Abstract
Purpose
This paper presents a three-dimensional patient-specific simulation of carmustine delivery to brain tumor. The simulation investigates several crucial factors, particularly the role of convective flow, affecting drug delivery efficacy.
Methods
The simulation utilizes a complete three-dimensional tissue geometry constructed from magnetic resonance images (MRI) of a brain tumor patient in whom commercially available Gliadel® wafers were implanted for sustained delivery of carmustine following excision of the tumor. This method permits an estimation of the convective flow field (in the irregularly shaped anatomical region) which can be used for prediction of drug penetration into the domain of interest, i.e. remnant tumor. A finite volume method is utilized to perform all simulations.
Results
Drug exposure exceeds its threshold therapeutic concentration (~15 μM) but for only a limited time (i.e. less than a week) and only in the immediately adjacent tissue (i.e. less than 2 mm). A quasi-steady transport process is established within 1 day following treatment, in which the drug is eliminated rapidly by transcapillary exchange, while its penetration into the tumor is mainly by diffusion. Convection appears to be crucial in influencing the drug distribution in the tumor: the remnant tumor near the ventricle is, by one to two orders of magnitude, less exposed to the drug than is the distal remnant tumor.
Conclusions
Carmustine penetration from Gliadel® wafers implanted in brain is limited by rapid elimination via transcapillary exchange. Therefore, it could be useful to consider other therapeutic agents such as paclitaxel. In addition, local convective flow within the cavity appears to be a crucial factor in distributing the drug so that the tumor domain near the ventricle is prone to minimal drug exposure. Thus, complete removal of the tumor from this region is of particular concern.












Similar content being viewed by others
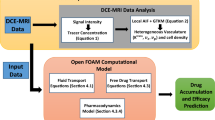
Abbreviations
- α:
-
Volume fraction of interstitial/extracellular space
- αT :
-
Volume fraction of interstitial/extracellular space in the remnant tumor
- αN :
-
Volume fraction of interstitial/extracellular space in the normal tissue
- α*:
-
Retardation constant
- α*T :
-
Retardation constant in the remnant tumor
- α*N :
-
Retardation constant in the normal tissue
- β:
-
Volume fraction of intracellular space
- ξ:
-
Dimensionless distance from cavity/remnant tumor interface (x/L d)
- ξN :
-
Dimensionless distance from the surface of polymer pellet (\( {x^{\text{N}}}/L_{\text{d}}^{\text{N}} \))
- γ:
-
Ratio of conductivity of Darcy’s permeability in the cavity and the tissue, K C/K N
- Γ:
-
Dimensionless drug concentration relative to effective concentration (C i/C i,eff)
- ΓT :
-
Dimensionless drug concentration relative to that at the cavity/remnant tumor interface (C i/C i,c)
- ΓN :
-
Dimensionless drug concentration relative to that at the surface of polymer pellet as no cavity is present (\( {C_{\text{i}}}/C_{\text{i,c}}^{\text{N}} \))
- μ:
-
Viscosity of the interstitial fluid (Pa-s)
- πi :
-
Interstitial osmotic pressure (Pa)
- πv :
-
Vascular osmotic pressure (Pa)
- ρ:
-
Density of the interstitial fluid (kg/m3)
- ψ:
-
Constant to determine the importance of convection in the remnant tumor
- ψN :
-
Constant to determine the importance of convection in the normal tissue
- ϕ:
-
Thiele modulus in the cavity
- σ:
-
Osmotic reflection coefficient of plasma
- τ:
-
Dimensionless time relative to the first-order elimination constant in the tissue
- B :
-
Average bound drug concentration (M)
- B i :
-
Bound drug concentration in the interstitial phase (M)
- B c :
-
Bound drug concentration in the intracellular phase (M)
- B m :
-
Bound drug concentration in the cell membrane phase (M)
- C :
-
Average free drug concentration (M)
- C i :
-
Free drug concentration in the interstitial phase (M)
- C c :
-
Free drug concentration in the intracellular phase (M)
- C m :
-
Free drug concentration in the cell membrane phase (M)
- C i,c :
-
Free interstitial drug concentration at the cavity/remnant tumor interface (M)
- \( C_{{\text{i}},{\text{c}}}^{\text{N}} \) :
-
Free interstitial drug concentration at the surface of polymer pellet (M)
- C i,eff :
-
Free effective interstitial drug concentration (M)
- D :
-
Lumped diffusion coefficient in the tissue (m2/s)
- D T :
-
Lumped diffusion coefficient in the remnant tumor (m2/s)
- D N :
-
Lumped diffusion coefficient in the normal tissue (m2/s)
- D i :
-
Diffusion coefficient in interstitial phase (m2/s)
- F V :
-
Rate of fluid gain from the capillary blood flow per unit volume of tissue (1/s)
- L :
-
Average thickness of the remnant tumor (m)
- L brain :
-
Average radius of the brain (m)
- L c :
-
Characteristic length scale of the cavity (m)
- L d :
-
Diffusion/reaction length scale in the tissue (m)
- \( L_{\text{d}}^{\text{T}} \) :
-
Diffusion/reaction length scale in the remnant tumor (m)
- \( L_{\text{d}}^{\text{N}} \) :
-
Diffusion/reaction length scale in the normal tissue (m)
- L p :
-
Hydraulic conductivity in the tissue (m/Pa/s)
- \( L_{\text{p}}^{\text{T}} \) :
-
Hydraulic conductivity in the remnant tumor (m/Pa/s)
- \( L_{\text{p}}^{\text{N}} \) :
-
Hydraulic conductivity in the normal tissue (m/Pa/s)
- k :
-
Lumped first-order elimination constant in the tissue (1/s)
- k T :
-
Lumped first-order elimination constant in the remnant tumor (1/s)
- k N :
-
Lumped first-order elimination constant in the normal tissue (1/s)
- k bbb :
-
Elimination constant to blood capillaries (1/s)
- k c :
-
Elimination constant in the cavity (1/s)
- k e :
-
Elimination constant due to enzymatic/non-enzymatic reactions (1/s)
- K :
-
Darcy’s permeability in the tissue (m2)
- K C :
-
Darcy’s permeability in the cavity (m2)
- K T :
-
Darcy’s permeability in the remnant tumor (m2)
- K N :
-
Darcy’s permeability in the normal tissue (m2)
- K c :
-
Binding constant between free and bound drugs in intracellular phase
- K i :
-
Binding constant between free and bound drugs in interstitial phase
- p i :
-
Interstitial fluid pressure (Pa)
- p v :
-
Vascular pressure (Pa)
- p outer :
-
Pressure at the arrachnoid villi on the outermost surface of the brain (Pa)
- p ventricle :
-
Pressure on the ventricle surface (Pa)
- P c/i :
-
Partition coefficient between cellular and interstitial phase
- P m/i :
-
Partition coefficient between membrane and interstitial phase
- Pec :
-
Peclet number in the cavity
- Pet :
-
Peclet number in the tissue, e.g. tumor
- R :
-
Average radius of the intact tumor (m)
- Re:
-
Reynolds number
- S/V :
-
Blood vessel exchange area in the tissue (m-1)
- S/V T :
-
Blood vessel exchange area in the remnant tumor (m-1)
- S/V N :
-
Blood vessel exchange area in the normal tissue (m-1)
- t :
-
Time (s)
- v :
-
Interstitial fluid velocity vector (m/s)
- v s :
-
Characteristic fluid velocity in the tissue (m/s)
- v s,c :
-
Characteristic fluid velocity in the cavity (m/s)
- v x :
-
Characteristic fluid velocity at the cavity/remnant tumor interface (m/s)
- x :
-
Distance from cavity/remnant tumor interface (m)
- x N :
-
Distance from polymer pellet surface (m)
References
Jemal A, Siegel R, Ward E, Xu J, Smigal C, et al. Cancer Statistics, 2006, CA. Cancer J Clin. 2006;56:106–30.
Berger MS, Bernstein M. Neuro-oncology: the essentials. New York: Thieme Medical; 2000.
Pardridge WM. The blood-brain barrier: bottleneck in brain drug delopment. Journal of the American Society for Experimental NeuroTherapeutics. 2005;2:3–14.
Huynh GH, Deen DF, Szoka FC Jr. Barriers to carrier mediated drug and gene delivery to brain tumors. J Control Release. 2006;110:236–59.
Heldin C-H, Rubin K, Pietras K, Ostman A. High interstitial fluid pressure—an obstacle in cancer therapy. Nat Rev Cancer. 2004;4:806–13.
Brem H, Gabikian P. Biodegradable polymer implants to treat brain tumors. J Control Release. 2001;74:63–7.
Westphal M, Ram Z, Riddle V, Hilt D, Bortey E, and On behalf of the Executive Committee of the Gliadel® Study Group. Gliadel® wafer in initial surgery for malignant glioma: long-term follow-up of a multicenter controlled trial. Acta Neurochir. 2006;148:269–75.
Giese A, Kucinski T, Knopp U, Goldbrunner R, Hamel W, Mehdorn HM, et al. Pattern of recurrence following local chemotherapy with biodegradable carmustine (BCNU) implants in patients with glioblastoma. J Neurooncol. 2004;66:351–60.
Wang CH, Li J, Teo CS, Lee T. The delivery of BCNU to brain tumors. J Control Release. 1999;61:21–41.
Tan KH, Wang FJ, Lee T, Wang CH. Delivery of etanidazole to brain tumor from PLGA wafers: a double burst release system. Biotechnol Bioeng. 2003;82:278–88.
Tan KH, Lee T, Wang CH. Simulation of intra-tumoral release of etanidazole: effects of the sized of surgical opening. J Pharm Sci. 2003;92:773–89.
Kimelberg HK. Water homeostasis in the brain: basic concepts. Neuroscience. 2004;120:852–60.
Rapoport SI. A mathematical model for vasogenic brain edema. J Theor Biol. 1978;74:439–67.
Gross JF, Popel AS. Mathematical models of transport phenomena in normal and neoplastic tissue. In: Peterson HI, editor. Tumor blood circulation. Baca Raton: CRC; 1979. p. 169–83.
Baxter LT, Jain RK. Transport of fluid and macromolecules in tumors. I. Role of interstitial pressure and convection. Microvasc Res. 1989;37:77–104.
Engelhard HH. The role of interstitial BCNU chemotherapy in the treatment of malignant glioma. Surg Neurol. 2000;53:458–64.
Nagashima T, Shirakuni T, Rapoport SI. A two-dimensional, finite element analysis of vasogenic brain edema. Neurol Med Chir (Tokyo). 1990;30:1–9.
Barzo P, Marmarou A, Fatouros P, Hayasaki K, Corwin F. Contribution of vasogenic and cellular edema to traumatic brain swelling measured by diffusion-weighted imaging. J Neurosurg. 1997;87:900–7.
Golan DE, Tashjian AH, Armstrong E, Galanter JM, Armstrong AW, Arnaout RA, et al. Principles of pharmacology: the pathophysiologic basis of drug therapy. Baltimore: Williams & Wilkins; 2008. p. 58–9.
Fleming AB, Saltzman WM. Pharmacokinetics of the carmustine implant. Clin Pharmacokinet. 2002;41:403–19.
Fung LK, Shin M, Tyler B, Brem H, Saltzman WM. Chemotherapeutic drugs released from polymers: distribution of 1, 3-bis(2-chloroethyl)-1-nitrosurea in the rat brain. Pharm Res. 1996;13:671–82.
Fung LK, Ewend MG, Silis A, Sipos EP, Thompson R, Watts M, et al. Pharmacokinetics of interstitial delivery of carmustine, 4-hydroperoxycyclophospamide, and paclitaxel from a biodegradable polymer implant in the monkey brain. Cancer Res. 1998;58:672–84.
Saltzman WM, Radomsky ML. Drugs released from polymers: diffusion and elimination in brain tissue. Chem Eng Sci. 1991;46:2429–44.
Perry RH, editor. Perry’s chemical engineers’ handbook. New York: McGraw-Hill; 1997. p. 59–71.
Kalyansundaram S, Calhoun VD, Leong KW. A finite element model for predicting the distribution of drugs delivered intracranially to the brain. Am J Physiol Regul Integr Comp Physiol. 1997;42:R1810–21.
Goodman Gilman A, Rall RW, Nies AS, Taylor P. Goodman and Gilman’s The Pharmacological Basis of Therapeutics. 8th ed. New York: Pergamon; 1990. p. 1221.
Deen WM. Analysis of Transport Phenomena. New York: Oxford University Press; 1998. p. 181–4.
Netti PA, Travascio F, Jain RK. Coupled macromolecular transport and gel mechanics: poroviscoelastic approach. AIChE Journal. 2003;49:1580–96.
Jain RK. Transport of molecules in the tumor interstitium: a review. Cancer Res. 1987;47:3039–51.
Boucher Y, Brekken C, Netti PA, Baxter LT, Jain RK. Intratumoral infusion of fluid: estimation of hydraulic conductivity and implications for the delivery of therapeutic agents. Br J Cancer. 1998;78:1442–8.
Johnson EM, Deen WM. Hydraulic permeability of agarose gel. AIChE Journal. 1996;42:1220–4.
Swabb EA, Wei J, Gullino PM. Diffusion and convection in normal and neoplastic tissues. Cancer Res. 1974;34:2814–22.
Neeves KB, Lo CT, Foley CP, Saltzman WM, Olbricht WL. Fabrication and characterization of microfluidic probes for convection enhanced delivery. J Control Release. 2006;111:252–62.
Bobo RH, Laske DW, Akbasak A, Morrison PF, Dedrick RL, Oldfield EH. Convection-enhanced delivery of macromolecules in the brain. Proc Natl Acad Sci U S A. 1994;91:2076–80.
Basser PJ. Interstitial pressure, volume, and flow during infusion into brain tissue. Microvasc Res. 1992;44:143–65.
Paulson OB, Hertz MM, Bolwig TG, Lassen NA. Filtration and diffusion of water across the blood-brain barrier in man. Microvasc Res. 1977;13:113–24.
Fraser PA, Dallas AD. Measurement of filtration coefficient cerebral microvessels of the frog. J Physiol. 1990;423:343–61.
Gerlowski LE, Jain RK. Microvascular permeability of normal and neoplastic tissues. Microvasc Res. 1986;31:288–305.
Wang PP, Frazier J, Brem H. Local delivery to the brain. Adv Drug Deliv Rev. 2002;54:987–1013.
Bickel U. How to measure drug transport across the blood-brain barrier. NeuroRX. 2005;2:15–26.
Li KW, Dang W, Tyler BM, Troiano G, Tihan T, Brem H, et al. Polifocate microshperes for paclitaxel delivery to central nervous system malignancies. Clin Cancer Res. 2003;9:3441–7.
Lee LY, Wang CH, Smith KA. Supercritical antisolvent production of biodegradable micro- and nanoparticles for controlled delivery of paclitaxel. J Control Release. 2008;125:96–106.
Ranganath SH, Wang CH. Biodegradable microfiber implants delivering paclitaxel for post-surgical chemotherapy against malignant glioma. Biomaterials. 2008;29:2996–3003.
Arifin DY, Lee LY, Wang CH. Mathematical modeling and simulation of drug release from microspheres: Implications to drug delivery systems. Adv Drug Deliv Rev. 2006;58:1274–325.
Acknowledgments
This work was supported by Biomedical Research Council (BMRC), A*STAR, Singapore and National University of Singapore under the grant number BMRC/07/1/21/19/508 (R-279-000-257-305). The authors thank the Supercomputing and Visualization Unit (SVU) of National University of Singapore for providing facilities to perform all the simulation works.
Author information
Authors and Affiliations
Corresponding author
Rights and permissions
About this article
Cite this article
Arifin, D.Y., Lee, K.Y.T., Wang, CH. et al. Role of Convective Flow in Carmustine Delivery to a Brain Tumor. Pharm Res 26, 2289–2302 (2009). https://doi.org/10.1007/s11095-009-9945-8
Received:
Accepted:
Published:
Issue Date:
DOI: https://doi.org/10.1007/s11095-009-9945-8