Abstract
The kinetics of the carbothermic reduction of iron oxides in a composite pellet made of taconite concentrate and high-volatility coal has been studied by means of mathematical modeling that simultaneously takes into account the transfer rates of both the mass and the heat, and the rates of chemical reactions. The computational results, which have been validated with experimental data in the literature, confirm that the overall rate of the carbothermic reduction, which is strongly endothermic, is limited by heat-transfer steps. From a kinetics viewpoint, the optimum composition of the composite pellet is approximately in accordance with the stoichiometry, when CO is assumed to be the sole oxide of carbon in the gas. To raise the temperature of the pellet from its ambient value to furnace temperature, the heat required is greater than that needed for sustaining all chemical reactions, including the Boudouard reaction. The gaseous product consists mainly of CO and H2, except in the very initial stage. The overall observable reaction rate, in terms of the volumetric rate of the generation of gases, peaks at approximately 30 seconds of reaction time.














Similar content being viewed by others
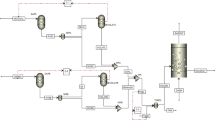
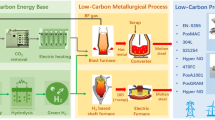
Abbreviations
- A :
-
total area of the reaction front per unit volume of pellet (m2 · m−3)
- a i , b i :
-
stoichiometric coefficient of the solid and gaseous reactant in reaction i
- C p :
-
heat capacity (J · mol−1 · K−1)
- c :
-
concentration of gaseous species (mol · m−3)
- d p :
-
pellet diameter (m)
- E a :
-
apparent activation energy (J · mol−1)
- f m,j :
-
volumetric fraction of component j
- f i−a (c g ):
-
\( f_{i - a} (c_g ) = \left( {c_{{\text{CO}}} - c_{{\text{CO}}_2 } /K_{E,i - a} } \right)/b_{i - a} \) (i = R1, R2, and R3)
- f i−b (c g ):
-
\( f_{i - b} (c_g ) = \left( {c_{{\text{H}}_2 } - c_{{\text{H}}_{\text{2}} {\text{O}}} /K_{E,i - b} } \right)/b_{i - b} \) (i = R1, R2, and R3)
- f i−a (c g ):
-
\( f_{i - a} (c_g ) = \left( {c_{{\text{CO}}_{\text{2}} } - c_{{\text{CO}}}^2 /K_{E,i - a} } \right)/b_{i - a} \) (i = R4)
- f i−b (c g ):
-
\( f_{i - b} (c_g ) = \left( {c_{{\text{H}}_{\text{2}} {\text{O}}} - c_{{\text{CO}}} c_{{\text{H}}_2 } /K_{E,i - b} } \right)/b_{i - b} \) (i = R4)
- ΔH°:
-
reaction heat (J)
- K E,i :
-
equilibrium constant for reaction i
- k red, k gas :
-
apparent reaction rate constants of the reduction of iron oxides and the gasification of carbon, \( k_{i - \nu } = k_{o,i - \nu } \exp {\text{ }}( - \frac{{E_{a,i - \nu } }} {{{\text{R}}T}}) \) (m · s−1)
- k dev :
-
apparent reaction rate constant of the devolatilization of coal, \( k_{{\text{dev}},j} = k_{{\text{devo}},j} \exp {\text{ }}( - \frac{{E_{{\text{deva}},j} }} {{{\text{R}}T}}) \) (s−1)
- M :
-
molecular weight (kg · mol−1)
- \( {\mathop N\limits^ \bullet}_{i\nu } \) :
-
local reaction rate of reaction (i − ν) (mol · s−1 · m−3)
- \( {\mathop N\limits^ \bullet}_{{\text{dev}},j} \) :
-
devolatilization rate of the volatile matter j in the coal (mol · s−1 · m−3)
- Δn :
-
generation rate of local extra gas (mol · s−1)
- R:
-
gas constant, 8.314 (J · mol−1 · K−1)
- R p :
-
pellet radius (m)
- r i :
-
position of the reaction front in each reacting grain (m)
- S f,j :
-
shape factor of grain j
- T :
-
temperature (K)
- T f :
-
temperature inside furnace chamber (K)
- T s :
-
temperature at pellet surface (K)
- t :
-
time (s)
- V :
-
pellet volume (m3)
- W :
-
weight (kg)
- y :
-
molar fraction
- ε :
-
emissivity of pellet surface
- κ j :
-
unit conversion factor, κ j = 1/M j (mol · kg−1)
- λ eff :
-
effective thermal conductivity (W · m−1 · K−1)
- λ g :
-
thermal conductivity of gas, \( \lambda _g = \frac{{\sum\limits_j {y_j \lambda _{g,j} M_j^{1/3} } }} {{\sum\limits_j {y_j M_j^{1/3} } }} \) (W · m−1 · K−1)
- λ s :
-
thermal conductivity of solid, \( \lambda _s = \prod\limits_j {\lambda _{m,j}^{f_{m,j} } } \) (W · m−1 · K−1)
- ξ :
-
dimensionless radius of the pellet, ξ = R p /R o
- ρ m :
-
apparent density of pellet (kg · m−3)
- ρ s,i :
-
density of the grain concerned with reaction i (kg · m−3)
- σ :
-
Stefan–Boltzmann constant, 5.6651 × 10−8 (W · m−2 · K−4)
- ϕ :
-
porosity
- Ψ j :
-
mass fraction of component j in the volatile matter contained in the coal
- ω :
-
mass fraction of all volatile matter in the coal
References
Direct Reduction of Iron Ore: A Bibliographical Survey, The Metals Society, London, 1979, pp. 86–633
R.L. Stephenson: Direct Reduced Iron: Technology and Economics of Production and Use, The Iron and Steel Society of AIME, Warrendale, PA, 1980, pp. 64–95
W.-K. Lu: Metall. Mater. Trans. B, 2001, vol. 32B, pp. 757–62
S. Sun: Doctoral Thesis, McMaster University, Hamilton, ON, Canada, 1997
W.-K. Lu, and D. Huang: Miner. Process. Extr. Metall. Rev., 2003, vol. 24, pp. 293–324
O.M. Fortini, and R.J. Fruehan: Metall. Mater. Trans. B, 2005, vol. 36B, pp. 709–17
S. Prakash: J. S. Afr. Inst. Min. Metall., 1996, Jan.–Feb., pp. 3–16
E. Donskoi, and D.L.S. McElwain: Ironmaking and Steelmaking, 2001, vol. 28 (5), pp. 384–89
S. Meissner, I. Kobayashi, Y. Tanigaki, and K.-H. Tacke: Ironmaking and Steelmaking, 2003, vol. 30 (2), pp. 170–76
P. C. Pistorius: Scand. J. Metall., 2005, vol. 34, pp. 122–30
T. Coetsee, P.C. Pistorius, and E.E. de Villiers: Miner. Eng., 2002, vol. 15, pp. 919–29
F.S. Manning and W.O. Philbrook: in Blast Furnace––Theory and Practice, J.H. Strassburger, ed., Gordon and Breach Science Publishers, New York, 1969, pp. 839–78.
E.E. Seaton, J.S. Foster, and J. Velasco: Trans. ISIJ, 1983, vol. 23, pp. 497–503
S. Sun, and W.-K. Lu: ISIJ Int., 1999, vol. 39 (2), pp. 130–38
P.G. Collishaw, and J.R.G. Evens: J. Mater. Sci., 1994, vol. 29, pp. 2261–73
D.M. Dawson, and A. Briggs: J. Mater. Sci., 1981, vol. 16, pp. 3346–56
E.E. Gonzo: Chem. Eng. J., 2002, vol. 90, pp. 299–302
E. Donskoi, and D.L.S. McElwain: Metall. Mater. Trans. B, 2003, vol. 34B, pp. 93–102
T. Akiyama, H. Ohta, R. Takahashi, Y. Waseda, and J. Yagi: ISIJ Int., 1992, vol. 32 (7), pp. 829–37
B. Atkinson, and D. Merrick: Fuel, 1983, vol. 62, pp. 553–61
S.K. Dutta, and A. Ghosh: Metall. Mater. Trans. B, 1994, vol. 25B, pp. 15–26
E. Donskoi, D.L.S. McElwain, and L.J. Wibberley: Metall. Mater. Trans. B, 2003, vol. 34B, pp. 255–66
Q.T. Tsay, W.H. Ray, and J. Szekely: AIChE J., 1976, vol. 22 (6), pp. 1064–72
I. Barin and O. Knacke: Thermochemical Properties of Inorganic Substances, Springer-Verlag, Berlin, 1973, pp. 116, 162, 163, 275, 293, 294, 316, and 323
J. Zhang, T. Yang, Z. Gao, B. Gao, Z. Zhang, and L. Wang: J. Univ. Sci. Technol., Beijing, 2001, vol. 23 (4), pp. 308–10
S.V. Patankar: Numerical Heat Transfer and Fluid Flow, McGraw-Hill, New York, 1980, p. 56
Acknowledgments
The authors express their gratitude for the financial support for this project provided by the Natural Science and Engineering Research Council of Canada, in the form a Discovery Grant, and American Iron and Steel Institute, Washington, D. C., in the form of a contract.
Author information
Authors and Affiliations
Corresponding author
Additional information
Manuscript submitted October 30, 2006.
Appendix
Appendix
Rights and permissions
About this article
Cite this article
Sun, K., Lu, WK. Mathematical Modeling of the Kinetics of Carbothermic Reduction of Iron Oxides in Ore-Coal Composite Pellets. Metall Mater Trans B 40, 91–103 (2009). https://doi.org/10.1007/s11663-008-9199-6
Published:
Issue Date:
DOI: https://doi.org/10.1007/s11663-008-9199-6