Abstract
Developing excellent electrochemical sensors for the determination of pollutants in the environment is crucial to advances of human detection technology. In this work, Ni/N-doped graphene oxide (Ni/N-GO) composite was constructed as an electrochemical sensor (Ni/N-GO/GCE) for the selective determination of catechol (CC) and hydroquinone (HQ). Thereinto, the Ni/N-GO composite was prepared by a high-temperature thermal reduction method. Also, a series of characterizations and electrochemical measurements were used to research the electrochemical properties of Ni/N-GO/GCE to detect CC and HQ. Furthermore, the oxidation peak potentials of CC and HQ on Ni/N-GO/GCE were 0.07 and 0.17 V, respectively, allowing selective detection of HQ and CC. Ni/N-GO/GCE exhibited linear responses toward CC and HQ in the concentration ranges of 1.4 ~ 800 and 1.0 ~ 800 μM, respectively. Besides, the detection limits were calculated as 0.06 and 0.16 μM (S/N = 3) through differential pulse voltammetry, respectively. Under optimized experimental conditions, the electrochemical sensor revealed excellent catalytic performance for the redox of CC and HQ. Finally, the results showed that Ni/N-GO/GCE was a promising electrode with superb long-term stability and reproducibility for the determination of hydroquinone isomers.








Similar content being viewed by others
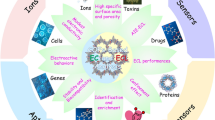
References
Lu XY, Zhang SF, Kong FY, Wang ZX, Li HY, Fang HL, Wang W (2021) Facile synthesis of TiO2-ZnO-rGO nanocomposites for highly sensitive simultaneous determination of hydroquinone and catechol. Microchem J 166:106246. https://doi.org/10.1016/j.microc.2021.106246
Huang HP, Chen YA, Chen ZZ, Chen JL, Hu YM, Zhu JJ (2021) Electrochemical sensor based on Ce-MOF/carbon nanotube composite for the simultaneous discrimination of hydroquinone and catechol. J Hazard Mater 416:125895. https://doi.org/10.1016/j.jhazmat.2021.125895
Patel BR, Noroozifar M, Kerman K (2020) Prussian blue-doped nanosized polyaniline for electrochemical detection of benzenediol isomers. Anal Bioanal Chem 412:1769–1784. https://doi.org/10.1007/s00216-020-02400-6
Choudhury SR, Houston KR, Mason D, Dingemans TJ (2021) Acid- and base-catalyzed epoxy-phenol thermosets from catechol, resorcinol and hydroquinone: A structure-property study. J Appl Polym Sci 138:e50995. https://doi.org/10.1002/app.50995
Pan ZY, Puente-Urbina A, Bodi A, van Bokhoven JA, Hemberger P (2021) Isomer-dependent catalytic pyrolysis mechanism of the lignin model compounds catechol, resorcinol and hydroquinone. Chem Sci 12:9–15. https://doi.org/10.1039/d1sc00654a
Zheng Y, Chen JB, Lu YL, Song XJ, Shi Z (2021) Direct synthesis of highly porous interconnected carbon nanosheets from sodium d-isoascorbic acid for the simultaneous determination of catechol and hydroquinone. New J Chem 45:1721–1726. https://doi.org/10.1039/d0nj04479b
Huang RM, Liao D, Chen SS, Yu JG, Jiang XY (2020) A strategy for effective electrochemical detection of hydroquinone and catechol: decoration of alkalization-intercalated Ti3C2 with MOF-derived N-doped porous carbon. Sensor Actuat B Chem 320:128386. https://doi.org/10.1016/j.snb.2020.128386
Zhou TT, Gao WL, Gao YS, Wang Q (2019) Simultaneous determination of catechol and hydroquinone using non-enzymatic Co3O4@carbon core/shell composites based sensor. J Electrochem Soc 166:B1069–B1078. https://doi.org/10.1149/2.1201902jes
Liu YY, Xie RR, Zou X, Liu JM, Wu ZY, Peng C, Zhao P (2021) A sensitive electrochemical sensor based on dual Co-doped N, P-rGO for simultaneous determination of hydroquinone and catechol. J Electrochem Soc 168:017514. https://doi.org/10.1149/1945-7111/abdc67
Zhu Y, Huai SS, Jiao JL, Xu QQ, Wu H, Zhang H (2020) Fullerene and platinum composite-based electrochemical sensor for the selective determination of catechol and hydroquinone. J Electroanal Chem 878:114726. https://doi.org/10.1016/j.jelechem.2020.114726
Garcia-Mesa JA, Mateos R (2007) Direct automatic determination of bitterness and total phenolic compounds in virgin olive oil using a pH-based flow-injection analysis system. J Agr Food Chem 55:3863–3868. https://doi.org/10.1021/jf070235v
Patil SK, Patil SA, Vadiyar MM, Awale DV, Sartape AS, Walekar LS, Kolekar GB, Ghorpade UV, Kim JH, Kolekar SS (2017) Tailor-made dicationic ionic liquid as a fluorescent sensor for detection of hydroquinone and catechol. J Mol Liq 244:39–45. https://doi.org/10.1016/j.molliq.2017.08.119
Hammani H, Laghrib F, Lahrich S, Farahi A, Bakasse M, Aboulkas A, Mhammedi MA (2019) Effect of activated carbon in distinguishing the electrochemical activity of hydroquinone and catechol at carbon paste electrode. Ionics 25:2285–2295. https://doi.org/10.1007/s11581-018-2648-6
Jeon JS, Kim BH, Lee SH, Kwon HJ, Bae HJ, Kim SK, Park JA, Shim JH, Abd El-Aty AM, Shin HC (2015) Simultaneous determination of arbutin and its decomposed product hydroquinone in whitening creams using high-performance liquid chromatography with photodiode array detection: effect of temperature and pH on decomposition. Int J Cosmetic Sci 37:567–573. https://doi.org/10.1111/ics.12228
Jurica K, Karaconji IB, Segan S, Opsenica DM, Kremer D (2015) Quantitative analysis of arbutin and hydroquinone in strawberry tree (Arbutus unedo L., Ericaceae) leaves by gas chromatography-mass spectrometry. Arh Hig Rada Toksiko 66:197–202. https://doi.org/10.1515/aiht-2015-66-2696
Chetankumar K, Swamy BEK, Naik TSSK (2020) A reliable electrochemical sensor for detection of catechol and hydroquinone at MgO/GO modified carbon paste electrode. J Mater Sci Mater El 31:19728–19740. https://doi.org/10.1007/s10854-020-04498-x
Kocak S (2020) Platinum nanoparticles/poly(isoleucine) modified glassy carbon electrode for the simultaneous determination of hydroquinone and catechol. Electroanalysis 33:375–382. https://doi.org/10.1002/elan.202060232
Gao J, He P, Yang TT, Zhou LH, Wang XJ, Chen SX, Lei H, Zhang H, Jia B, Liu JF (2019) Electrodeposited NiO/graphene oxide nanocomposite: an enhanced voltammetric sensing platform for highly sensitive detection of uric acid, dopamine and ascorbic acid. J Electroanal Chem 852:113516. https://doi.org/10.1016/j.jelechem.2019.113516
Chetankumar K, Swamy BEK, Sharma SC (2020) Fabrication of voltammetric efficient sensor for catechol, hydroquinone and resorcinol at MgO modified pre-treated carbon paste electrode. Mater Chem Phys 252:123231. https://doi.org/10.1016/j.matchemphys.2020.123231
Mashhadizadeh MH, Kalantarian SM, Azhdeh A (2020) A novel electrochemical sensor for simultaneous determination of hydroquinone, catechol, and resorcinol using a carbon paste electrode modified by Zn-MOF, nitrogen-doped graphite, and AuNPs. Electroanalysis 33:160–169. https://doi.org/10.1002/elan.202060326
Chetankumar K, Swamy BEK, Sharma SC (2020) Electrochemical preparation of poly (direct yellow 11) modified pencil graphite electrode sensor for catechol and hydroquinone in presence of resorcinol: a voltammetric study. Microchem J 156:104979. https://doi.org/10.1016/j.microc.2020.104979
Zheng XY, Hu YC, Li HZ, Han BL, Lin RY, Huang B (2020) N-doped carbon nanotube frameworks modified electrode for the selective sensing of hydroquinone and catechol. Electroanal Chem 861:113968. https://doi.org/10.1016/j.jelechem.2020.113968
Mehmandoust M, Erk N, Karaman C, Karaman O (2022) An electrochemical molecularly imprinted sensor based on CuBi2O4/rGO@MoS2 nanocomposite and its utilization for highly selective and sensitive for linagliptin assay. Chemosphere 291:132807. https://doi.org/10.1016/j.chemosphere.2021.132807
Baghayeri M, Ghanei Motlagh M, Tayebee R, Fayazi M, Narenji F (2020) Application of graphene/zinc-based metal-organic framework nanocomposite for electrochemical sensing of As(III) in water resources. Anal Chim Acta 1099:60–67. https://doi.org/10.1016/j.aca.2019.11.045
Zhang MW, Chen YP, Bosch M, Gentle T, Wang KC, Feng DW, Wang ZYU, Zhou HC (2014) Symmetry-guided synthesis of highly porous metal-organic frameworks with fluorite topology. Angew Chem Int Edit 53:815–818. https://doi.org/10.1002/anie.201307340
Ahmed A, Seth S, Purewal J, Wong Foy AG, Veenstra M, Matzger AJ, Siegel DJ (2019) Exceptional hydrogen storage achieved by screening nearly half a million metal-organic frameworks. Nat Commun 10:1568. https://doi.org/10.1038/s41467-019-09365-w
Guo H, Shen YL, Ouyang HY, Long YM, Li WF (2019) A voltammetric sensor for simultaneous determination of hydroquinone and catechol by using a heterojunction prepared from gold nanoparticle and graphitic carbon nitride. Microchim Acta 186:819. https://doi.org/10.1007/s00604-019-3798-6
Hod I, Sampson MD, Deria P, Kubiak CP, Farha OK, Hupp JT (2015) Fe-porphyrin-based metal-organic framework films as high-surface concentration, heterogeneous catalysts for electrochemical reduction of CO2. ACS Catal 5:6302–6309. https://doi.org/10.1021/acscatal.5b01767
Guo YF, Su JP, Yang H, Gu FL, Song YH, Zhu YM (2021) Flexible foam carbon/graphene oxide/Schiff base polymer-derived carbon/polyaniline for high-performance supercapacitor. Ionics 27:2639–2647. https://doi.org/10.1007/s11581-021-04032-x
Zhao NM, Song P, Wen H, Wei YH, Chen XJ, Liu Q (2022) Graphene oxide grafting naphthoquinone derivative with enhanced specific capacitance and energy density for zinc-ion hybrid supercapacitors. Ionics 28:4425–4433. https://doi.org/10.1007/s11581-022-04682-5
Shang YY, Xu L, Cai LL, Zhou B, Zhu YY, Jiang XQ (2020) Synthesis of the water-processable all-carbon composites of pristine graphene and graphene oxide through a simple one-step co-exfoliation method and application to supercapacitor. Ionics 26:5167–5177. https://doi.org/10.1007/s11581-020-03648-9
Wang DM, Liu ZH, Xu LL, Li CX, Zhao D, Ge GW, Wang ZL, Lin J (2019) A heterometallic metal-organic framework based on multi-nuclear clusters exhibiting high stability and selective gas adsorption. Dalton Trans 48:278–284. https://doi.org/10.1039/c8dt03826k
Huang RM, Chen SS, Yu JG, Jiang XY (2019) Self-assembled Ti3C2/MWCNTs nanocomposites modified glassy carbon electrode for electrochemical simultaneous detection of hydroquinone and catechol. Ecotox Environ Safe 184:109619. https://doi.org/10.1016/j.ecoenv.2019.109619
Ahmad K, Kumar P, Mobin SM (2019) Hydrothermally grown SnO2 flowers as efficient electrode modifier for simultaneous detection of catechol and hydroquinone. J Electrochem Soc 166:B1577–B1584. https://doi.org/10.1149/2.0871915jes
Wu JX, Pan ZY, Zhang Y, Wang BJ, Peng HS (2018) The recent progress of nitrogen-doped carbon nanomaterials for electrochemical batteries. J Mater Chem A 6:12932–12944. https://doi.org/10.1039/c8ta03968b
Kou Y, Yuan GH, Jin HF (2021) NiCo2S4 nanoparticles grown on graphene as the sulfur host for high-performance lithium/sulfur batteries. Ionics 28:601–607. https://doi.org/10.1007/s11581-021-04347-9
Balaji SS, Raj AGK, Karnan M, Sathish M (2020) Investigations on the nature of electrolyte on the electrochemical supercapacitor performance of heteroatom doped graphene. Ionics 26:2081–2094. https://doi.org/10.1007/s11581-020-03448-1
Fan HJ (2021) The preparation and electrochemical performance study based on nitrogen-doped cobaltosic oxide nanospheres anodes. Ionics 27:1081–1086. https://doi.org/10.1007/s11581-021-03913-5
Ji LD, Jin YS, Wu KB, Wan CD, Yang NJ, Tang Y (2018) Morphology-dependent electrochemical sensing performance of metal (Ni Co, Zn)-organic frameworks. Anal Chim Acta 1031:60–66. https://doi.org/10.1016/j.aca.2018.06.008
Wang JL, Du R, Yu CB, Xu CY, Shi ZY (2022) Application of transition metal compounds in cathode materials for lithium-sulfur battery. Ionics 28:5275–5288. https://doi.org/10.1007/s11581-022-04771-5
Danewalia SS, Khan S, Dhillon S, Bansal N, Sharma G, Singh K (2020) Effect of transition metals (MO-TiO2, MnO2, Fe2O3, and ZnO) on crystallization and electrical conductivity of SiO2-CaO-Na2O-P2O5-based glass-ceramics. Ionics 26:2959–2967. https://doi.org/10.1007/s11581-019-03311-y
Gao L, Chen GH, Zhang LL, Yan B, Yang XL (2021) Engineering pseudocapacitive MnMoO4@C microrods for high energy sodium ion hybrid capacitors. Electrochim Acta 379:138–185. https://doi.org/10.1016/j.electacta.2021.138185
Lan MQ, Xie CY, Li B, Yang SX, Xiao F, Wang S, Xiao JW (2022) Two-dimensional cobalt sulfide/iron–nitrogen–carbon holey sheets with improved durability for oxygen electrocatalysis. ACS Appl Mater Interfaces 14:11538–11546. https://doi.org/10.1021/acsami.2c00067
Yu L, Li YC, Ruan YF (2021) Fe–Mn oxides based multifunctional adsorptive/electrosensing nanoplatforms: dynamic site rearrangement for metal ion selectivity. Environ Sci Technol 55:3967–3975. https://doi.org/10.1021/acs.est.0c07733
Zhang YF, Nsabimana A, Zhu LD, Bo XJ, Han C, Li M, Guo LP (2014) Metal organic frameworks/macroporous carbon composites with enhanced stability properties and good electrocatalytic ability for ascorbic acid and hemoglobin. Talanta 129:55–62. https://doi.org/10.1016/j.talanta.2014.05.007
Guo YN, He DL, Xie AM, Qu W, Tang YN, Zhou L, Zhu RL (2019) The electrochemical oxidation of hydroquinone and catechol through a novel poly-geminal dicationic ionic liquid (PGDIL)-TiO2 composite film electrode. Polymers Basel 11:1907. https://doi.org/10.3390/polym11111907
Huang DL, Wang J, Cheng F, Ali A, Guo HS, Ying X, Si LP (2019) Synergistic effect of a cobalt fluoroporphyrin and graphene oxide on the simultaneous voltammetric determination of catechol and hydroquinone. Microchim Acta 186:553. https://doi.org/10.1007/s00604-019-3637-9
Fan JH, Pang JJ, Zhang Y, Zhang LJ, Xu WW, Wang JG (2019) Simultaneous detection of hydroquinone and catechol with decreasing pH at a bare glassy carbon electrode surface. Anal Methods-UK 11:604–609. https://doi.org/10.1039/c8ay02419g
Kocak CC, Kocak S (2020) Enhanced electrochemical determination of catechol and hydroquinone based on Pd nanoparticles/poly (Taurine) modified glassy carbon electrode. Electroanalytical 32:358–366. https://doi.org/10.1002/elan.201900500
Karabiberoglu SU, Kocak CC, Dursun Z (2019) An over-oxidized poly (Rutin) modified electrode for selective and sensitive determination of catechol and hydroquinone. J Electroanal Chem 850:113415. https://doi.org/10.1016/j.jelechem.2019.113415
Zhang Y, Xie Q, Xia Z, Gui GF, Deng F (2019) Fulvic acid reduced GO and phthalocyanine nanorods as reaction platform for simultaneous determination of catechol, hydroquinone, phenol and p-nitrophenol. J Electrochem Soc 166:B1293–B1299. https://doi.org/10.1149/2.0351914jes
Chen SS, Huang RM, Yu JG, Jiang XY (2019) Simultaneous voltammetric determination of hydroquinone and catechol by using a glassy carbon electrode modified with a ternary nanocomposite prepared from oxidized multiwalled carbon nanotubes, manganese dioxide and manganese ferrite. Microchim Acta 186:643. https://doi.org/10.1007/s00604-019-3750-9
Yang M, Guo H, Sun L, Wu N, Wang MY, Yang F, Zhang TT, Zhang JY, Pan ZL, Yang W (2021) Simultaneous electrochemical detection of hydroquinone and catechol using MWCNT-COOH/CTF-1 composite modified electrode. Colloid Surface A 625:126917. https://doi.org/10.1016/j.colsurfa.2021.126917
Song YW, Zhao MG, Wang XT, Qu HY, Liu Y, Chen SG (2019) Simultaneous electrochemical determination of catechol and hydroquinone in seawater using Co3O4/MWCNTs/GCE. Mater Chem Phys 234:217–223. https://doi.org/10.1016/j.matchemphys.2019.05.071
Rajkumar C, Thirumalraj B, Chen SM, Veerakumar P, Lin KC (2018) Voltammetric determination of catechol and hydroquinone using nitrogen-doped multiwalled carbon nanotubes modified with nickel nanoparticles. Microchim Acta 185:395. https://doi.org/10.1007/s00604-018-2926-z
Deng M, Lin SR, Bo XJ, Guo LP (2017) Simultaneous and sensitive electrochemical detection of dihydroxybenzene isomers with UiO-66 metal-organic framework/mesoporous carbon. Talanta 174:527–538. https://doi.org/10.1016/j.talanta.2017.06.061
Alshahrani LA, Liu LY, Sathishkumar P, Nan JM, Gu FL (2018) Copper oxide and carbon nano-fragments modified glassy carbon electrode as selective electrochemical sensor for simultaneous determination of catechol and hydroquinone in real-life water samples. J Electroanal Chem 815:68–75. https://doi.org/10.1016/j.jelechem.2018.03.004
Zhao L, Yu J, Yue SZ, Zhang LX, Wang ZH, Guo PR, Liu QY (2018) Nickel oxide/carbon nanotube nanocomposites prepared by atomic layer deposition for electrochemical sensing of hydroquinone and catechol. J Electroanal Chem 808:245–251. https://doi.org/10.1016/j.jelechem.2017.12.019
Shen MJ, Zhang Z, Ding YP (2016) Synthesizing NiAl-layered double hydroxide microspheres with hierarchical structure and electrochemical detection of hydroquinone and catechol. Microchem J 124:209–214. https://doi.org/10.1016/j.microc.2015.08.011
Li J, Xia JF, Zhang FF, Wang ZH, Liu QY (2018) An electrochemical sensor based on copper-based metal-organic frameworks-graphene composites for determination of dihydroxybenzene isomers in water. Talanta 181:80–86. https://doi.org/10.1016/j.talanta.2018.01.002
Xiang YH, Li L, Liu H, Shi Z, Tan YB, Wu CY, Liu YX, Wang JS, Zhang SH (2018) One-step synthesis of three-dimensional interconnected porous carbon and their modified electrode for simultaneous determination of hydroquinone and catechol. Sensor Actuat B Chem 267:302–311. https://doi.org/10.1016/j.snb.2018.04.051
Jian X, Liu X, Yang HM, Guo MM, Song XL, Dai HY, Liang ZH (2016) Graphene quantum dots modified glassy carbon electrode via electrostatic self-assembly strategy and its application. Electrochim Acta 190:455–462. https://doi.org/10.1016/j.electacta.2016.01.045
Qi YL, Cao Y, Meng XT, Cao J, Li XW, Hao QL, Lei W, Li QH, Li J, Si WM (2019) Facile synthesis of 3D sulfur/nitrogen co-doped graphene derived from graphene oxide hydrogel and the simultaneous determination of hydroquinone and catechol. Sensor Actuat B Chem 279:170–176. https://doi.org/10.1016/j.snb.2018.09.067
Acknowledgements
This work was supported by the Sichuan Provincial College Students Innovation and Entrepreneurship Training Program (Grant No. S202010619008) and the International Science and Technology Cooperation Laboratory of Micro-nanoparticle Application Research of Southwest University of Science and Technology (Grant No. 19MNA001). National Natural Science Foundation of China (41872039 and 41831285).)
Author information
Authors and Affiliations
Corresponding authors
Ethics declarations
Conflict of interest
The authors declare no competing interests.
Additional information
Publisher's note
Springer Nature remains neutral with regard to jurisdictional claims in published maps and institutional affiliations.
Supplementary Information
Below is the link to the electronic supplementary material.
Rights and permissions
Springer Nature or its licensor (e.g. a society or other partner) holds exclusive rights to this article under a publishing agreement with the author(s) or other rightsholder(s); author self-archiving of the accepted manuscript version of this article is solely governed by the terms of such publishing agreement and applicable law.
About this article
Cite this article
Liao, L., Zhou, P., Xiao, F. et al. Electrochemical sensor based on Ni/N-doped graphene oxide for the determination of hydroquinone and catechol. Ionics 29, 1605–1615 (2023). https://doi.org/10.1007/s11581-023-04892-5
Received:
Revised:
Accepted:
Published:
Issue Date:
DOI: https://doi.org/10.1007/s11581-023-04892-5