Abstract
Owing to the excellent redox reversibility and structural diversity, polytriphenylamine (PTPAn) has been regarded as one of the promising cathode candidates for sodium-ion batteries. However, it still suffers from the bulk aggregation and low operating capacity in practical applications. Assisted by the in-situ polymerization, leaf-like PTPAn nanosheets are uniformly introduced on the surface of carbon nanofibers (CNFs) to form the free-standing CNF@PTPAn composite electrodes. Interestingly, the formation mechanism of the leaf-on-branch structure of CNF@PTPAn composites is systematically explored, confirming that the controlling of oxidation rate and growth degree plays crucial roles in tuning the morphology and active material content of the composite electrodes. Supported by the capacity-cutting analysis, the effective coupling between the active PTPAn and conductive CNFs can provide fast electron/ion-shuttling channels and deepen the electrochemical reaction process. At 50 mA g−1, the capacity of the optimized CNF@PTPAn composite can reach 105 mA h g−1, with a stable rate capability of 78 mA h g−1 even at 400 mA g−1 after 500 cycles in a half cell. The detailed kinetic analysis confirms that the ion-storage behaviors in the low-voltage region can be tailored for the improved capacitive contribution and diffusion coefficients. Meanwhile, the flexible CNF-based full cell with CNF@PTPAn as the cathode and CNFs as the anode exhibits a high energy density of 60 W h kg−1 at 938 W kg−1. Based on this, the rational design is expected to provide more possibilities to obtain advanced freestanding electrode systems.

摘要
聚三苯胺(PTPAn)因具有优异的氧化还原可逆性和结构多样性, 被认为是极有前景的钠离子电池正极材料之一. 然而, 在实际应用中, 大块体的PTPAn材料仍存在团聚和工作容量低的问题. 本文通过原位 聚合法将树叶状的PTPAn纳米片均匀地生长在碳纳米纤维(CNFs)表 面, 构建了一种自支撑的CNF@PTPAn复合电极. 本文还系统探索了 CNF@PTPAn复合材料中枝叶结构的形成机理, 证实了原位反应的氧 化速率和生长程度对调控复合电极的形貌及其活性物质含量起了关键 作用. 通过截止容量分析发现, PTPAn活性材料与导电CNFs之间的高 效复合可以提供快速的电子/离子传输通道, 有助于增强电极的深度电 化学反应过程. 由优化后的CNF@PTPAn复合材料所组装的钠离子半 电池具有高比容量和稳定的倍率性能. 在50 mA g−1的电流密度下可达 105 mAh g−1, 甚至在400 mAg−1的高电流密度下循环500圈后仍能保持 78 mAh g−1的比容量. 动力学分析证明, 通过调控材料在低电压区域的 离子存储行为, 可以有效提升其电容主导的容量贡献和扩散系数. 同时, 以CNF@PTPAn为正极和CNFs为负极组装的柔性CNF基全电池, 在 938Wkg−1功率密度下表现出60Wh kg−1的高能量密度. 基于此, 该设 计有望为获得先进的自支撑电极体系提供更多的可能性.
Similar content being viewed by others
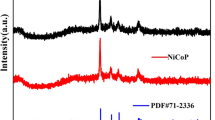
References
Palomares V, Serras P, Villaluenga I, et al. Na-ion batteries, recent advances and present challenges to become low cost energy storage systems. Energy Environ Sci, 2012, 5: 5884
Ma M, Yao Y, Wu Y, et al. Progress and prospects of transition metal sulfides for sodium storage. Adv Fiber Mater, 2020, 2: 314–337
Massé RC, Uchaker E, Cao G. Beyond Li-ion: Electrode materials for sodium- and magnesium-ion batteries. Sci China Mater, 2015, 58: 715–766
Li W-, Han C, Wang W, et al. Commercial prospects of existing cathode materials for sodium ion storage. Adv Energy Mater, 2017, 7: 1700274
Zhao C, Chen Z, Wang W, et al. In situ electropolymerization enables ultrafast long cycle life and high-voltage organic cathodes for lithium batteries. Angew Chem Int Ed, 2020, 59: 11992–11998
Xiang X, Zhang K, Chen J. Recent advances and prospects of cathode materials for sodium-ion batteries. Adv Mater, 2015, 27: 5343–5364
Cao X, Liu J, Zhu L, et al. Polymer electrode materials for high-performance lithium/sodium-ion batteries: A review. Energy Technol, 2019, 7: 1800759
Zhou G, Mo L, Zhou C, et al. Flexible naphthalene-based polyimide nanofiber cathode with hierarchical micro/nanoporous structure for high-performance organic sodium-ion batteries. Compos Commun, 2020, 22: 100490
Xiong P, Yin H, Chen Z, et al. Thiourea-based polyimide/RGO composite cathode: A comprehensive study of storage mechanism with alkali metal ions. Sci China Mater, 2020, 63: 1929–1938
Han H, Lu H, Jiang X, et al. Polyaniline hollow nanofibers prepared by controllable sacrifice-template route as high-performance cathode materials for sodium-ion batteries. Electrochim Acta, 2019, 301: 352–358
Luo W, Allen M, Raju V, et al. An organic pigment as a high-performance cathode for sodium-ion batteries. Adv Energy Mater, 2014, 4: 1400554
Yang J, Xiong P, Shi Y, et al. Rational molecular design of benzoquinone-derived cathode materials for high-performance lithium-ion batteries. Adv Funct Mater, 2020, 30: 1909597
Zhao R, Zhu L, Cao Y, et al. An aniline-nitroaniline copolymer as a high capacity cathode for Na-ion batteries. Electrochem Commun, 2012, 21: 36–38
Zhou M, Xiong Y, Cao Y, et al. Electroactive organic anion-doped polypyrrole as a low cost and renewable cathode for sodium-ion batteries. J Polym Sci B Polym Phys, 2013, 51: 114–118
Zhao Q, Whittaker AK, Zhao XS. Polymer electrode materials for sodium-ion batteries. Materials, 2018, 11: 2567
Deng W, Liang X, Wu X, et al. A low cost, all-organic Na-ion battery based on polymeric cathode and anode. Sci Rep, 2013, 3: 2671
Fan L, Liu Q, Xu Z, et al. An organic cathode for potassium dual-ion full battery. ACS Energy Lett, 2017, 2: 1614–1620
Dong X, Yu H, Ma Y, et al. All-organic rechargeable battery with reversibility supported by “water-in-salt” electrolyte. Chem Eur J, 2017, 23: 2560–2565
Feng JK, Cao YL, Ai XP, et al. Polytriphenylamine: A high power and high capacity cathode material for rechargeable lithium batteries. J Power Sources, 2008, 177: 199–204
Chen Z, Su C, Zhu X, et al. Micro-/mesoporous conjugated polymer based on star-shaped triazine-functional triphenylamine framework as the performance-improved cathode of Li-organic battery. J Polym Sci Part A-Polym Chem, 2018, 56: 2574–2583
Su C, Ye YP, Bu XD, et al. Preparation and properties of polytriphenylamine/multi-walled carbon nanotube composite as a cathode material for Li-ion batteries. Adv Mater Res, 2011, 335–336: 1512–1515
She QJ, Wei ZK, Zheng MS, et al. Electrochemical performance of polytriphenylamine as a novel non-aqueous supercapcitor cathode material. Electrochem, 2011, 17: 53–56
Yuan W, Wang B, Wu H, et al. A flexible 3D nitrogen-doped carbon foam@CNTs hybrid hosting TiO2 nanoparticles as free-standing electrode for ultra-long cycling lithium-ion batteries. J Power Sources, 2018, 379: 10–19
Sun Z, Tan K, Hou L, et al. Coordination polymer nanowires/reduced graphene oxide paper as flexible anode for sodium-ion batteries. Sci China Mater, 2020, 63: 1966–1972
Xiong G, He P, Lyu Z, et al. Bioinspired leaves-on-branchlet hybrid carbon nanostructure for supercapacitors. Nat Commun, 2018, 9: 790
Li H, Zhou Q, Liu F, et al. Biomimetic design of ultrathin edge-riched FeOOH@carbon nanotubes as high-efficiency electrocatalysts for water splitting. Appl Catal B-Environ, 2019, 255: 117755
Takahashi C, Moriya S, Fugono N, et al. Preparation and characterization of poly(4-alkyltriphenylamine) by chemical oxidative polymerization. Synth Met, 2002, 129: 123–128
Ni W, Cheng J, Li X, et al. Polymeric cathode materials of electroactive conducting poly(triphenylamine) with optimized structures for potential organic pseudo-capacitors with higher cut-off voltage and energy density. RSC Adv, 2015, 5: 9221–9227
Ali Z, Asif M, Huang X, et al. Hierarchically porous Fe2CoSe4 binarymetal selenide for extraordinary rate performance and durable anode of sodium-ion batteries. Adv Mater, 2018, 30: 1802745
Weng W, Kurihara R, Wang J, et al. Electrospun carbon nanofiber-based composites for lithium-ion batteries: Structure optimization towards high performance. Compos Commun, 2019, 15: 135–148
Hu G, Zhang X, Liu X, et al. Strategies in precursors and post treatments to strengthen carbon nanofibers. Adv Fiber Mater, 2020, 2: 46–63
Zhang L, Huang Y, Zhang Y, et al. Flexible electrospun carbon nanofiber@NiS core/sheath hybrid membranes as binder-free anodes for highly reversible lithium storage. Adv Mater Interfaces, 2016, 3: 1500467
Louarn G, Lapkowski M, Quillard S, et al. Vibrational properties of polyanilineisotope effects. J Phys Chem, 1996, 100: 6998–7006
Boyer MI, Quillard S, Rebourt E, et al. Vibrational analysis of polyaniline: A model compound approach. J Phys Chem B, 1998, 102: 7382–7392
Luo W, Schardt J, Bommier C, et al. Carbon nanofibers derived from cellulose nanofibers as a long-life anode material for rechargeable sodium-ion batteries. J Mater Chem A, 2013, 1: 10662–10666
Kvarnström C, Petr A, Damlin P, et al. Raman and FTIR spectroscopic characterization of electrochemically synthesized poly(triphenylamine), PTPA. J Solid State Electrochem, 2002, 6: 505–512
Zhang J, Yue L, Kong Q, et al. Sustainable, heat-resistant and flameretardant cellulose-based composite separator for high-performance lithium ion battery. Sci Rep, 2014, 4: 3935
Ni Q, Bai Y, Li Y, et al. 3D electronic channels wrapped large-sized Na3V2(PO4)3 as flexible electrode for sodium-ion batteries. Small, 2018, 14: 1702864
Li H, Yu X, Bai Y, et al. Effects of Mg doping on the remarkably enhanced electrochemical performance of Na3V2(PO4)3 cathode materials for sodium ion batteries. J Mater Chem A, 2015, 3: 9578–9586
Ge P, Zhang L, Zhao W, et al. Interfacial bonding of metal-sulfides with double carbon for improving reversibility of advanced alkali-ion batteries. Adv Funct Mater, 2020, 30: 1910599
Salanne M, Rotenberg B, Naoi K, et al. Efficient storage mechanisms for building better supercapacitors. Nat Energy, 2016, 1: 16070
Choi C, Ashby DS, Butts DM, et al. Achieving high energy density and high power density with pseudocapacitive materials. Nat Rev Mater, 2019, 5: 5–19
Li P, Chen C, Ding S, et al. Controllable deposition of FeV2S4 in carbon fibers for sodium-ion storage with high capacity and long lifetime. Sci China Mater, 2021, 64: 1355–1366
Mahankali K, Thangavel NK, Ding Y, et al. Interfacial behavior of water-in-salt electrolytes at porous electrodes and its effect on supercapacitor performance. Electrochim Acta, 2019, 326: 134989
Taberna PL, Simon P, Fauvarque JF. Electrochemical characteristics and impedance spectroscopy studies of carbon-carbon supercapacitors. J Electrochem Soc, 2003, 150: A292
Ates M, Kuzgun O, Yildirim M, et al. rGO/MnO2/polyterthiophene ternary composite: Pore size control, electrochemical supercapacitor behavior and equivalent circuit model analysis. J Polym Res, 2020, 27: 202
Zhu X, Ni Z, Dong L, et al. In-situ modulation of interactions between polyaniline and graphene oxide films to develop waterborne epoxy anticorrosion coatings. Prog Org Coatings, 2019, 133: 106–116
Luo SW, Gu R, Shi P, et al. π-π interaction boosts catalytic oxygen evolution by self-supporting metal-organic frameworks. J Power Sources, 2020, 448: 227406
Fang Y, Xiao L, Qian J, et al. 3D graphene decorated NaTi2(PO4)3 microspheres as a superior high-rate and ultracycle-stable anode material for sodium ion batteries. Adv Energy Mater, 2016, 6: 1502197
Wang X, Kajiyama S, Iinuma H, et al. Pseudocapacitance of MXene nanosheets for high-power sodium-ion hybrid capacitors. Nat Commun, 2015, 6: 6544
Le Z, Liu F, Nie P, et al. Pseudocapacitive sodium storage in mesoporous single-crystal-like TiO2-graphene nanocomposite enables highperformance sodium-ion capacitors. ACS Nano, 2017, 11: 2952–2960
Hou Z, Li X, Liang J, et al. An aqueous rechargeable sodium ion battery based on a NaMnO2-NaTi2(PO4)3 hybrid system for stationary energy storage. J Mater Chem A, 2015, 3: 1400–1404
Acknowledgements
This work was financially supported by the National Natural Science Foundation of China (22075042), the Natural Science Foundation of Shanghai (20ZR1401400 and 18ZR1401600), the Shanghai Scientific and Technological Innovation Project (18JC1410600), the Fundamental Research Funds for the Central Universities and DHU Distinguished Young Professor Program (LZB2021002).
Author information
Authors and Affiliations
Contributions
Mo L, Zhou G and Miao Y conceived the idea. Mo L performed the experiments, analyzed the data and wrote the manuscript. Zhou G performed partial experiments and tests. Ge P contributed to the schematic diagrams partly and guided the manuscript. Miao Y contributed to the theoretical analysis, supervision and editing. Liu T contributed to the supervision and editing. All authors contributed to the general discussion.
Corresponding authors
Additional information
Lulu Mo received her Master’s degree in Chemistry from Henan University of Technology in 2017. Currently, she is a PhD candidate under the supervision of Prof. Tianxi Liu at the College of Materials Science and Engineering, Donghua University. Her research focuses on the design and synthesis of novel carbon nanofiber composites for lithium/sodium ion batteries.
Peng Ge is an associate professor at the School of Minerals Processing and Bioengineering, Central South University. He received his PhD at the Central South University in 2019. His research interests are the development of new materials derived from mineral chemistry and their applications in electrochemical energy storage and conversion.
Yue-E Miao received her BS degree from the Southeast University in 2010 and PhD degree from Fudan University in 2015. She is now an associate professor at the College of Materials Science and Engineering, Donghua University. Her research interests mainly focus on high-performance organic fiber electrodes/separators, carbon nanofiber composites, as well as their applications in electrochemical energy storage devices (such as Li/Na-ion batteries and Li-S batteries).
Tianxi Liu obtained his BS degree from Henan University (1992) and PhD degree from Changchun Institute of Applied Chemistry, Chinese Academy of Sciences (1998). He is currently a full professor at the College of Materials Science and Engineering, Donghua University. His main research interests include polymer nanocomposites, organic/inorganic hybrid materials, nanofibers and their composites, and advanced energy materials for energy conversion and storage.
Conflict of interest
The authors declare that they have no conflict of interest.
Supporting Information
40843_2021_1718_MOESM1_ESM.pdf
Flexible polytriphenylamine-based cathodes with reinforced energy-storage capacity for high-performance sodium-ion batteries
Rights and permissions
About this article
Cite this article
Mo, L., Zhou, G., Ge, P. et al. Flexible polytriphenylamine-based cathodes with reinforced energy-storage capacity for high-performance sodium-ion batteries. Sci. China Mater. 65, 32–42 (2022). https://doi.org/10.1007/s40843-021-1718-2
Received:
Accepted:
Published:
Issue Date:
DOI: https://doi.org/10.1007/s40843-021-1718-2