ABSTRACT
Purpose
Convection enhanced delivery (CED) is a promising method of anticancer treatment to bypass the blood–brain barrier. This paper is aimed to study drug transport under different CED operating conditions.
Methods
The convection enhanced delivery of chemotherapeutics to an intact and a remnant brain tumour after resection is investigated by means of mathematical modelling of the key physical and physiological processes of drug transport. Realistic models of brain tumour and its holding tissue are reconstructed from magnetic resonance images. Mathematical modelling is performed for the delivery of carmustine and paclitaxel with different infusion rates, solution concentrations and locations of infusion site.
Results
Modelling predications show that drug penetration can be improved by raising the infusion rate and the infusion solution concentration. The delivery of carmustine with CED is highly localised. High drug concentration only can be achieved around the infusion site. The transport of paclitaxel is more sensitive to CED-enhanced interstitial fluid as compared to carmustine, with deeper penetration into tumour interior. Infusing paclitaxel in the upstream of interstitial fluid flow leads to high spatial averaged concentration and relatively uniform distribution.
Conclusion
Results obtained in this study can be used to guide the design and optimisation of CED treatment regimens.











Similar content being viewed by others
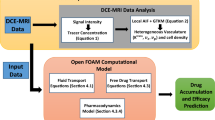
Abbreviations
- BBB:
-
Blood–brain Barrier
- CED:
-
Convection enhanced delivery
- CM:
-
Cell Membrane
- ECS:
-
Extracellular Space
- ICS:
-
Intracellular Space
- IFP:
-
Interstitial Fluid Pressure
- IFV:
-
Interstitial Fluid Velocity
- MRI:
-
Magnetic Resonance Image
REFERENCES
Zhang P, Hu L, Yin Q, Feng L, Li Y. Transferrin-modified c[RGDfK]-paclitaxel loaded hybrid micelle for sequential blood–brain barrier penetration and glioma targeting therapy. Mol Pharm. 2012;9(6):1590–8.
Mangiola A, Anile C, Pompucci A, Capone G, Rigante L, De Bonis P. Glioblastoma therapy: going beyond Hercules Columns. Expert Rev Neurother. 2010;10(4):507–14.
Alam MI, Beg S, Samad A, Baboota S, Kohli K, Ali J, et al. Strategy for effective brain drug delivery. Eur J Pharm Sci. 2010;40(5):385–403.
Lonser RR, Sarntinoranont M, Morrison PF, Oldfield EH. Convection-enhanced delivery to the central nervous system. J Neurosurg. 2015;122(3):697–706.
Bruce JN, Falavigna A, Johnson JP, Hall JS, Birch BD, Yoon JT, et al. Intracerebral clysis in a rat glioma model. Neurosurgery. 2000;46(3):683–91.
Hamstra DA, Moffat BA, Hall DE, Young JM, Desmond TJ, Carter J, et al. Intratumoral injection of BCNU in ethanol (DTI-015) results in enhanced delivery to tumor–a pharmacokinetic study. J Neuro-Oncol. 2005;73(3):225–38.
Lidar Z, Mardor Y, Jonas T, Pfeffer R, Faibel M, Nass D, et al. Convection-enhanced delivery of paclitaxel for the treatment of recurrent malignant glioma: a phase I/II clinical study. J Neurosurg. 2004;100(3):472–9.
Hassenbusch SJ, Nardone EM, Levin VA, Leeds N, Pietronigro D. Stereotactic injection of DTI-015 into recurrent malignant gliomas: phase I/II trial. Neoplasia. 2003;5(1):9–16.
Groh CM, Hubbard ME, Jones PF, Loadman PM, Periasamy N, Sleeman BD, et al. Mathematical and computational models of drug transport in tumours. J R Soc Interface. 2014;11(94):20131173.
Ulmschneider MB, Searson PC. Mathematical models of the steps involved in the systemic delivery of a chemotherapeutic to a solid tumor: From circulation to survival. J Control Release. 2015;212:78–84.
Baxter LT, Jain RK. Transport of fluid and macromolecules in tumors. I. Role of interstitial pressure and convection. Microvasc Res. 1989;37(1):77–104.
Baxter LT, Jain RK. Transport of fluid and macromolecules in tumors. II. Role of heterogeneous perfusion and lymphatics. Microvasc Res. 1990;40(2):246–63.
Baxter LT, Jain RK. Transport of fluid and macromolecules in tumors. III. Role of binding and metabolism. Microvasc Res. 1991;41(1):5–23.
Raghavan R, Brady ML, Croteau D, Friedman AH, Reardon DA, Coleman RE, et al. Clinical utility of a patient-specific algorithm for simulating intracerebral drug infusions. Neuro-Oncology. 2007;9(3):343–53.
Raghavan R, Brady M. Predictive models for pressure-driven fluid infusions into brain parenchyma. Phys Med Biol. 2011;56(19):6179.
Raghavan R, Brady ML, Sampson JH. Delivering therapy to target: improving the odds for successful drug development. Ther Deliv. 2016;7(7):457–81.
Støverud KH, Darcis M, Helmig R, Hassanizadeh SM. Modeling concentration distribution and deformation during convection-enhanced drug delivery into brain tissue. Transp Porous Media. 2012;92(1):119–43.
Linninger AA, Somayaji MR, Mekarski M, Zhang L. Prediction of convection-enhanced drug delivery to the human brain. J Theor Biol. 2008;250(1):125–38.
Zhang L, Yang M, Jiang M. Mathematical modeling for convection-enhanced drug delivery. Procedia Eng. 2012;29:268–74.
Arifin DY, Lee KYT, Wang C-H, Smith KA. Role of convective flow in carmustine delivery to a brain tumor. Pharm Res. 2009;26(10):2289–302.
Haar PJ, Broaddus WC, Z-j C, Fatouros PP, Gillies GT, Corwin FD. Quantification of convection-enhanced delivery to the ischemic brain. Physiol Meas. 2010;31(9):1075.
Zhan W, Gedroyc W, Yun XX. Mathematical modelling of drug transport and uptake in a realistic model of solid tumour. Protein Pept Lett. 2014;21(11):1146–56.
Weller RO, Djuanda E, Yow H-Y, Carare RO. Lymphatic drainage of the brain and the pathophysiology of neurological disease. Acta Neuropathol. 2009;117(1):1–14.
Fleming AB, Saltzman WM. Pharmacokinetics of the carmustine implant. Clin Pharmacokinet. 2002;41(6):403–19.
Golan DE, Tashjian AH, Armstrong EJ. Principles of pharmacology: the pathophysiologic basis of drug therapy: Lippincott Williams & Wilkins; 2011.
Fung LK, Ewend MG, Sills A, Sipos EP, Thompson R, Watts M, et al. Pharmacokinetics of interstitial delivery of carmustine, 4-hydroperoxycyclophosphamide, and paclitaxel from a biodegradable polymer implant in the monkey brain. Cancer Res. 1998;58(4):672–84.
Eikenberry S. A tumor cord model for doxorubicin delivery and dose optimization in solid tumors. Theor Biol Med Model. 2009;6:16.
Fung LK, Shin M, Tyler B, Brem H, Saltzman WM. Chemotherapeutic drugs released from polymers: distribution of 1, 3-bis (2-chloroethyl)-l-nitrosourea in the rat brain. Pharm Res. 1996;13(5):671–82.
Kalyanasundaram S, Calhoun V, Leong K. A finite element model for predicting the distribution of drugs delivered intracranially to the brain. Am J Phys Regul Integr Comp Phys. 1997;273(5):R1810–R21.
Seader J, Siirola JJ, Barnicki SD. Perry’s chemical engineer’s handbook. Perry's Chemical Engineers’ Handbook. 1997.
Kimelberg H. Water homeostasis in the brain: basic concepts. Neuroscience. 2004;129(4):851–60.
Arifin DY, Lee KYT, Wang C-H. Chemotherapeutic drug transport to brain tumor. J Control Release. 2009;137(3):203–10.
Kuh H-J, Jang SH, Wientjes MG, Au JL-S. Computational model of intracellular pharmacokinetics of paclitaxel. J Pharmacol Exp Ther. 2000;293(3):761–70.
Domb AJ, Israel ZH, Elmalak O, Teomim D, Bentolila A. Preparation and characterization of carmustine loaded polyanhydride wafers for treating brain tumors. Pharm Res. 1999;16(5):762–5.
Tiwari SB, Amiji MM. Improved oral delivery of paclitaxel following administration in nanoemulsion formulations. J Nanosci Nanotechnol. 2006;6(9–10):3215–21.
Goodman LS, Gilman A, Brunton LL, Lazo JS, Parker KL. Goodman & Gilman’s the pharmacological basis of therapeutics. 11th ed. New York: McGraw-Hill; 2005. 2021 p. p.
Allard E, Passirani C, Benoit J-P. Convection-enhanced delivery of nanocarriers for the treatment of brain tumors. Biomaterials. 2009;30(12):2302–18.
Mardor Y, Roth Y, Lidar Z, Jonas T, Pfeffer R, Maier SE, et al. Monitoring response to convection-enhanced taxol delivery in brain tumor patients using diffusion-weighted magnetic resonance imaging. Cancer Res. 2001;61(13):4971–3.
Raghavan R, Brady ML, Rodríguez-Ponce MI, Hartlep A, Pedain C, Sampson JH. Convection-enhanced delivery of therapeutics for brain disease, and its optimization. Neurosurg Focus. 2006;20(4):E12.
Layton PB, Greenberg HS, Stetson PL, Ensminger WD, Gyves JW. BCNU solubility and toxicity in the treatment of malignant astrocytomas. J Neurosurg. 1984;60(6):1134–7.
Liggins RT, Hunter W, Burt HM. Solid‐state characterization of paclitaxel. J Pharm Sci. 1997;86(12):1458–63.
Gross JF, Popel AS. Mathematical models of transport phenomena in normal and neoplastic tissue. Tumor Blood Circulation. Boca Raton: CRC Press; 1979. p. 169–83.
Jain RK. Transport of molecules in the tumor interstitium: a review. Cancer Res. 1987;47(12):3039–51.
Mitragotri S, Blankschtein D, Langer R. Ultrasound-mediated transdermal protein delivery. Science. 1995;269(5225):850.
Mitragotri S, Blankschtein D, Langer R. Transdermal drug delivery using low-frequency sonophoresis. Pharm Res. 1996;13(3):411–20.
Koike H, Tomita N, Azuma H, Taniyama Y, Yamasaki K, Kunugiza Y, et al. An efficient gene transfer method mediated by ultrasound and microbubbles into the kidney. J Gene Med. 2005;7(1):108–16.
Liu Y, Paliwal S, Bankiewicz KS, Bringas JR, Heart G, Mitragotri S, et al. Ultrasound-enhanced drug transport and distribution in the brain. AAPS PharmSciTech. 2010;11(3):1005–17.
Hynynen K. Ultrasound for drug and gene delivery to the brain. Adv Drug Deliv Rev. 2008;60(10):1209–17.
Nhan T, Burgess A, Lilge L, Hynynen K. Modeling localized delivery of Doxorubicin to the brain following focused ultrasound enhanced blood–brain barrier permeability. Phys Med Biol. 2014;59(20):5987.
Saucier-Sawyer JK, Seo Y-E, Gaudin A, Quijano E, Song E, Sawyer AJ, et al. Distribution of polymer nanoparticles by convection-enhanced delivery to brain tumors. J Control Release. 2016;232:103–12.
Sawyer AJ, Saucier-Sawyer JK, Booth CJ, Liu J, Patel T, Piepmeier JM, et al. Convection-enhanced delivery of camptothecin-loaded polymer nanoparticles for treatment of intracranial tumors. Drug Del Transl Res. 2011;1(1):34–42.
Kulkarni SA, Feng S-S. Effects of surface modification on delivery efficiency of biodegradable nanoparticles across the blood–brain barrier. Nanomedicine. 2011;6(2):377–94.
El-Kareh AW, Secomb TW. A mathematical model for comparison of bolus injection, continuous infusion, and liposomal delivery of doxorubicin to tumor cells. Neoplasia. 2000;2(4):325–38.
Sirianni RW, Zheng M-Q, Patel TR, Shafbauer T, Zhou J, Saltzman WM, et al. Radiolabeling of Poly (lactic-co-glycolic acid)(PLGA) Nanoparticles with Biotinylated F-18 Prosthetic Groups and Imaging of Their Delivery to the Brain with Positron Emission Tomography. Bioconjug Chem. 2014;25(12):2157–65.
Unterberg A, Stover J, Kress B, Kiening K. Edema and brain trauma. Neuroscience. 2004;129(4):1019–27.
Teo CS, Tan WHK, Lee T, Wang C-H. Transient interstitial fluid flow in brain tumors: Effect on drug delivery. Chem Eng Sci. 2005;60(17):4803–21.
Yu J, Guo Y, Zhao S, Xu K. Brainstem edema caused by traumatic carotid-cavernous fistula: A case report and review of the literature. Exp Ther Med. 2015;10(2):445–50.
Vogelbaum MA, Aghi MK. Convection-enhanced delivery for the treatment of glioblastoma. Neuro-Oncology. 2015;17 suppl 2:ii3–8.
Casanova F, Carney PR, Sarntinoranont M. Effect of needle insertion speed on tissue injury, stress, and backflow distribution for convection-enhanced delivery in the rat brain. PLoS ONE. 2014;9(4):e94919.
Linninger AA, Somayaji MR, Erickson T, Guo X, Penn RD. Computational methods for predicting drug transport in anisotropic and heterogeneous brain tissue. J Biomech. 2008;41(10):2176–87.
ACKNOWLEDGMENTS AND DISCLOSURES
The authors acknowledge the funding support from the National Medical Research Council (NMRC, Singapore) under the grant numbers NMRC EDG11may084, and support from the National Research Foundation, Prime Minister’s Office, Singapore under its Campus for Research Excellence and Technological Enterprise (CREATE) programme. Grant Number R-706-001-101-281, National University of Singapore. The authors thank the Supercomputing and Visualization Unit (SVU) of National University of Singapore for providing facilities to perform all the simulation works in this project, and Wei-Cheng Yan for his support in literature review.
Author information
Authors and Affiliations
Corresponding author
Rights and permissions
About this article
Cite this article
Zhan, W., Arifin, D.Y., Lee, T.K. et al. Mathematical Modelling of Convection Enhanced Delivery of Carmustine and Paclitaxel for Brain Tumour Therapy. Pharm Res 34, 860–873 (2017). https://doi.org/10.1007/s11095-017-2114-6
Received:
Accepted:
Published:
Issue Date:
DOI: https://doi.org/10.1007/s11095-017-2114-6