). The slightly larger (3 km2) joint estuary of the Keurbooms and Bitou rivers is a typical bar-built system mainly in the form of a longshore back-barrier coastal lagoon (Schumann 2021), and hence is usually termed the Keurbooms Lagoon. Its relatively large freshwater input maintains an open mouth which migrates in position along the 4 km long enclosing sand bar (Duvenage & Morant 1984; Adams et al. 2015).
Work in 1947 as part of a survey of all South Africa’s major estuaries led to the Knysna estuarine bay early being regarded as supporting the richest estuarine fauna in the country, both in terms of numbers of individual animals and number of species (Day et al. 1951). Accordingly, over the next 70 + years it received very much more scientific attention than neighbouring Swartvlei or Keurbooms/Bitou (see summary in Whitfield et al. 2023), and it remains top of the overall national estuarine biodiversity rankings (jointly with the Swartkops, Klein and Kosi estuaries) (Turpie 2004; Turpie and Clark 2007). To some extent the pre-eminence of Knysna’s biodiversity could therefore be a reflection of years of differential research effort (Awad et al. 2002; Azovsky 2011). It is possible, however, to place the apparent relative richness of Knysna in a truer context by comparing individual shared habitat types in comparably-sized locations in the three estuaries, using sampling regimes of equivalent nature, intensity, and extent. The present study seeks to achieve this, based on historical datasets recently collected from Z. capensis localities along the main channel at Knysna (see Barnes and Claassens 2020; and Barnes 2022: Mendeley Data, VI, doi: https://doi.org/10.17632/nmwsrpd738.1 and https://doi.org/10.17632/nj2mvv8fn5.1), and equivalent new data collected from Knysna and from a comparable locality at each of Swartvlei and Keurbooms/Bitou in 2023. The null hypothesis that there are no differences in abundance and biodiversity between a given characteristic and important habitat type in a representative locality in the flagship Knysna estuary and in comparable sites of equivalent area in the other two ‘lesser’ estuaries was tested using the benthic macrofauna as an example.
Of particular biodiversity interest are a number of ‘Knysna specialities’—species that dominate that estuarine system but appear neither in the authoritative Day (1981a) Appendix 9.1 list of the 120 invertebrates that are the “characteristic macrobenthic species in estuaries of southern Africa” nor in the Knox et al. (2004) list of species occurring in the other South African estuaries along the 1000 km of warm-temperate coast centred on Knysna, i.e. between Cape Point (34° 22′ S, 18° 30′ E) and the Mbashe estuary (32° 17′ S, 28° 54′ E). Such seagrass-associated Knysna specialities include the three endemic South African microgastropods Alaba pinnae (in densities of up to 39,000 m−2), ‘Assiminea’ capensis (sensu Criscione and Ponder 2013) (up to 32,000 m−2), and ‘Hydrobia’ knysnaensis (sensu Wilke et al. 2013) (up to 16,000 m−2), and the polychaete Paradoneis lyra capensis (up to 7000 m−2) [‘Assiminea’ and ‘Hydrobia’ are here names of convenience, both species await the description of the new genera required for them, and in the case of ‘Assiminea’ possibly a new subfamily or family (Fukuda and Ponder 2010)]. A second null hypothesis that the dominance of these species is in fact not restricted to Knysna but extends to all three local estuaries was therefore also examined.
Materials and methods
Protocol and study areas
Previous work on dwarf-eelgrass benthic macrofaunas within individual paralic systems has demonstrated a high degree of assemblage constancy across quite wide ranges of environmental conditions (Barnes 2022, 2023; Barnes et al. 2022). Hence precise site location within the estuaries concerned was not considered to be too critical a potential variable. Two of the localities recently surveyed by Barnes and Claassens (2020) and Barnes (2022), ‘Brenton’ (34° 03.5′ S, 23° 02.0′ E) and ‘Steenbok’ (34° 03.7′ S, 23° 02.9′ E), appear representative of the non-backwater regions of Knysna’s marine basin [i.e. areas subject to the ‘marine-bay hydrological and ecological regime’ of Largier et al. (2000) with their marine or aeolian sandy sediments (Reddering and Esterhuysen 1987)], and they support the nearest permanent seagrass beds to the estuarine mouth along Knysna’s main channel. These represented Knysna, in the case of assemblage metrics by the average of their values.
Localities at Swartvlei and Keurbooms/Bitou selected for comparison were also located in equivalent positions along their main channels in the most marine influenced zone of each estuary and in positions sheltered from high-velocity tidal fluxes of water through the mouth but nevertheless closest to it; these were at 34° 01.5′ S, 22° 48.6′ E and 34° 02.7′ S, 23° 22.5′ E, respectively. At each locality, the Zostera capensis beds were sampled during the austral summer (January–March) at two replicate sites, > 100 m apart. During the sampling period, the mouth of the Swartvlei estuary was closed, as is often the case (Kok and Whitfield 1986; Russell and Randall 2017), and therefore although subtidal seagrass beds could be sampled, there was no intertidal zone. At Keurbooms/Bitou, however, it was possible to sample at two tidal-heights: one some 1.5 m below low water spring (LWS) tide level, and the other intertidally at LWS. In addition, adjacent intertidal areas of bare sediment near LWS were sampled for comparison with the equivalent Brenton data of Barnes (2022) and new data were collected to represent the Steenbok site. Samples were taken at least 2 m away from habitat interfaces to avoid any possible edge effects (Nakaoka 2005; Barnes and Hamylton 2016), and each seagrass site was represented by 16 replicate cores at ~ 1 m intervals parallel to the water line and each bare-sand one by 20 such samples. These replicate sample numbers at individual Swartvlei and Keurbooms/Bitou sites were fixed to conform to those in the specific historical Knysna datasets being compared, so that all site comparisons were based on the same number of samples and total sampled area. Subtidal sampling was effected by high-tide snorkelling, and intertidal sites were sampled during low tide.
Also to conform to the dataset previously collected from Knysna, individual cores were of 0.0054 m2 area and 10 cm depth, thus collecting the smaller and most numerous members of the macrofauna that constitute the large majority of invertebrate biodiversity, at least insofar as molluscs are representative (Albano et al. 2011). All samples were collected during daylight hours, the intertidal ones just before tidal ebb from the area of shore concerned whilst it was still covered by > 15 cm of water, and were gently sieved on site through 710 µm mesh. Retained material from each core: (1) was placed in a large polythene bag of local estuary water within which all seagrass was shaken vigorously to dislodge all but sessile animals and then discarded; (2) was then re-sieved and transported immediately to a local laboratory, and (3) was there placed in a 30 × 25 cm translucent dish over a LED light pad in which the living fauna was located by visual inspection. Samples from the bare sediment were treated similarly except that stage 1 was omitted. As macrofaunal assemblage characteristics may vary with the degree of seagrass cover (McCloskey and Unsworth 2015), all seagrass samples were taken from areas with coverages greater than the McKenzie (2003) maximum 75% standard for estuarine dwarf eelgrass. The earlier samples from Knysna were previously collected and sorted in identical fashion and taken during the same months of the year.
Because of taxonomic uncertainty, including amongst numerically-dominant local animal groups (see e.g. Simon et al. 2022), identification of collected fauna was generally attempted only to morphologically-based operational taxonomic units (‘morphotaxa’), an appropriate procedure to detect spatial patterns of numbers of taxa and their differential abundance (Dethier and Schoch 2006; Gerwing et al. 2020). Although this incurs a risk of failing to distinguish any closely similar species, experience of taxonomic resolution/sufficiency in equivalent soft-sediment macrobenthic studies (e.g. Warwick 1988; Tataranni et al. 2009) indicates that operating at various levels from species up to family all produce similar conclusions. Wherever possible, however, all animals of particular interest were identified to those species currently recognised. Nomenclature below is as given by the World Register of Marine Species (WoRMS, www.marinespecies.org, accessed March 2023), except in respect of ‘Assiminea’ capensis (see Barnes 2018) which is listed in WoRMS as Rissoa capensis and as a taxon inquirendum [= the ‘A’. aff capensis of Miranda et al. 2014, but not their ‘A’. cf capensis]. Sessile and mobile species can differentially influence spatial patterns of biodiversity (Davidson et al. 2004), and this study excluded any sessile or semi-sessile animals that had become detached from the seagrass leaves during sampling.
Data analysis
Numbers per unit area (per core, site, and locality) of each component zoobenthic morphotaxon were subjected to similarity analysis, and assemblage metrics were derived and compared via PAST 4.11 software (Hammer et al. 2001) or Microsoft Excel for Mac 16.71 with the StatPlus:mac Pro 8.0.4 add-on, all metrics being based on animal abundance. Ranking of dominant species was determined by the Barnes (2014) index of numerical importance (INI), and differences in rank orders were tested using the Friedman non-parametric ANOVA.
Univariate metrics assessed were those known to have a major influence on local-scale biodiversity patterns (Blowes et al. 2022); i.e. (1) overall faunal numbers, (2) observed numbers of morphotaxa, i.e. Hill’s N0 [‘species density’ sensu (Gotelli and Colwell 2001)], and (3) relative evenness (= equitability) of taxon abundances (Pielou’s J). In addition, (4) the Gatti et al. (2020) AED biodiversity index incorporating Hill’s N0, N1 and N2 metrics was also assessed, as was (5) patchiness in assemblage abundance (as estimated by Lloyd’s Ip).
Multivariate comparison of assemblage composition used hierarchical clustering analysis of S17 Bray–Curtis similarity carried out on standardised taxon abundances (i.e. all samples adjusted to the same total abundance to reflect solely differential taxonomic composition), one- and two-way ANOSIM and PerMANOVA, SIMPER, and IndVal, all with 9999 permutations. In order to compare numbers of species in seagrass and bare sand, those in the sand were reduced to numbers per 32 cores by sample rarefaction using Mao’s tau (Colwell et al. 2004). β diversity was assessed as the beta-2 index of Harrison et al. (1992), which uses presence-absence data, ranges from 0 (complete similarity) to 1 or 100% (complete dissimilarity), and is a ‘narrow sense’ non-directional index in the terminology of Koleff et al. (2003); all species represented only by a singleton individual in the entire database under analysis were omitted from β diversity calculations because of the computational errors associated with the occurrence of numerous rare species (Colwell and Coddington 1994).
Results
Subtidal seagrass
The benthic macrofauna of subtidal seagrass beds nearest to the mouth of the Knysna system was dominated by gastropod molluscs (particularly Alaba), as were those of Swartvlei and Keurbooms/Bitou although in those localities the dominant gastropod taxa were ‘Assiminea’ and ‘Hydrobia’ at Swartvlei, and Nassarius and Turritella at Keurbooms/Bitou (Table 1). Thus there were very low levels of compositional similarity between the three (PerMANOVA F = 45.4; P < < 0.0001) (Table 2). Compositional differences between replicate sites within localities were also marked (mean Bray–Curtis 0.61), although much less than between localities. Overall, the faunal composition in Swartvlei and Keurbooms/Bitou was more similar to that in the upper estuarine and middle lagoonal reaches of the Knysna system, as documented by Barnes and Claassens (2020), than to the marine zone near its mouth (Fig. 2), although Bray–Curtis similarity values concerned were all low to very low. Their assemblage metrics were also divergent (Table 3); and there were significant differences both in overall abundance (ANOVA F2,93 = 20.8; P < < 0.0001) and in number of species per core sample (ANOVA F2,93 = 19.7; P < < 0.0001; all Tukey pairwise test P ≤ 0.02). The only similarity was in the overall macrofaunal abundance per sample at Swartvlei and Keurbooms/Bitou (Tukey pairwise P > 0.5).
Pattern of standardised Bray–Curtis similarity of the subtidal seagrass macrobenthos in the Swartvlei, Keurbooms/Bitou, and Knysna estuaries (data from different reaches of the Knysna estuary after Barnes and Claassens 2020)
Intertidal seagrass
The intertidal macrobenthos of seagrass beds nearest to the mouth of the Knysna system was co-dominated by a range of taxa including the Alaba that dominated subtidal regions and the nereid Simplisetia. Nereid polychaetes and, to a considerably lesser degree, Alaba also dominated that at Keurbooms/Bitou (Table 1). As with the subtidal seagrass fauna, however, there were very low levels of compositional similarity between the two localities (PerMANOVA F = 22.5; P < < 0.0001) (Table 2). Nevertheless, the rank orders in importance of their total assemblage components (marginally) did not differ (Friedman P = 0.06); their assemblage metrics were comparable (Table 3); and there was no difference in overall macrobenthic abundance per core sample (ANOVA F1,62 = 1.05; P > 0.3). Numbers of species per core, however, was significantly smaller at Keurbooms/Bitou (ANOVA F1,62 = 10.6; P < 0.002). Compositional differences between replicate sites within localities were also marked (Bray–Curtis 0.45–0.52), although less than between localities. In a Keurbooms/Bitou vs Knysna comparison, locality and seagrass water depth (i.e. intertidal vs subtidal) were subequally important influences on the associated macrofaunal assemblages (two-way ANOSIM: locality R = 0.36, P < 0.0001; water depth R = 0.44, P < 0.0001).
Adjacent intertidal bare sand
Areas of bare sand adjacent to the intertidal seagrass beds investigated were visibly structured by Upogebia burrows, except at Steenbok. Their faunal assemblages were overwhelmingly dominated numerically by the gastropod mollusc ‘Assiminea’ in Keurbooms/Bitou, and in Knysna by the polychaetes Paradoneis, Simplisetia, Orbinia and Prionospio (Table 1). Simplisetia and Prionospio were also important components of the Keurbooms/Bitou sands but there were very low levels of compositional similarity between the two localities (PerMANOVA F = 25.7; P < < 0.0001) (Table 2). Nevertheless, the rank orders in importance of their total assemblage components did not differ (Friedman P = 0.18); their assemblage metrics were comparable (Table 3); and there was (marginally) no difference in overall macrobenthic abundance per core sample (ANOVA F1,78 = 3.69; P = 0.06). Numbers of species per core, however, was significantly smaller at Keurbooms/Bitou (ANOVA F1,78 = 9.2; P < 0.004). Compositional differences between replicate sites within localities were also marked, particularly in Knysna (Bray–Curtis 0.21).
Overall comparisons
Table 2 summarises a marked divergence in assessment of degrees of compositional similarity using different measures: presence/absence-based β-diversity indicates high levels of similarity between the three estuarine faunas, whereas abundance-based ANOSIM, PerMANOVA and Bray–Curtis suggest the opposite. This is consequent on all three faunas sharing a very similar taxonomic composition (and see Fig. 3), but showing marked differences in the relative abundance of individual shared taxa, both of morphospecies (Table 1) and of higher groupings (Fig. 4). In terms of major taxa, worms dominated the species lists, although in most cases gastropods dominated in numbers of individuals; crustaceans were relatively poorly represented on both counts. The total number of species present at the equal-area localities in Knysna and Keurbooms/Bitou (total in all three habitat types) was: 84 (mean of Brenton and Steenbok data) and 81, respectively (AED biodiversity indices of 88 and 85). All species recorded from Keurbooms/Bitou are also known to occur in Knysna except for an unidentified small anthurid isopod (? Haliophasma sp.) and a polyclad flatworm; likewise all subtidal species from Swartvlei also occur in Knysna. Overall, ‘Knysna specialities’ formed three of the five most abundant intertidal soft-sediment species in the investigated regions of the Knysna and Keurboom/Bitou estuaries, contributing > 55% of total invertebrate numbers.
Stacked-column diagrams illustrating the taxonomic composition of the macrobenthic faunas of subtidal and intertidal seagrass and of associated areas of intertidal bare sediment: 1. Proportions of component morphospecies in different major taxa at the various localities. Note the marked similarity across all localities and habitats
Stacked-column diagrams illustrating the taxonomic composition of the macrobenthic faunas of subtidal and intertidal seagrass and of associated areas of intertidal bare sediment: 2. Proportional abundance of animals in different major taxa at the various localities. Gastropods averaged 61% of all individuals except in the intertidal seagrass at Keurbooms/Bitou and the adjacent sand at Knysna which were dominated by worms (72% and 91% of the totals respectively)
Ordination of the complete quantitative datasets from Knysna and Keurbooms/Bitou (Fig. 5) reveals a close similarity between the two Knysna subtidal localities (together with a lesser affinity with one of the associated intertidal seagrass sites), but no other patterns of similarity at a level greater than a Bray–Curtis value ≈0.6. The two subtidal sites at Keurbooms/Bitou (again together with one of the associated intertidal seagrass sites), and the two bare sand sites at the same locality do each show some degree of affinity, but the remaining intertidal sand and seagrass sites show no clear assortment based on habitat type or locality. Comparably, two-way ANOSIM of the dataset shows that both estuary (R = 0.70, P < 0.0001) and habitat type (R = 0.64, P < 0.0001) have a similar effect on assemblage composition.
Assemblage structure as represented by species abundance distributions, in which abundance is assessed as numerical importance values (INI) (standardised as per Passy 2016) (Fig. 6), shows that notwithstanding the differences in the nature of their dominant species (Table 1), the intertidal seagrass assemblages of Knysna and Keurbooms/Bitou share an effectively identical structure. The location of its curve and the few datapoints also highlight the poverty of the Swartvlei subtidal seagrass system.
Discussion
Comparisons of estuarine biodiversities
Although the high levels of local-scale heterogeneity seen in other seagrass systems (Alsaffar et al. 2020; Barry et al. 2021; Barnes 2023) occurred in these three adjacent estuaries too, their macrobenthic soft-sediment faunas are clearly nested within the same species pool—a pool furthermore that shows marked local geographical turnover from that generally regarded as being characteristic of South African estuaries. At their near-mouth localities surveyed here, this common pool was dominated by the five species Alaba pinnae, Simplisetia erythraeensis, ‘Assiminea’ capensis, Nassarius kraussianus and Paradoneis lyra capensis, which together comprised > 70% of total assemblage numbers, and of which only Simplisetia and Nassarius are more widely typical of South African estuaries (Day 1981a). Again as seen elsewhere (e.g. Barry et al. 2021), differences between the three systems were then mainly a matter of variation in the relative importance of individual members of their shared numerically-dominant taxa.
Swartvlei is the most species poor, as might be expected in a temporarily closed system (Perissinotto et al. 2010; van Niekerk et al. 2020a), although its low overall abundance is somewhat unusual (Teske and Wooldridge 2001; Froneman 2018). Knysna supported the greatest subtidal macrofaunal abundances although intertidal densities were much more finely balanced, and in respect of species density Keurbooms/Bitou ranked subequally with its more-intensively-studied neighbour for both seagrass and bare-sand biodiversity. Such would not have been concluded on the basis of the few earlier accounts of its benthos which suggested a relatively restricted fauna, totalling only some 40 soft-sediment species for the whole Keurbooms/Bitou system (Turpie et al. 2004). Indeed in its assessment summary, the 2018 management plan for the estuary (Western Cape Government 2018: p. 28) comments that on the basis of the available information both the abundance and species density of the benthic invertebrates are low and well below levels that might be expected. In complete contrast, the present survey recorded 81 macrofaunal morphotaxa from within only a small section of the Keurbooms/Bitou estuary (< 500 m2), of which more than half were not previously known to occur there. The comparable samples from the two Knysna localities yielded a similar mean of 84 such taxa. Further, intertidal invertebrate densities at Keurbooms/Bitou were effectively the same as those in equivalent situations in Knysna, which generally supports some 4500–7000 m−2 (Barnes 2021).
The explanation of this disparity in estimations of the biodiversity supported by Keurbooms/Bitou can really only be insufficiency and/or inadequacy of earlier sampling; the one exception being that recently carried out in the region > 4 km upstream of the mouth (de Villiers et al. 2021)—a zone likely to support relatively low values of species richness on the basis of comparable work at Knysna (Barnes 2021). It is evident, for example, that the animals mentioned by Day (1981b) and Duvenage and Morant (1984) are all relatively large (Nassarius, Upogebia, Hymenosoma, Arenicola, etc.) and, historically, taxa of small body size have been greatly underestimated in South Africa (Griffiths et al. 2010). In terms of abundance, the seagrass and associated bare sediment at Knysna (Barnes et al. 2023), and at Keurbooms/Bitou too (this study), are dominated by tiny gastropods and polychaetes; species with a modal size within the range of 2–10 mm [Alaba < 10 mm; ‘Hydrobia’ < 4 mm; ‘Assiminea’ < 2.5 mm; Paradoneis < 10 mm]. Failure adequately to include these in analyses would certainly grossly underestimate abundance and biodiversity, and lead to a very false impression of local ecology and biodiversity.
An apparently distinctive ecological feature of the marine-influenced zone at Knysna is that areas of bare sandflat there may often support as much biodiversity as the adjacent seagrass beds: nearly as many species and equal or even greater macrofaunal abundance (Barnes and Barnes 2014; Barnes 2022). Although greater abundance than in the local dwarf eelgrass beds (there of Z. noltei) also typifies bare sediments in the Mediterranean Mistral Lagoon (Magni and Gravina 2023), this state contrasts with the situation in other regions of South Africa (Siebert and Branch 2006; Pillay et al. 2007; Pillay and Branch 2011) and indeed at many sites elsewhere in the world (Hemminga and Duarte 2000) where sandflats support much less abundance and far fewer species than seagrass beds, including those of other Zostera species [e.g. Z. muelleri in Queensland (Barnes and Barnes 2012)]. It has been suggested that this contrast results from differential levels of bioturbation of the sediment by callianassids at (the more marine) affected sites versus relatively benign upogebiids at (the more estuarine) non-affected ones (Barnes and Barnes 2014). The Keurbooms/Bitou sands were clearly structured by Upogebia but in contrast to Knysna faunal abundance in the bare sand was only some 60% of the values in the adjacent seagrass beds, whereas it was 94–103% of that at Brenton and Steenbok (Barnes 2022). The difference in species densities in sand and seagrass was similar in the two localities, however: 60% of the seagrass values in the Keurbooms/Bitou sands and 60–70% in Knysna (Barnes 2022). It was noticeable that the adjacent areas of sand at Knysna were extensive flats with shallow slopes, whereas those sampled in Keurbooms/Bitou were narrower and more steeply sloping fringes; whether this is significant is as yet unknown but such flats vs slopes dichotomy is known to affect nektonic ecology (Gross et al. 2019).
Distributions of the ‘Knysna specialities’
It was not apparent from earlier work that Knysna’s unusual suite of dominant species occurred more widely as abundant faunal elements, although their real distributions remain far from clear. In respect of the dominant microgastropods, the situation in other South African estuaries is unfortunately confused and confusing (Barnes 2018), not least because although whether those inhabiting seagrass and surrounding sediment are Assiminea, ‘Assiminea’ or ‘Hydrobia’ is obvious when they are alive, in common with some other very small gastropods (Kensley 1973) it is far from easy accurately to distinguish them if samples have been preserved because they cannot reliably be separated on shell characteristics. In most cases, published data from other localities were indeed based on preserved samples, and hence their generic (let alone specific) nature is uncertain. Species of true AssimineaFootnote 1—A. globulus and A. ovata in the nomenclature accepted by WoRMS—have long been known to be important elements in Cape estuaries (Day 1981a; Teske and Wooldridge 2001), although they characterise high shore levels and are very rarely encountered in or at the level of seagrass. Otherwise, all these estuarine microgastropods seem to be ecologically-similar browsers of microphytobenthic biofilms that cannot be differentiated in terms of preferred habitat or horizon in the inter- or subtidal zone either.
Neither Duvenage and Morant (1984) or Adams et al. (2015) recorded any of the dominant Knysna microgastropods from the Keurbooms/Bitou. Nor did Davies (1982) do so from Swartvlei, whilst Whitfield (1989) lumped all non-Alaba microgastropods together there but in any event found very few of them in total (≤ 1% of total biomass). De Villiers et al. (2021: Appendix A. Supplementary data) did record—and only recorded—‘Hydrobia’ knysnaensis from the Keurbooms arm of the joint Keurbooms/Bitou system. Nevertheless, the present data do show a common and consistent pattern of dominant microgastropod identity, abundance and distribution across the three estuaries. Alaba, ‘Assiminea’, ‘Hydrobia’ (and Assiminea) do clearly occur in all three, in some areas abundantly so: ‘Hydrobia’ is most in evidence upstream in Keurbooms (de Villiers et al. 2021) and Knysna (Barnes 2021), and downstream at Swartvlei when the mouth is closed and salinities there fall to some half that of sea water. Conversely, Alaba occurs downstream in all three, less so during mouth closure at Swarvlei, but abundantly in Knysna and (although previously unrecorded) in Keurbooms. ‘A.’ capensis, although also previously unrecorded, occurs in some numbers downstream in Swartvlei (up to 25,000 m−2) and Keurbooms (> 10,000 m−2), as well as in Knysna (Barnes 2021) (in densities of up to 32,000 m−2), and probably is present in upstream regions of the three estuaries as well [it certainly is in Knysna and was between the middle and upper reaches of Swartvlei in March 2005 (RSKB unpublished)]. In Keurbooms/Bitou it also proved the most numerous faunal component of the associated LWS intertidal sands [in the backwater channels at Knysna, it is also more numerous in bare sand than in adjacent seagrass (1.6–2.0×), whereas ‘Hydrobia’ is equally abundant in the two compartments (data of Barnes and Barnes 2014)]. Distributions of dominant species within Swartvlei are, of course, likely to change with state of the mouth, prolonged periods of closure resulting in lowered salinity and the consequent species turnover frequently seen elsewhere (Menegotto et al. 2019).
Two other dominant soft-sediment species have also been considered Knysna specialities. The small polychaete Paradoneis lyra capensis, previously regarded a Knysna endemic, was also present in both the inter- and subtidal samples at Keurbooms/Bitou, although never abundantly, but appears to occur nowhere else. The present surveys did not record the other, the small seagrass-associated form of the dwarf cushion-star Parvulastra exigua (20 mm) from either Swartvlei or Keurbooms/Bitou, although (for comparability with Knysna) sampling was restricted to regions near the mouth and P. exigua is not widespread or abundant in the comparable region at Knysna either. However, it is known to be an important member of both the Keurbooms/Bitou and Knysna fauna further upstream (Barnes 2021; de Villiers et al. 2021). The small polychaete Schistomeringos sp. (< 10 mm) which was a important element of the Knysna and Keurbooms/Bitou intertidal sands may also belong to this characteristic group of species in that it appears not previously to have been recorded in any other South African estuary (Global Biodiversity Information Facility and Ocean Biodiversity Information System, accessed March 2023). A number of species in other habitat types have also been considered to be ‘Knysna specialities’, including the seagrass-leaf-associated sedentary false-limpet Siphonaria compressa (the present survey did not include species firmly attached to the seagrass leaves). A 2008 search failed to find any living S. compressa in Keurbooms/Bitou (de Coito et al. 2023), but it may have been present there in the recent past (Herbert 1999).
Although it is the case that there are individual records of all these various species, except P. lyra capensis, from elsewhere along the southern coast of South Africa, they currently amount to only one or a few sites per species and never in large numbers. Notwithstanding their occasional presence elsewhere (and taking their apparent absences at face value), however, the observation that these unusual species are all present together in systems of such contrasting size, form and hydrographical features as Swartvlei, Knysna and Keurbooms/Bitou suggests the likelihood of historical biogeographic explanations of community composition rather than ecological ones such as particular association with estuarine-bay-like habitats (see Day 1967). Nevertheless, geophysical/geological evidence suggests that although a number of Western Cape estuaries were united before discharge to the sea at various points in the late Pleistocene, when the present coastline would have been some 80 km seawards of its present position (Spratt & Lisiecki 2016; Cooper et al. 2018), those of Swartvlei, Knysna and Keurbooms/Bitou are likely to have remained separate during most glacial phases, although maybe discharging into a single bay (Cawthra et al. 2020).
Conservation implications
Extending from the high-water neap-tide mark throughout the inter- and subtidal zone of the estuarine bay except near its mouth, the seagrass system at Knysna is not its only component habitat although it is the most extensive, productive, and economically valuable one. Further, granted the world-wide vulnerable status of Zostera capensis, it is also the habitat in particular need of conservation (Skowno et al. 2019). There is no reason, however, to consider the seagrass habitat there as a special case (except possibly the subtidal portions although data here are very scarce), and so the comparison undertaken here therefore indicates that Knysna’s individual habitat types are not necessarily richer than those elsewhere when compared on an equal-area basis. Nevertheless, Knysna is a relatively large body of water and its seagrass beds all interconnect effectively to form one single meadow. Local population losses in any specific area could therefore relatively easily be counterbalanced by immigration from outside that region. To that extent, it would be predicted to be less fragile than smaller estuaries such as Keurbooms/Bitou, although Keurbooms/Bitou has clearly managed to retain its singular biodiversity through time notwithstanding major episodes of freshwater flooding and other periods of environmental adversity (Western Cape Government 2018; Schumann 2021).
The present results from Keurbooms/Bitou are from but a single component region near the mouth, but again there is no reason to consider that the areas investigated there are in any way unrepresentative. In total over the last 15 years, > 200 macrobenthic invertebrate species have been recorded in the Knysna seagrass, although this is from > 2,500 core samples distributed over 25 times as many sites across the whole of that large estuarine bay (RSKB unpublished). A quarter of these species are very rare, i.e. are represented only by singletons or doubtletons in the 76,400 animals total, and the upper species quartile comprises 96.5% of faunal individuals. No corresponding data are available from Keurbooms/Bitou (the present samples being equivalent to only ≤ 3.0% of those from Knysna both in terms of core and of individual numbers), but its smaller size may result in a lesser total fauna because of area effects (Turpie et al. 2004; Loke et al. 2019). Some evidence, however, suggests that this will not necessarily be the case: regional differentiation of its seagrass fauna is evident from the contrasting upstream sites studied by de Villiers et al. (2021); similarities in their species abundance distributions suggest the same assemblage structure in the two systems with equally important proportions of rarities; and the near-mouth areas at Knysna are known to support > 90% of its overall macrobenthic seagrass species (Barnes 2013) and if the same applies to Keurbooms/Bitou too, the similar shared numbers of species per unit area near their mouths will equate to similar sized total species richness. Regardless of such considerations, conservation status of South African estuaries is based on a whole basket of factors, of which their supported invertebrate biodiversity is responsible only for < 7% of the overall score (Turpie et al. 2002; Turpie 2004) irrespective of the occurrence of species of considerable conservational interest or concern. Nevertheless, it is clear that any previously perceived relative poverty of the Keurbooms/Bitou benthic macrofauna (Turpie et al. 2004; Adams et al. 2015) was a false impression, and this emphasises the need for considerable caution when considering emergent inferences on relative biodiversity derived from sampling a number of systems to differing degrees in what van Niekerk et al. (2022) have termed a data-limited environment.
Seagrass meadows provide multiple important ecosystem services (Lima et al. 2023) yet unfortunately appear to have a “charisma gap” (Dennison 2009: p. 102) and do not attract the same attention as more high profile or ‘glamorous’ species or habitats. They continue to be allowed to decline, and even within conservation areas are often marginalised in management agendas (Cullen-Unsworth and Unsworth 2016; Unsworth et al. 2022). It has earlier been remarked (Barnes and Claassens 2020) that in Knysna greater conservation attention appears to be devoted to the widespread and abundant bait organism Upogebia than to the rare and threatened Zostera capensis amongst which it lives. Equivalently to that of the invertebrates, biodiversity of the aquatic and semi-aquatic vegetation only accounts for < 7% of Knysna’s conservation-importance score (Turpie et al. 2002; Turpie 2004), notwithstanding that the site supports the largest single stand remaining of the endangered Z. capensis. Elsewhere and for other species, such a situation has provided 100% of the justification for intensive protective measures (Watson et al. 2014), and improved protection at Knysna is certainly needed (Adams and van der Colff 2018).
Further, the Wilderness and Knysna sections of the Garden Route National Park are far from being wilderness, and, unlike other South African National Parks, that of the open-access, common-pool-resource Garden Route has a large human population, of which some 76,000 live on the shores of the Knysna estuarine bay (Western Cape Government 2021). The whole coastal area from Wilderness to Keurbooms contains four out of the five temperate South African estuaries with the highest tourism value (Turpie and Clark 2007), and Knysna in particular supports a flourishing recreational industry (Turpie and de Wet 2009) and subsistence fishery (Napier et al. 2009), both of which use the Z. capensis beds, legally and/or illegally, as a source of bait (Hodgson et al. 2000; Simon et al. 2019). The location of these beds therefore means that in practice conservation management is a complex compromise between the socio-economic requirements of the local and tourist human population and the desirability of protecting the resource, environment and any rare and/or endangered species (Roux et al. 2023). There is no doubt, however, that human exploitation for bait causes serious local damage to the threatened Z. capensis population (Barnes and Claassens 2020; Wasserman et al. 2023), as does human-induced nutrient enhancement of the water body, directly or via inflowing streams, and the resultant blooms of smothering green algae (Human et al. 2016; Claassens et al. 2020).
In comparison to other seagrasses, estuarine species like Z. capensis are at particularly high risk, since their habitat is the most threatened of all ecosystems in South Africa, again notwithstanding the great socio-economic benefit of estuarine ecosystem services to the country (van Niekerk et al. 2020b; Taljaard et al. 2023). Three-quarters of South Africa’s estuarine area is either critically endangered or endangered (Skowno et al. 2019). Like other wetlands, they are often viewed as wasteland that is best ‘reclaimed’ for human use (Wetlands International 2023). Extensive areas of Knysna estuarine bay have indeed been substantially modified by development (Russell et al. 2010: p. 16), including marina construction, major road and bridge/causeway building, hotel complexes, infilling, and induced siltation including from the building of structures such as golf courses on the surrounding hillsides. Almost 21 km of its shoreline is now artificial and 107 ha of natural habitat has been lost (Raw et al. 2020). However, many small to medium sized estuaries are still relatively healthy—Keurbooms/Bitou is in a higher Current Health Category (see Turpie et al. 2012a) than either Knysna or Swartvlei (Turpie et al. 2012b)—and these may prove vital in the conservation of estuarine faunal and floral biodiversity. They may not be perceived as meriting the same conservation attention as large flagship sites, but can shelter unanticipated and, at the moment at least, relatively unthreatened biodiversity.
Data availability
New data on which the work reported in this paper is based have been uploaded to Mendeley Data (doi: https://doi.org/10.17632/d7fmxgx95t/1).
Notes
Insofar as any South African species are true Assiminea (see Miranda et al. 2014).
References
Adams JB (2016) Distribution and status of Zostera capensis in South African estuaries—a review. S Afr J Bot 107:63–73. https://doi.org/10.1016/j.sajb.2016.07.007
Adams JB, van der Colff D (2018) Official interim publication of Zostera capensis red list assessment. Available at: http://opus.sanbi.org/jspui/bitstream/20.500.12143/8473/1/Zostera%20capensis_NationalRedListAssessment.pdf
Adams JB, Huizinga P, Lamberth S, Snow G, Taljaard S, Theron A, Turpie J, van Niekerk L, Wooldridge T (2015) Desktop re-evaluation of the 2008 EWR study on the Keurbooms Estuary. Department of Water and Sanitation Report RDM/WMA16/04/CON/0613.
Albano PG, Sabelli B, Bouchet P (2011) The challenge of small and rare species in marine biodiversity surveys: microgastropod diversity in a complex tropical coastal environment. Biodivers Conserv 20:3233–3237. https://doi.org/10.1007/s10531-011-0117-x
Alsaffar Z, Cúrdia J, Irigoien X, Carvalho S (2020) Composition, uniqueness and connectivity across tropical coastal lagoon habitats in the Red Sea. BMC Ecol 20:61. https://doi.org/10.1186/s12898-020-00329-z
Awad AA, Griffiths CL, Turpie JK (2002) Distribution of South African marine benthic invertebrates applied to the selection of priority conservation areas. Divers Distrib 8:129–145. https://doi.org/10.1046/j.1472-4642.2002.00132.x
Azovsky A (2011) Species-area and species-sampling effort relationships: disentangling the effects. Ecography 34:18–30. https://doi.org/10.1111/j.1600-0587.2010.06288.x
Barnes RSK (2013) Distribution patterns of macrobenthic biodiversity in the intertidal seagrass beds of an estuarine system, and their conservation significance. Biodivers Conserv 22:357–372. https://doi.org/10.1007/s10531-012-0414-z
Barnes RSK (2014) The nature and location of spatial change in species assemblages: a new approach illustrated by the seagrass macrofauna of the Knysna estuarine bay, South Africa. Trans R Soc S Afr 69:75–80. https://doi.org/10.1080/0035919X.2014.899277
Barnes RSK (2018) Little-known and phylogenetically obscure South African estuarine microgastropods (Mollusca: Truncatelloidea) as living animals. J Nat Hist 52:87–113. https://doi.org/10.1080/00222933.2017.1408867
Barnes RSK (2021) Patterns of seagrass macrobenthic biodiversity in the warm-temperate Knysna estuarine bay, Western Cape: a review. Aquat Ecol 55:327–345. https://doi.org/10.1007/s10452-021-09848-3
Barnes RSK (2022) Biodiversity differentials between seagrass and adjacent bare sediment change along an estuarine gradient. Estuar Coast Shelf Sci 274:107951. https://doi.org/10.1016/j.ecss.2022.107951
Barnes RSK (2023) Seagrass macrobenthic biodiversity does not vary in conformity with a leaky-lagoonal confinement gradient. Mar Environ Res 185:105897. https://doi.org/10.1016/j.marenvres.2023.105897
Barnes RSK, Barnes MKS (2012) Shore height and differentials between macrobenthic assemblages in vegetated and unvegetated areas of an intertidal sandflat. Estuar Coast Shelf Sci 106:112–120. https://doi.org/10.1016/j.ecss.2012.5.011
Barnes RSK, Barnes MKS (2014) Biodiversity differentials between the numerically-dominant macrobenthos of seagrass and adjacent unvegetated sediment in the absence of sandflat bioturbation. Mar Environ Res 99:34–43. https://doi.org/10.1016/j.marenvres.2014.05.013
Barnes RSK, Claassens L (2020) Do beds of subtidal estuarine seagrass constitute a refuge for macrobenthic biodiversity threatened intertidally? Biodivers Conserv 29:3227–3244. https://doi.org/10.1007/s10531-020-02019-0
Barnes RSK, Hamylton S (2016) On the very edge: faunal and functional responses to the interface between benthic seagrass and unvegetated-sand assemblages. Mar Ecol Progr Ser 553:33–48. https://doi.org/10.3354/meps11800
Barnes RSK, Claassens L, Seath J (2022) Where ecologically ‘tis better to go brown than green: enhanced seagrass macrobenthic biodiversity within the canals of a brownfield coastal marina. Biodivers Conserv 31:2981–2997. https://doi.org/10.1007/s10531-022-02468-9
Barnes RSK, Wooldridge TH, Hodgson AN (2023) Benthic and planktonic invertebrates of the Knysna Estuary. In: Whitfield AK, Breen CM, Read M (eds) Knysna Estuary—jewel of the garden route. Knysna Basin Project, Knysna, pp 143–181
Barry SC, Hyman AC, Jacoby CA, Reynolds LK, Kowalewski M, Frazer TK (2021) Variation in seagrass-associated macroinvertebrate communities along the Gulf Coast of peninsular Florida: an exploration of patterns and ecological consequences. Front Mar Sci 8:596966. https://doi.org/10.3389/fmars.2021.596966
Bateman MD, Carr AS, Dunajko AC, Murray-Wallace CV (2011) The evolution of coastal barrier systems: a case study of the middle-late Pleistocene wilderness barriers, South Africa. Quatern Sci Rev 30:63–81. https://doi.org/10.1016/j.quascirev.2010.10.003
Blowes SA, Daskalova GN, Dornelas M, Chase JM (2022) Local biodiversity change reflects interactions among changing abundance, evenness and richness. Ecology 103:e3820. https://doi.org/10.1002/ecy.3820
Cawthra H, Cowling RM, Andò S, Marean CW (2020) Geological and soil maps of the Palaeo-Agulhas plain for the last glacial maximum. Quatern Sci Rev 235:105858. https://doi.org/10.1016/j.quascirev.2019.07.040
Claassens L, Barnes RSK, Wasserman J, Lamberth SJ, Miranda NAF, van Niekerk L, Adams JB (2020) Knysna Estuary health: ecological status, threats and options for the future. Afr J Aquat Sci 45:65–82. https://doi.org/10.2989/16085914.2019.1672518
Colwell RK, Coddington JA (1994) Estimating terrestrial biodiversity through extrapolation. Phil Trans R Soc B Biol Sci 345:101–118. https://doi.org/10.1098/rstb.1994.0091
Colwell RK, Mao CX, Chang J (2004) Interpolating, extrapolating, and comparing incidence-based species accumulation curves. Ecology 85:717–2727. https://doi.org/10.1890/03-0557
Cooper JAG, Green AN, Compton JS (2018) Sea-level change in southern Africa since the last glacial maximum. Quatern Sci Rev 201:303–318. https://doi.org/10.1016/j.quascirev.2018.10.013
Criscione F, Ponder WF (2013) A phylogenetic analysis of rissooidean and cingulopsoidean families (Gastropoda: Caenogastropoda). Mol Phylogenet Evol 66:1075–1082. https://doi.org/10.1016/j.ympev.2012.11.026
Cullen-Unsworth LC, Unsworth RKF (2016) Strategies to enhance the resilience of the world’s seagrass meadows. J Appl Ecol 53:967–972. https://doi.org/10.1111/1365-2664.12637
Davidson IC, Crook AC, Barnes DKA (2004) Quantifying spatial patterns of intertidal biodiversity: is movement important? Mar Ecol 25:15–34. https://doi.org/10.1111/j.1439-0485.2004.00015.x
Davies BR (1982) Studies on the zoobenthos of some southern Cape coastal lakes. Spatial and temporal changes in the benthos of Swartvlei, South Africa, in relation to changes in the submerged littoral macrophyte community. J Limnol Soc S Afr 8:33–45. https://doi.org/10.1080/03779688.1982.9633232
Day JH (1967) The biology of Knysna Estuary, South Africa. In: Lauff GH (ed) Estuaries. American Association for the Advancement of Science, Washington DC, pp 397–407
Day JH (1981a) The estuarine fauna. In: Day JH (ed) Estuarine ecology with particular reference to Southern Africa. Balkema, Rotterdam, pp 147–178
Day JH (1981b) Summaries of current knowledge of 43 estuaries in southern Africa: Keurbooms Estuary. In: Day JH (ed) Estuarine ecology with particular reference to Southern Africa. Balkema, Rotterdam, pp 303–305
Day JH, Millard NAH, Harrison AD (1951) The ecology of South African estuaries. Part 3. Knysna: a clear open estuary. Trans R Soc S Afr 33:367–413. https://doi.org/10.1080/00359195109510891
de Coito PM, Emami-Khoyi A, Hedderson TA, Toonen RJ, Teske PR, Branch GM (2023) A critically endangered estuarine limpet’s only two populations are genomically and morphologically distinct. Aquat Conserv 2023:1–17. https://doi.org/10.1002/aqc.3993
de Villiers NM, Harasti D, Hodgson AN, Claassens L (2021) A comparison of the fauna in eelgrass and erosion control structures in a warm temperate South African estuary. Region Stud Mar Sci 44:101757. https://doi.org/10.1016/j.rmsa.2021.101757
Dennison WC (2009) Global trajectories of seagrasses, the biological sentinels of coastal ecosystems. In: Duarte CM et al (eds) Global loss of coastal habitats: rates, causes and consequences. BBVA Foundation, Bilbao, pp 89–106
Dethier MN, Schoch GC (2006) Taxonomic sufficiency in distinguishing natural spatial patterns on an estuarine shoreline. Mar Ecol Progr Seri 306:41–49. https://doi.org/10.3354/meps306041
Duvenage IR, Morant PD (1984) Estuaries of the cape, Part II. Synopses of available information on individual systems, Report 31: Keurbooms/Bitou System and Piesang. CSIR, Stellenbosch
Froneman PW (2018) The ecology and food-web dynamics of South African intermittently open estuaries. In: Fronemen PW (ed) Estuary. IntechOpen, Croatia, pp 85–98
Fukuda H, Ponder WF (2010) Bizarre unidentified rissooids occurring in tidal-flats of South Africa. In: PowerPoint presentation to the 2010 Meeting of the Malacological Society of Japan
Gatti RC, Amoroso N, Monaco A (2020) Estimating and comparing biodiversity with a single universal metric. Ecol Model 424:109020. https://doi.org/10.1016/j.ecolmodel.2020.109020
Gerwing TG, Cox K, Allen Gerwing AM, Campbell L, Macdonald T, Dudas SE, Juanes F (2020) Varying intertidal invertebrate taxonomic resolution does not influence ecological findings. Estuar Coast Shelf Sci 232:106516. https://doi.org/10.1016/j.ecss.2019.106516
Gotelli NJ, Colwell RK (2001) Quantifying biodiversity: procedures and pitfalls in the measurement and comparison of species richness. Ecol Lett 4:379–391
Griffiths CL, Robinson TB, Lange L, Mead A (2010) Marine biodiversity in South Africa: an evaluation of current states of knowledge. PLoS ONE 5(8):e12008. https://doi.org/10.1371/journal.pone.0012008
Gross C, Donoghue C, Pruitt C, Trimble AC, Ruesink JL (2019) Nekton community responses to seagrass differ with shoreline slope. Estuar Coast 42:1156–1168. https://doi.org/10.1007/s12237-019-00556-8
Hammer Ø, Harper DAT, Ryan PD (2001) PAST: paleontological statistics software package for education and data analysis. Palaeont Electron 4(1):9
Harrison S, Ross SJ, Lawton JH (1992) Beta diversity on geographic gradients in Britain. J Anim Ecol 61:151–158
Hemminga MA, Duarte CM (2000) Seagrass ecology. Cambridge University Press, Cambridge
Herbert DG (1999) Siphonaria compressa, South Africa’s most endangered marine mollusc. S Afr J Sci 95:77–79
Hill BJ (1975) The origin of Southern African coastal lakes. Trans R Soc S Afr 41:225–240. https://doi.org/10.1080/00359197509519440
Hodgson AN, Allanson BR, Cretchley R (2000) The exploitation of Upogebia africana (Crustacea: Thalassinidae) for bait in the Knysna Estuary. Trans R Soc S Afr 55:197–204. https://doi.org/10.1080/00359190009520444
Human LRD, Adams JB, Allanson BR (2016) Insights into the cause of an Ulva lactuca Linnaeus bloom in the Knysna Estuary. S Afr J Bot 107:55–62. https://doi.org/10.1016/j.sajb.2016.05.016
Illenberger WK (1996) The geomorphologic evolution of the Wilderness dune cordons, South Africa. Quatern Int 33:11–20. https://doi.org/10.1016/1040-6182(95)00099-2
Kensley B (1973) Sea-shells of Southern Africa—Gastropods. Maskew-Miller, Cape Town
Knox D, Clark B, Turpie J (2004) A field study of the invertebrate fauna of the warm temperate estuaries. In: Adams JB (ed), Contributions to information requirements for the implementation of resource directed measures for estuaries. Volume 1. Improving the biodiversity importance rating of South African estuaries. Report to the Water Research Commission No. 1247/01/04:45-79. ISBN 1-77005-267-4
Kok HM, Whitfield AK (1986) The influence of open and closed mouth phases on the marine fish fauna of the Swartvlei estuary. S Afr J Zool 21:309–315. https://doi.org/10.1080/02541858.1986.11448004
Koleff P, Gaston KJ, Lennon JL (2003) Measuring beta diversity for presence-absence data. J Anim Ecol 72:367–382. https://doi.org/10.1046/j.1365-2656.2003.00710.x
Largier JL, Attwood C, Harcourt-Baldwin JL (2000) The hydrographic character of the Knysna Estuary. Trans R Soc S Afr 55:107–122. https://doi.org/10.1080/00359190009520437
Lima MDOAC, Bergamo TF, Ward RD, Joyce CB (2023) A review of seagrass ecosystem services: providing nature-based solutions for a changing world. Hydrobiologia 850:2655–2670. https://doi.org/10.1007/s10750-023-05244-0
Loke LHL, Chisholm RA, Todd PA (2019) Effects of habitat area and spatial configuration on biodiversity in an experimental intertidal community. Ecology 100:e02757. https://doi.org/10.1002/ecy.2757
Magni P, Gravina MF (2023) Macrobenthos of lagoon ecosystems: a comparison in vegetated and bare sediments. Adv Oceanogr Limn 14:11124. https://doi.org/10.4081/aiol.2023.11124
McCloskey RM, Unsworth RKF (2015) Decreasing seagrass density negatively influences associated fauna. PeerJ 3(6):e1053. https://doi.org/10.7717/peerj.1053
McKenzie LJ (2003) Guidelines for the rapid assessment and mapping of tropical seagrass habitats. Queensland Department of Primary Industries, Cairns
Menegotto A, Dambros CS, Netto SA (2019) The scale-dependent effect of environmental filters on species turnover and nestedness in an estuarine benthic community. Ecology 100:e02721. https://doi.org/10.1002/ecy.2721
Miranda NAF, van Rooyen R, MacDonald A, Ponder WF, Perissinotto R (2014) Genetics and shell morphometrics of assimineids (Mollusca, Caenogastropoda, Truncatelloidea) in the St Lucia Estuary, South Africa. ZooKeys 419:73–86. https://doi.org/10.3897/zookeys.419.7556
Nakaoka M (2005) Plant-animal interactions in seagrass beds: ongoing and future challenges for understanding population and community dynamics. Popul Ecol 47:167–177. https://doi.org/10.1007/s10144-005-0226-z
Napier VR, Turpie JK, Clark BM (2009) Value and management of the subsistence fishery at Knysna Estuary, South Africa. Afr J Mar Sci 31:297–310. https://doi.org/10.2989/AJMS.2009.31.3.3.991
van Niekerk L, Taljaard S, Adams JB, Lamberth SJ, Weerts SP (2020b) Experimental ecosystem accounts for South African estuaries: extent, condition and ecosystem services accounts. CSIR Report CSIR/SPLA/SECO/IR/2020/0026/A
Passy SI (2016) Abundance inequality in freshwater communities has an ecological origin. Amer Nat 187:505–516. https://doi.org/10.1086/685424
Perissinotto R, Stretch DD, Whitfield AK, Adams JB, Forbes AT, Demetriades NT (2010) Ecosystem functioning of temporarily open/closed estuaries in South Africa. In: Crane JR, Solomon AE (eds) Estuaries: types, movement patterns and climatic impacts. Nova Science, New York, pp 1–69
Pillay D, Branch GM (2011) Bioengineering effects of burrowing thalassinidean shrimps on marine soft-bottom ecosystems. Oceanogr Mar Biol Ann Rev 49:137–192
Pillay D, Branch GM, Forbes AT (2007) The influence of bioturbation by the sandprawn Callianassa kraussi on feeding and survival of the bivalve Eumarcia paupercula and the gastropod Nassarius kraussianus. J Exp Mar Biol Ecol 344:1–9. https://doi.org/10.1016/j.jembe.2006.10.044
Raw JL, Riddin T, Wasserman J, Lehman TWK, Bornman TG, Adams JB (2020) Salt marsh elevation and responses to future sea-level rise in the Knysna Estuary, South Africa. Afr J Aquat Sci 45:49–64. https://doi.org/10.2989/16085914.2019.1662763
Reddering JSV, Esterhuysen K (1987) Sediment dispersal in the Knysna estuary: environmental management considerations. S Afr J Geol 90:448–457
Roux D, Freitag S, Russell I, Taplin M (2023) Knysna Estuary—Collaboratively governing and managing a shared resource. In: Whitfield AK, Breen CM, Read M (eds) Knysna Estuary—Jewel of the Garden Route. Knysna Basin Project, Knysna, pp 271–294
Russell IA, Randall RM, Kruger N (2010) Garden route national park. Knysna Coastal Area, State of Knowledge, South African National Parks, NY
Russell IA, Randall RM (2017) Effects of prolonged elevated water salinity on submerged macrophyte and waterbird communities in Swartvlei lake, South Africa. Water SA 43:666–672. https://doi.org/10.4314/wsa.v43i4.14
Schumann E (2021) Floods, sedimentation and tidal exchanges in the Keurbooms Estuary. South Africa Geomar Lett 41:34. https://doi.org/10.1007/s00367-021-00709-4
Siebert T, Branch GM (2006) Ecosystem engineers: interactions between eelgrass Zostera capensis and the sandprawn Callianassa kraussi and their indirect effects on the mudprawn Upogebia africana. J Exp Mar Biol Ecol 338:253–270. https://doi.org/10.1016/j.jembe.2006.06.024
Simon C, du Toit AN, Smith MKS, Claassens L, Smith F, Smith P (2019) Bait collecting by subsistence and recreational fishers in Knysna estuary may impact management and conservation. Afr Zool 54:91–103. https://doi.org/10.1080/15627020.2019.1608862
Simon CA, Kara J, Clarke DT, Sedick S (2022) Revisiting ‘a monograph on the Polychaeta of Southern Africa’: establishing taxonomic research priorities in southern Africa. Afr J Mar Sci 44:83–100. https://doi.org/10.2989/1814232X.2022.2041094
Skowno AL, Poole CJ, Raimondo DC, Driver A (2019) National biodiversity assessment 2018: the status of South Africa’s ecosystems and biodiversity. Synthesis Report. South African National Biodiversity Institute, Pretoria
Spratt RM, Lisiecki LE (2016) A late Pleistocene sea level stack. Clim past 12:1079–1092. https://doi.org/10.5194/cp-12-1079-2016
Tagliapietra D, Sigovini M, Ghirardini AV (2009) A review of terms and definitions to categorise estuaries, lagoons and associated environments. Mar Freshwat Res 60:497–509. https://doi.org/10.1071/MF08088
Taljaard S, van Niekerk L, Adams JB, Riddin T (2023) Advancing ecosystem accounting in estuaries. S Afr J Sci 119:14303. https://doi.org/10.17159/sajs.2023/14303
Tataranni M, Maltagliati F, Floris A, Castelli A, Lardicci C (2009) Variance estimates and taxonomic resolution: an analysis of macrobenthic spatial patterns at different scales in a western Mediterranean coastal lagoon. Mar Environ Res 67:219–229. https://doi.org/10.1016/j.marenvres.2009.02.003
Teske PR, Wooldridge T (2001) A comparison of the macrobenthic faunas of permanently open and temporarily open/closed South African estuaries. Hydrobiologia 464:227–243. https://doi.org/10.1023/A:1013995302300
Teske PR, Cherry MI, Matthee CA (2003) Population genetics of the endangered Knysna seahorse, Hippocampus capensis. Mol Ecol 12:1703–1715. https://doi.org/10.1046/j.1365-294X.2003.01852.x
Turpie J, de Wet J (2009) Recreational use and value of the Garden Route coast, South Africa. Anchor Environmental, Cape Town
Turpie JK, Adams JB, Joubert A, van Niekerk L (2002) Assessment of the conservation priority status of South African estuaries for use in management and water allocation. Water SA 28:191–206. https://doi.org/10.4314/wsa.v28i2.4885
Turpie JK, Clark B (2007) Development of a conservation plan for temperate South African estuaries on the basis of biodiversity importance, ecosystem health and economic costs and benefits. C.A.P.E. Regional Estuarine Management Programme/Anchor Environmental Consultants. Available at: https://anchorenvironmental.co.za/sites/default/files/2017-11/Cape%20Estuaries%20Cons%20Plan%20Final%20Report.pdf
Turpie JK, Clark B, Savy C (2004) Predicting invertebrate species richness on the basis of broadscale estuary characteristics. In: Adams JB (ed), Contributions to information requirements for the implementation of resource directed measures for estuaries. Volume 1. Improving the biodiversity importance rating of South African estuaries. Report to the Water Research Commission No. 1247/01/04:80-108. ISBN 1-77005-267-4
Turpie JK, Taljaard S, van Niekerk L, Adams J, Wooldridge T, Cyrus D, Clark B, Forbes N (2012a) The Estuary Health Index: a standardised metric for use in estuary management and the determination of ecological water requirements. Water Research Commission Report No. 1930/1/12. ISBN 978-1-4312-0433-4
Turpie JK, Wilson G, van Niekerk L (2012b) National biodiversity assessment 2011: national estuary biodiversity plan for South Africa. Anchor Environmental Consultants Report AEC2012/01. Cape Town
Turpie JK (2004) An updated importance rating of all South African estuaries. In: Adams JB (ed), Contributions to information requirements for the implementation of resource directed measures for estuaries. Volume 1. Improving the biodiversity importance rating of South African estuaries. Report to the Water Research Commission No. 1247/01/04:109-120. ISBN 1-77005-267-4
Unsworth RKF, Cullen-Unsworth LC, Jones BLH, Lilley RJ (2022) The planetary role of seagrass conservation. Science 377:609–613. https://doi.org/10.1126/science.abq6923
van Niekerk L, Adams JB, James NC, Lamberth SJ, MacKay CF, Turpie JK, Rajkaran A, Weerts SP, Whitfield AK (2020a) An estuary classification that encompasses biogeography and a high diversity of types in support of protection and management. Afr J Aquat Sci 45:199–216. https://doi.org/10.2989/16085914.2019.1685934
van Niekerk L, Lamberth SJ, James NC, Taljaard S, Adams JB, Theron AK, Krug M (2022) The vulnerability of South African estuaries to climate change: a review and synthesis. Diversity 14:697. https://doi.org/10.3390/d14090697
Warwick RM (1988) Analysis of community attributes of the macrobenthos of Frierfjord/Langesundfjord at the taxonomic level higher than species. Mar Ecol Progr Ser 46:167–170. https://doi.org/10.3354/meps046167
Wasserman J, Adams J, Human L (2023) The role of plants and algae in the functioning of the Knysna estuary. In: Whitfield AK, Breen CM, Read M (eds) Knysna estuary—Jewel of the Garden Route. Knysna Basin Project, Knysna, pp 115–141
Watson JEM, Dudley N, Segan DB, Hockings M (2014) The performance and potential of protected areas. Nature 515:67–73. https://doi.org/10.1038/nature13947
Western Cape Government (2018) Keurbooms-Bitou estuarine management plan. Department of Environmental Affairs and Development Planning, Cape Town
Western Cape Government (2021) Socio-Economic Profile. Knysna Municipality 2021
Wetlands International (2023) Wetlands. https://south-asia.wetlands.org/wetlands/. Accessed 23 March 2023
Whitfield AK (1989) The benthic invertebrate community of a southern Cape estuary: structure and possible food sources. Trans R Soc S Afr 47:159–179. https://doi.org/10.1080/00359198909520160
Whitfield AK (2005) Fishes and freshwater in southern African estuaries—a review. Aquat Living Resour 18:275–289. https://doi.org/10.1051/alr.2005032
Whitfield AK, Elliott M (2011) Ecosystem and biotic classifications of estuaries and coasts. In: Wolanski E, McLusky DS (eds) Treatise on estuarine and coastal science. Academic Press, Waltham, pp 99–124
Whitfield AK, Breen CM (2023). The Knysna Estuary. Jewel of the Garden Route. Knysna Basin Project, Knysna
Whitfield AK, Allanson BR, Heinecken TJE (1983) Estuaries of the cape, Part II. Synopses of Available Information on Individual Systems, Report 22: Swartvlei. CSIR, Stellenbosch
Wilke T, Haase M, Hershler R, Liu H-P, Misof B, Ponder W (2013) Pushing short DNA fragments to the limit: phylogenetic relationships of ‘hydrobioid’ gastropods (Caenogastropoda: Rissooidea). Mol Phylogenet Evol 66:715–736. https://doi.org/10.1016/j.ympev.2012.10.025
Acknowledgements
RSKB is most grateful to: Rhodes University Research Committee for support; SANParks Garden Route Scientific Services for permission to undertake fieldwork in the Wilderness and Knysna Sections of the Garden Route National Park under permit BARN-RSK/2019-034; Ruth Farre of the South African Navy Hydrographic Office for advance information on Knysna tidal data; and Janine Adams for the loan of equipment. We would also like to thank Cloverley Lawrence for her help collecting the Swartvlei data.
Funding
No external or specific funding was received for conducting this study.
Author information
Authors and Affiliations
Contributions
RSKB conceived and designed this study; data collection was performed by RSKB (all three estuaries), JLS (Keurbooms/Bitou) and CJA (Swartvlei and Keurbooms/Bitou), and data analysis by RSKB; the first draft of the manuscript was written by RSKB and all authors commented on later versions; all authors read and approved the final manuscript.
Corresponding author
Ethics declarations
Conflict of interest
The authors declare that they have no known competing financial interests or personal relationships that could have appeared to influence the work reported in this paper.
Ethical approval
All applicable international, national and/or institutional guidelines for sampling and care of organisms for the study were followed and all necessary permissions and approvals were obtained in respect of the original collections of the data.
Consent for publication
All authors consent to publication.
Additional information
Communicated by Vinicius Giglio.
Publisher's Note
Springer Nature remains neutral with regard to jurisdictional claims in published maps and institutional affiliations.
Rights and permissions
Open Access This article is licensed under a Creative Commons Attribution 4.0 International License, which permits use, sharing, adaptation, distribution and reproduction in any medium or format, as long as you give appropriate credit to the original author(s) and the source, provide a link to the Creative Commons licence, and indicate if changes were made. The images or other third party material in this article are included in the article's Creative Commons licence, unless indicated otherwise in a credit line to the material. If material is not included in the article's Creative Commons licence and your intended use is not permitted by statutory regulation or exceeds the permitted use, you will need to obtain permission directly from the copyright holder. To view a copy of this licence, visit http://creativecommons.org/licenses/by/4.0/.
About this article
Cite this article
Barnes, R.S.K., Seath, J.L. & Arendse, C.J. Differential sampling in the assessment of conservation and biodiversity merit: a comparison of the seagrass macrofauna in three nearby South African estuaries. Biodivers Conserv 33, 509–532 (2024). https://doi.org/10.1007/s10531-023-02754-0
Received:
Revised:
Accepted:
Published:
Issue Date:
DOI: https://doi.org/10.1007/s10531-023-02754-0