Abstract
Melatonin is known to improve the tolerance of plants to adverse abiotic factors (AAF), including dehydration. Nevertheless, details of the action of this phytohormone are not sufficiently comprehended. In the current study, the effects of melatonin on the growth, water status, and content of malonic dialdehyde were evaluated in etiolated seedlings of narrow-leaved lupine (Lupinus angustifolius L.) subjected to dehydration. The respiration of mitochondria isolated from different organs of these plants was also analyzed. Here, the 4-day-old seedlings have been deprived of water for 1 day. This entailed the increased water deficit up to 22% in the roots and 6% in the hypocotyls. Such desiccation of the seedlings retarded the growth of their hypocotyls but increased that of the roots by 13% in comparison with the undesiccated control. Both root and hypocotyl tissues accumulated MDA under these conditions. The dehydration of the seedlings negatively affected the respiration of the mitochondria isolated from these organs. The rate of oxidation of the substrates, especially malate, was diminished at the metabolic State 3 mainly due to an inhibition of the cytochrome respiration pathway (CP). For example, upon oxidation of malate, the activity of CP decreased 1.8 times in the mitochondria from hypocotyls and four to five times in those from roots. Pretreatment of the seedlings with 0.1 µM melatonin prevented the dehydration-induced rise in the MDA level in the hypocotyls but, by contrast, stimulated this parameter by 27% in the roots. Under drought conditions combined with exogenous melatonin, the growth of hypocotyls was close to that in the undesiccated control, while the root growth was lower than that in the control. In the seedlings normally supplied with water, treatment with melatonin did not significantly influence the respiration of the mitochondria prepared from the hypocotyls or roots. However, under water shortage, melatonin completely blocked the inhibition of a substrate oxidation in the hypocotyl mitochondria, chiefly because of sustaining the CP activity. As a result, exogenous melatonin increased the rate of malate oxidation by 87% and succinate oxidation by 26% in metabolic state 3 in the mitochondria from the hypocotyls. Application of this hormone to the plants only partially reversed the dehydration-induced inhibition of the oxidative phosphorilation in the root mitochondria. In this case, the oxidation of respiratory substrates, especially succinate, and activity of CP were still inhibited. Possible causes of the differences between the hypocotyl and root cells of the lupine seedling in their responses to melatonin and water deficit, namely, an extent of oxidative stress and functions of mitochondria, are discussed.
Similar content being viewed by others
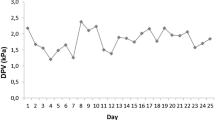
Avoid common mistakes on your manuscript.
INTRODUCTION
Melatonin has been broadly investigated for several years as a molecule with a wide action spectrum that influences metabolism of both plant and animal organisms [1–3]. It is primarily considered as an antioxidant restricting accumulation of surplus ROS exerted by adverse abiotic factors (AAF) [3]. Rather recently, after the discovery of melatonin receptors, this compound has been attributed to regulatory molecules as a novel phytohormone [4, 5]. Meanwhile, a negative prooxidative action of melatonin is also described, mainly in experiments with isolated mitochondria [2, 6]. A similar action was found in products of melatonin conversion in tobacco leaves [7]. Agathokleous et al. [2] concluded that melatonin is involved in the antistress protection in plants and animals through a mechanism of hormesis, which is peculiar to pharmacological agents and obeys biphasic dose-effect dependence. Hernandes et al. [8] reported that dose-effect dependencies of the melatonin’s effects on seeds and plants of Arabidopsis thaliana are different.
The role of melatonin in maintenance of metabolism under unfavorable conditions has mainly been studied for extreme temperatures, dehydration, salinization, and heavy metal intoxication. Water loss by plants is a common event since it accompanies many abiotic impacts, for instance, too high or low temperatures and soil salinization or dehydration. Water stress disturbs a balance between generation and inactivation of ROS and, hence, leads to oxidative stress [9, 10]. The mitochondrial respiratory system contributes to these processes, because it produces ROS, and this activity is highly enhanced under drought conditions due to an impaired function of ETC [10, 11]. To cope with oxidative stress, the mitochondria activate oxidoreductases, such as alternative CN-resistant oxidase and rotenone-insensitive NADPH dehydrogenase [12].
It seems feasible that treatment of plants or seeds with melatonin would significantly elevate plant tolerance to dehydration and, therefore, prevent large losses of agricultural crops [13]. In the investigations of plant desiccation, the main attention has been paid to the role of melatonin in deactivation of ROS, stimulation of plant antioxidant defense, and activation of the photosynthetic system [13]. Meanwhile, it should be noted that respiratory metabolism, in addition to photosynthesis, is an essential energy-supplying process in the plants, and its role determines survival of the plants impacted by AAF. Under strong water deficit, photosynthesis may fall down to zero, but respiration always remains at a minimal necessary level [14] or increases in some cases [15]. Restoration of photosynthesis after water stress requires an intense respiratory metabolism [16]. In etiolated plant objects, photosynthesis is absent, and respiration plays the dominant role in maintenance of energy supply of the cell. In fact, melatonin’s interactions with components of the respiratory system have been principally investigated on animal mitochondria before [17, 18]. Effects of melatonin on functions of the complexes of the mitochondrial respiratory chain and the antioxidant system were documented. As far as we know, only one publication reports the influence of melatonin on functions of the components of the mitochondrial respiratory system in the plants subjected to AAF [1]. It is shown that both chilling and melatonin stimulate the rate of succinate oxidation, the activity of ATP synthase, and the yield of ATP. Besides, melatonin accelerates plant growth. Taking a lack of such studies into account, the goal of the present work was to elucidate an effect of melatonin on the growth of seedlings, level of oxidative stress, and respiration of the mitochondria isolated from hypocotyls and roots of lupine seedlings deprived of water.
MATERIALS AND METHODS
Plant material. Etiolated 5-day-old seedlings of narrow-leaved lupine (Lupinus angustifolius L., cv. Ladnyi) were examined. The seeds were purchased from Nemchinovka Federal Research Center (https://www.ficnemchinovka.ru/). The 4-day-old seedlings grown on 0.5 Hoagland nutrient solution were treated with melatonin. For this purpose, the seedlings were incubated for 1 h in the same solution, which was air-bubbled and amended with 0.1 µM melatonin. Afterwards, the seedlings were placed onto dry filter paper for 1 day. The 5-day-old, nondesiccated seedlings were taken as controls. This melatonin concentration was previously tested and chosen as effective for the mitochondrial ETC. The lengths of the seedlings were measured by means of a ruler with a vernier.
Mitochondria were isolated from the hypocotyls or roots of lupine seedlings, the control or desiccated, the treated or not with melatonin. The slightly modified uniform technique of differential centrifugation was used [19]. All steps of the procedure were performed at 4°C using a high-speed Hitachi CR22G-III centrifuge (Japan). Plant material was disrupted in a homogenization medium (1 : 4, w/v, g/mL). The medium consisted of 0.4 M sucrose, 10 mM EDTA, 3 mM DTT, and 0.1% of FA-free BSA in 20 mM HEPES buffer, pH 8.0. The homogenate was filtered through four layers of Miracloth followed by 5-min centrifugation at 15000g. The sediment was resuspended in the washing medium (0.4 M sucrose, 10 mM EDTA, and 0.2% of FA-free BSA in 20 mM HEPES, pH 7.4) and was centrifuged at 4000g for 5 min. The resultant supernatant was centrifuged at 12 000g for 10 min. The sediment of mitochondria, containing approximately 5 mg/mL protein, was resuspended in 1 mL medium, containing 0.3 M sucrose, and 0.1% FA-free BSA in 20 mM HEPES, pH 7.2, to be stored at 0°C.
Oxygen uptake by mitochondria was measured polarographically using a Clark-type oxygen electrode. A standard incubation medium (1 mL) contained 0.3 M sucrose, 5 mM MgCl2, 5 mM KH2PO4 (рН 7.4), and 0.1% BSA in 20 mM HEPES buffer, pH 7.4. Alternatively, where is specified in figures, respiratory substrates were added, namely, 10 mM malate in the presence of 10 mM glutamate or 10 mM succinate. Upon oxidation of succinate or malate in the metabolic state 3, respectively, 100 or 200 µM ADP were added. The content of mitochondrial protein was assayed according to Bradford with BSA as a standard.
Activities of the pathways of mitochondrial oxidation were evaluated with the help of inhibitory analysis [20]. The activity of the cytochrome respiration pathway (CP) was derived from the degree of sensitivity of mitochondrial respiration in state 3 to 2 mM KCN. The maximal activity of the alternative cyanide-resistant pathway (AP) was assessed by the degree of respiration sensitivity to 2 mM salicylhydroxamic acid in the presence of cyanide. The optimal concentrations of the inhibitors were chosen by titration in the preliminary tests.
Water deficit of the tissues (WD) was estimated by the following formula [21]:
Malonic dialdehyde (MDA) was assayed in the tissues by the method in [21]. Tissue material (1 g) was disrupted in a water-ethanol solution (20 : 80, v/v) followed by centrifugation of the homogenate at 3000g for 10 min. Later on, the supernatant (1 mL) was added to test-tubes containing 20% TCA (– TBA variant) or 20% TCA + 65% TBA (+ TBA variant). The samples were heated in a water bath at 95°C for 30 min followed by rapid cooling and 10-min centrifugation at 3000g. The intensity of the diffusate coloration was read at 440, 532, and 600 nm with a Genesis 10UV spectrophotometer. MDA content (nmol/mL) was evaluated by the following formulas:
Statistics. In the table and figures, means and their standard deviations are reported. The experiments were performed with 3 to 5 biological replications. The statistical significance was estimated with Tukey’s test in the ANOVA program. The means significantly different at P ≤ 0.05 are indicated with different letters.
RESULTS
Effect of Seedlings’ Desiccation and Treatment with Melatonin on Their Growth
The index of water deficit was limited within a regular range of 4–10% in the hypocotyls and roots of the control 5-day-old plants. These organs actively grew: the length of the hypocotyls augmented by 52% and that of the roots by 29.9% per day (Table 1). Under dehydration, the water deficit increased from 3.9 to 5.9% in the hypocotyls and from 8 to 22% in the roots. Treatment with melatonin did not affect the water deficit in the nondesiccated control but reduced the water loss in the seedlings subjected to dehydration: down to 4.9% in the hypocotyls and to 8.3% in the roots (Fig. 1).
Effect of desiccation and treatment with melatonin on water deficit of (1) hypocotyls and (2) roots of 5-day-old lupine seedlings. Experimental variants: I—control seedlings; II—melatonin-treated seedlings; III—desiccated seedlings; IV—melatonin-treated desiccated seedlings. Means and their standard deviations are reported. The statistical significance was estimated with Tukey’s test in the ANOVA program. The means significantly different at P ≤ 0.05 are indicated with different letters.
One-day desiccation slowed down the daily growth rate of the hypocotyls to 42.8% but stimulated that of the roots up to 46.5%. Pretreatment of the water-deprived seedlings with melatonin led to the hypocotyl and root daily elongation by 51.8 and 41.5%, respectively (Table 1). Therefore, the application of melatonin to the seedlings exposed to drought accelerated the growth of hypocotyls but retarded the root growth.
Effect of Seedlings’ Desiccation on Respiration of Mitochondria Isolated from Their Hypocotyls and Roots
Mitochondria isolated from the hypocotyls of the control seedlings displayed a high rate of malate and succinate oxidation in state 3 (in the presence of ADP), which mainly proceeded through the major CP of electron transport. They also manifested tight coupling of oxidation and phosphorilation. That was evidenced by a high value of respiratory control (RC), which is derived from the ratio of a substrate oxidative rate in state 3 (in the presence of ADP) to that in state 4 (after exhausting of ADP utilized in the ATP synthesis) (Fig. 2).
Effect of desiccation and treatment with melatonin on respiration and activities of different pathways of oxidation of (1) malate and (2) succinate by mitochonria isolated from hypocotyls of 5-day-old seedlings. (a) Rate of substrate oxidation in state 3, (b) rate of substrate oxidation through the cytochrome pathway, (c) rate of substrate oxidation through the alternative pathway, (d) respiratory control. Experimental variants: I—control seedlings; II—melatonin-treated seedlings; III—desiccated seedlings; IV—melatonin-treated desiccated seedlings. Means and their standard deviations are reported. The statistical significance of the difference between control and treated samples was estimated with Tukey’s test in the ANOVA program. The means corresponding to each substrate (1 or 2) that are significantly different at P ≤ 0.05 are indicated with different letters.
In the mitochondria prepared from the hypocotyls of the seedlings subjected to desiccation, the oxidation rate of an NAD-dependent substrate (malate) in state 3 decreased by 40% in comparison with the undesiccated control due to the decrease in activity of CP. The value of RC was by almost 40% lower than the control. The maximal activity of AP significantly (by 56%) increased in this case. The succinate oxidation involving the Complex II of ETC was found to be less sensitive to water deficit (Fig. 2).
The mitochondria from the roots of the control plants, in comparison with those from the hypocotyls, exhibited a lower rate of oxidation of malate and succinate in state 3 (by 16 and 25%, respectively). The activity of CP was also decreased: by 45 and 31%. The RC value was the most strongly suppressed (by 68 and 41%) upon oxidation of malate and succinate. Presumably, this demonstrates a partial uncoupling of the oxidative phosphorilation in the root mitochondria (Fig. 3).
Effect of desiccation and treatment with melatonin on respiration and activities of different pathways of oxidation of (1) malate and (2) succinate by mitochonria isolated from of roots of 5-day-old seedlings. (a) Rate of substrate oxidation in state 3, (b) rate of substrate oxidation through the cytochrome pathway, (c) rate of substrate oxidation through the alternative pathway, (d) respiratory control. Experimental variants: I—control seedlings; II—melatonin-treated seedlings; III—desiccated seedlings; IV—melatonin-treated desiccated seedlings. Means and their standard deviations are reported. The statistical significance of difference between control and treated samples was estimated with Tukey’s test in the ANOVA program. The means corresponding to each substrate (1 or 2) that are significantly different at P ≤ 0.05 are indicated with different letters.
It was also found that the respiration of the root mitochondria was more vulnerable to water deficiency. In particular, the rate of oxidation of the respiratory substrates in state 3 sharply fell, namely, by 80 and 70% for malate and succinate, respectively. Apparently, this was a consequence of inhibition of the CP activity, which decreased by 86% upon malate oxidation and by 76% for succinate. In this case, the value of RC also decreased in the oxidation of malate and succinate, respectively, by 29 and 26%. This effect, however, was not related to the alternative pathway of electron transport, because the AP activity was also suppressed under shortage of water (Fig. 3).
Effect of Treatment with Melatonin and Desiccation of Seedlings on Respiration of Mitochondria Isolated from Their Hypocotyls and Roots
Treatment of the control undesiccated seedlings with melatonin did not significantly change the respiration of the mitochondria from their hypocotyls and roots. Meanwhile, the rate of oxidation of the NAD-dependent substrate in state 3 by the mitochondria from hypocotyls was significantly reduced by 28% (Fig. 2). If the mitochondria have been isolated from the desiccated hypocotyls, melatonin, on the contrary, stimulated the respiration. In this case, the rate of oxidation of succinate and malate increased, respectively, by 25 and 15% in state 3. The activity of CP remained close to the control level upon the oxidation of malate and was by almost 35% higher than the control upon the succinate oxidation. Besides, the value of RC increased by 33% during the malate oxidation (Fig. 2).
In the mitochondria isolated from the roots of the dehydrated seedlings, exogenous melatonin only partially reversed the inhibitory action of this unfavorable factor on the respiration. Here, the rate of malate oxidation increased 1.5 times and that of succinate oxidation by 40% above the levels of the hormone-untreated plants. Nevertheless, the uncoupling of respiration and phosphorilation, together with the suppressed rates of substrate oxidation in state 3, witness to the fall of phosphorilating capacity of the organelles (Fig. 3).
Effect of Seedlings’ Desiccation and Treatment with Melatonin on MDA Content in Their Hypocotyls and Roots
Dehydration of seedlings gave rise to conditions of oxidative stress and deranged structural integrity of their membranes. This was evidenced by the enhanced, by 57.2%, concentration of MDA in the hypocotyls of 5-day-old seedlings after their desiccation. Pretreatment of the seedlings with melatonin reduced this index in the hypocotyls down to the level of control plants (Fig. 4).
Effect of desiccation and treatment with melatonin on MDA content in (1) hypocotyls and (2) roots of 5-day-old seedlings. Experimental variants: I—control seedlings; II—melatonin-treated seedlings; III—desiccated seedlings; IV—melatonin-treated desiccated seedlings. Means and their standard deviations are reported. The statistical significance was estimated with Tukey’s test in the ANOVA program. The means significantly different at P ≤ 0.05 are indicated with different letters.
The roots of the control seedlings contained more than twofold higher amounts of MDA than their hypocotyls. Dehydration stimulated the oxidative stress that was indicated as a 15–17% rise in the MDA content in the hypocotyls and roots. Treatment with melatonin, combined with desiccation, further increased the MDA content in the roots (by 27%) and, inversely, decreased it down to nearly control level in the hypocotyls (Fig. 4). Therefore, melatonin afforded antipodal effects on the oxidative stress in the hypocotyls and roots of lupine seedlings. Its action was antioxidative in the hypocotyls and prooxidative in the roots.
DISCUSSION
Dehydration, as an example of abiotic stresses, considerably alters plant metabolism depending on duration and speed of water losses from tissues of a seedling. Under the influence of AAF, the usual plant strategy involves abatement of metabolic activity and slowing down the processes that are not vitally crucial. Growth belongs to such processes [3, 10, 22]. Its retardation reflects rearrangements within the network of plant metabolic reactions. We revealed earlier that the mitochondrial membranes, isolated from desiccated pea seedlings, display a large fall in the ratio of the total content of unsaturated fatty acids to a total content of saturated FAs. In addition, the unsaturation index of the FAs containing 18 carbon atoms decreased [23]. It is important that this extreme factor causes the structural changes in the mitochondrial membranes that correlate with the functional changes in mitochondrial activity. In this regard, the decline in the oxidation rate of the NAD-dependent substrate (malate) is especially pronounced. The present study demonstrates that the water loss from the tissues of the 5-day-old lupine seedlings (Fig. 1) is also accompanied by a retardation of the growth of hypocotyls (Table 1). An inhibition of malate oxidation in state 3 also occurs in the mitochondria of hypocotyls mainly due to suppression of the CP activity (Fig. 2).
The root tissues, translocated to air medium, experienced much more severe water deficit than the hypocotyl tissues (Fig. 1). The mitochondria isolated from such roots manifested significantly suppressed functional activity, namely, malate and succinate oxidation in state 3 and RC value (Fig. 3). The suppression of the activities of the cytochrome and alternative pathways of electron transport might shift the balance of ROS towards their accumulation in the cells and mitochondria that was evidenced by MDA accumulation in the tissues of hypocotyls and roots (Fig. 4).
It is interesting to note that the roots of the desiccated seedlings continued growing and that even more intensively than that of the normally watered seedlings (Table 1). This phenomenon—accelerated root elongation under lack of water—has also been reported earlier [24, 25]. It is physiologically justified, because water supply becomes a vital problem for the plant under these conditions. Here, the growth might be associated with the cell elongation rather than root mass accumulation [26]. It is also found that the growth retardation is a metabolically controlled process under drought. Here, shoot and root growth is regulated by hormones, in particular, ABA and ethylene [24].
The desiccation of the 4-day-old seedlings, combined with the application of melatonin, favored the rise in water content of the hypocotyl tissues (Fig. 1). Besides, this treatment prevented the decrease in the oxidation rate of malate and succinate in the presence of ADP (in state 3). It also prevented the inhibition of the CP activity and, thus, positively influenced the functional activity of mitochondria (Fig. 2). Simultaneously, the seedling treatment with melatonin reduced POL (Fig. 4) and accelerated the growth of hypocotyls whose length approached to the control values (Table 1).
Although the application of melatonin increased the water content of the roots to some extent (Fig. 1), it also stimulated ROS formation entailing POL intensification in the roots (Fig. 4). In addition, the treatment with melatonin did not favor the maintenance of the mitochondrial oxidative and phosphorilating activities upon oxidation of malate or succinate (Fig. 3). As a result, melatonin retarded the growth of the desiccated roots (Table 1). Therefore, in contrast to hypocotyls, the contact of lupine seedlings with exogenous melatonin did not attenuate the suppression of mitochondrial activity under drought conditions.
It is still hard to explain why melatonin influences in such contrasting manner the respiration of mitochondria isolated from hypocotyls and roots of lupine. The facts have accumulated in literature that treatments with this compound may bring about not only different but sometimes opposed consequences to physiological processes in the plants. The particular outcome depends on melatonin concentration, conditions of its action, and specificity of metabolism of plant organisms and their organs. In this respect, melatonin is similar to different phytohormones.
It is reported that melatonin, while performing the auxin-like activity, stimulates the growth of hypocotyls of lupine and coleoptiles of wheat, oat, and barley. This action occurs at micromolar concentrations of melatonin, which, however, inhibits the growth processes at higher concentrations [27–29]. The root growth is affected by melatonin in a concentration-dependent manner [28, 29]. This hormone not only regulates the growth processes but also interacts with other substances of a hormonal nature [29]. Debnach et al. [30] point to the fact that melatonin and auxin are synthesized from common precursors. For this reason, both hormones may express the same functional activity under stress conditions, in particular, drought. Nevertheless, mechanisms of reciprocal regulation and signal transduction between melatonin and other hormones are so far uncertain under stress impact.
Interactions of melatonin with the mitochondrial respiratory system of the plants are also inadequately explored. Its antioxidant function is well documented, including on isolated plant mitochondria [3, 31]. Our results demonstrate that melatonin promotes the adaptation of the hypocotyl cells to dehydration by, in particular, suppressing POL (Fig. 4), and, thus, preventing dysfunction of cellular membranes. The melatonin-exerted maintenance of metabolic state of the hypocotyl mitochondria is expressed not only in sustaining a high rate of substrate oxidation in CP but also in activation of alternative oxidase, which can also fulfill antioxidant function under stress conditions [1, 12]. The effect of melatonin on mitochondrial functions was quite different in the root cells. In our experiments, root dehydration strongly suppressed the mitochondrial metabolism. Here, the treatment with melatonin aggravated rather than mitigated the consequences of this extreme factor, because the phosphorilating activity of these organelles was completely blocked. In this case, some acceleration of substrate oxidation in state 3 was not accompanied by AP activation, which might accomplish antioxidant function that was reported by Turk and Genizel [1]. Therefore, our study demonstrates that melatonin, under lack of water, does not act as an antioxidant towards roots of lupine but, vice versa, enhances POL, which was evidenced to by accumulation of MDA in this organ.
The mechanism of a feasible prooxidant action of melatonin was investigated on isolated animal mitochondria [2, 6, 32]. This action was revealed to usually occur at its rather high concentration (above 1 µM). For instance, melatonin at micromolar concentrations stimulates ROS formation in the complex III (ubiquinone-cytochrome c reductase) in kidney mitochondria [32]. Lee and Back [7] found that the products of melatonin conversion—2-hydroxymelatonin and 3-hydroxymelatonin—can fulfill their specific physiological functions in the plants, as opposed to animals. Thus, the first product causes a burst of ROS formation through stimulation of NADPH oxidase; this is not pertinent to melatonin. Wang et al. [6] showed that, in the system containing quercetin and Сu2+, melatonin induces formation of ROS because of a reduction in copper ions. Another example of a negative effect of melatonin on ROS reactions in the plants is represented by suppression of the growth of apple tree due to down-regulation of fructokinase-2 gene expression [33]. Furthermore, Sarti et al. [34] revealed that 1 nM melatonin elevates the NO content that leads to diminishing the efficiency of oxidative phosphorilation, dissipation of membrane potential, and shift of the cellular metabolism towards glycolysis. It should be elucidated whether such a mode of action of melatonin is valid for plant mitochondria.
Therefore, this study has found for the first time that, upon treatment of lupine seedlings with melatonin under drought conditions, this hormone exerts opposite influences on the hypocotyl and root as to their growth, POL level, and mitochondrial respiration. In other words, melatonin acted as an antioxidant towards hypocotyl and a prooxidant against root.
Change history
08 May 2023
An Erratum to this paper has been published: https://doi.org/10.1134/S1021443723330026
REFERENCES
Turk, H. and Genisel, M., Melatonin-related mitochondrial respiration responses are associated with growth promotion and cold tolerance in plants, Cryobiology, 2020, vol. 92, p. 76. https://doi.org/10.1016/j.cryobiol.2019.11.006
Agathokleus, E., Kitao, M., and Calabrese, E., New insight into the role of melatonin in plants and animals, Chemico-biological interactions, 2019, vol. 299, p. 163. https://doi.org/10.1016/j.cbi.2018.12.008
Tiwari, R.K., Lal, M.K., Kumar, R., Chourasia, K.N., Naga, K.C., Kumar, D., Das, S.K., and Zinta, G., Mechanistic insights on melatonin-mediated drought stress mitigation in plants, Physiol. Plant., 2021, vol. 172, p. 1212. https://doi.org/10.1111/ppl.13307
Wei, J., Li, D.-X., Zhang, J.-R., Shan, C., Rengel, Z., Song, Z.-B., and Chen, Q., Phytomelatonin receptor PMTR1-mediated signaling regulates stomatal closure in Arabidopsis thaliana, J. Pineal Res., 2018, vol. 65, e12500. https://doi.org/10.1111/jpi.12500
Arnao, M.B. and Hernandez-Ruiz, J. Melatonin: A New plant hormone and/or a plant master regulator?, Trends Plant Sci., 2019, vol. 24, p. 38. https://doi.org/10.1016/j.tplants.2018.10.010
Wang, J., Wang, X., He, Y., Jia, L., Yang, C.S., Reiter, R.J., and Zhang J., Antioxidant and pro-oxidant activities of melatonin in the presence of copper and polyphenols in vitro and in vivo, Cells, 2019, vol. 8, p. 903. https://doi.org/10.3390/cells8080903
Lee, H.Y. and Back, K., 2-hydroxymelatonin, rather than melatonin, is responsible for RBOH-dependent reactive oxygen species production leading to premature senescence in plants, Antioxidants, 2021, vol. 10, p. 1. https://doi.org/10.3390/antiox10111728
Hernandez, I.G., Gomez, F.V., Cerutti, S., Arana, M.V., and Silva, M.F., Melatonin in Arabidopsis thaliana acts as plant growth regulator at low concentrations and preserves seed viability at high concentrations, Plant Physiol. Biochem., 2015, vol. 94, p. 191. https://doi.org/10.1016/j.plaphy.2015.06.011
Sharma P., Dubey R.S. Drought induces oxidative stress and enhances the activities of antioxidant enzymes in growing rice seedlings, Plant Growth Regul. 2005. V. 46. P. 209. https://doi.org/10.1007/s10725-005-0002-2
Atkin, O.K. and Macherel, D., The crucial role of plant mitochondria in orchestrating drought tolerance, Ann. Bot., 2008, vol. 103, p. 581. https://doi.org/10.1093/aob/mcn094
Andreyev, A.Y., Kushnareva, Y.E., Murphy, A.N., and Starkov, A.A., Mitochondrial ROS metabolism: 10 Years later, Biochem. Moscow, 2015, vol. 80, p. 517. https://doi.org/10.1134/S0006297915050028
Vanlerberghe, G.C., Alternative oxidase: a mitochondrial respiratory pathway to maintain metabolic and signaling homeostasis during abiotic and biotic stress in plants, Int. J. Mol. Sci., 2013, vol. 14, p. 6805. https://doi.org/10.3390/ijms14046805
Sharma, A. and Zheng, B., Melatonin mediated regulation of drought stress: physiological and molecular aspects, Plants, 2019, vol. 8, p. 190. https://doi.org/10.3390/plants8070190
Flexas, J., Bota, J., Galme, S.J., Medrano, H., and Ribas-Carbo, M., Keeping a positive carbon balance under adverse conditions: responses of photosynthesis and respiration to water stress, Phys Plant., 2006, vol. 127, p. 343. https://doi.org/10.1111/j.1399-3054.2005.00621.x
Liu, H.-S., Li, F.-M., and Xu, H., Deficiency of water can enhance root respiration rate of drought-sensitive but not drought-tolerant spring wheat, Agric. Water Manag., 2004, vol. 64, p. 41. https://doi.org/10.1016/S0378-3774(03)00143-4
Kirschbaum, M.U.F., Recovery of photosynthesis from water stress in Eucalyptus pauciflora – a process in two stages, Plant Cell Environ., 1988, vol. 11, p. 685. https://doi.org/10.1111/j.1365-3040.1988.tb01151.x
Acuna-Castroviejo, D., Escames, G., Leon, J., Carazo, A., and Khaldy, H., Mitochondrial regulation by melatonin and its metabolites, Adv. Exp. Med. Biol., 2003, vol. 527, p. 549. https://doi.org/10.1007/978-1-4615-0135-0_63
Hardeland, R., Melatonin and the electron transport chain, Cell. Mol. Life Sci., 2017, vol. 74, p. 3883. https://doi.org/10.1007/s00018-017-2615-9
Shugaev, A.G., Butsanets, P.A., Andreev, I.M., and Shugaeva, N.A., Effect of salicylic acid on the metabolic activity of plant mitochondria, Russ. J. Plant Physiol., 2014, vol. 61, p. 520. https://doi.org/10.1134/S1021443714040189
McDonald, A.E., Sieger, S.M., and Vanlerberhe, G.C., Methods and approaches to study plant mitochondrial oxidase, Physiol. Plant., 2002, vol. 116, p. 135. https://doi.org/10.1034/j.1399-3054.2002.1160201.x
Schmitt, N. and Dizengremel, P., Effect of osmotic stress on mitochondria isolated from etiolated mung bean and sorghum seedlings, Plant Physiol. Biochem., 1989, vol. 27, p. 17.
Hodges, D.M., DeLong, J.M., Forney, C.F., and Prange, R.K., Improving the thiobarbituric acid-reactive-substances assay for estimating lipid peroxidation in plant tissues containing anthocyanin and other interfering compounds, Planta, 1999, vol. 207, p. 604. https://doi.org/10.1007/s004250050524
Generozova, I.P. and Shugaev, A.G., Respiration metabolism in mitochondria of pea seedlings of different age under water deficit and rewatering, Russ. J. Plant Physiol, 2012, vol. 59, p. 235. https://doi.org/10.1134/S1021443712020021
Zhigacheva, I.V., Burlakova, E.B., Misharina, T.A., Terenina, M.B., Krikunova, N.I., Generosova, I.P., Shugaev, A.G., and Fattakhov, S.G., Fatty acid composition of membrane lipids and energy metabolism in mitochondria of pea seedlings under water deficit, Russ. J. Plant Physiol., 2013, vol. 60, p. 212. https://doi.org/10.1134/S1021443713010111
Sharp, R.E., Interaction with ethylene: changing views on the role of abscisic acid in root and shoot growth responses to water stress, Plant, Cell Environm., 2002. vol. 25, p. 211. https://doi.org/10.1046/j.1365-3040.2002.00798.x
Farooq, M., Wahid, A., Kobayashi, N., Fujita, D., and Basra, S.M.A., Plant drought stress: effects, mechanisms and management, Agron. Sustain Dev., 2009, vol. 29, p. 185. https://doi.org/10.1051/agro:2008021
Cui, G., Sun, F., Gao, X., Xie, K., Zhang, C.S. Liu, S., and Xi, Y., Proteomic analysis of melatonin‑mediated osmotic tolerance by improving energy metabolism and autophagy in wheat (Triticum aestivum L.), Planta, 2018, vol. 248, p. 69. https://doi.org/10.1007/s00425-018-2881-2
Hernandez-Ruiz, J., Cano, A., and Arnao, M.B., Melatonin: a growth-stimulating compound present in lupin tissues, Planta, 2004, vol. 220, p. 140. https://doi.org/10.1007/s00425-004-1317-3
Chen, Q., Qic, W., Reiter, R.J., Wie, W., and Wan, B., Exogenously applied melatonin stimulates root growth and raises endogenous indoleacetic acid in roots of etiolated seedlings of Brassica juncea, J. Plant Physiol., 2009, vol. 166, p. 324. https://doi.org/10.1016/j.jplph.2008.06.002
Debnath, B., Islam, W., Li, M., Sun, Y., Lu, X., Mitra, S., Hussain, M., Liu, S., and Qiu, D., Melatonin mediates enhancement of stress tolerance in plants, Int. J. Mol. Sci., 2019, vol. 20, p. 1040. https://doi.org/10.3390/ijms20051040
Butsanets, P.A., Baik, A.S., Shugaev, A.G., and Kuznetsov, V.V., Melatonin inhibits peroxide production in plant mitochondria, Dokl. Biochem. Biophys., 2019, vol. 489, p. 367. https://doi.org/10.1134/S1607672919060036
Zhang, H., Zhang, H.-M., Wu, L.-P., Tan, D.-X., K-amat, A., Li, Y.-Q., Katz, M.S., Abboud, H.E., Reiter, R.J., and Zhang, B.-X., Impaired mitochondrial complex III and melatonin responsive reactive oxygen species generation in kidney mitochondria of db/db mice, J. Pin. Res., 2011, vol. 51, p. 338. https://doi.org/10.1111/j.1600-079X.2011.00894.x
Yang, J., Zhang, C., Wang, Z., Sun, S., Zhan, R., Zao, Y., Ma, B., Ma, F., and Li, M., Melatonin-mediated sugar accumulation and growth inhibition in apple plants involves down-regulation of fructokinase 2 expression and activity, Front. Plant Sci, 2019, vol. 19, p. 150. https://doi.org/10.3389/fpls.2019.00150
Sarti, P., Magnifico, M.C., Altieri, F., Mastronicola, D., and Arese, M., New evidence for cross talk between melatonin and mitochondria mediated by a circadian-compatible interaction with nitric oxide, Int. J. Mol. Sci., 2013, vol. 14, p. 11259. https://doi.org/10.3390/ijms140611259
Funding
The work was supported by the Ministry of Education and Science of Russian Federation through State Task no. 121040800153-1 entitled “Mechanisms of Plant Adaptations to Factors of Aridization of Global Climate and Anthropogenic Pollution of the Environment.”
Author information
Authors and Affiliations
Corresponding author
Ethics declarations
The authors declare that they have no conflicts of interest. This article does not contain any work conducted on animal or human participants.
Additional information
Translated by A. Aver’yanov
Abbreviations: AAF—adverse abiotic factors; AP—alternative respiration pathway; CP—cytochrome respiration pathway; WD—water deficit.
The original online version of this article was revised: Due to a retrospective Open Access order.
Rights and permissions
Open Access. This article is licensed under a Creative Commons Attribution 4.0 International License, which permits use, sharing, adaptation, distribution and reproduction in any medium or format, as long as you give appropriate credit to the original author(s) and the source, provide a link to the Creative Commons license, and indicate if changes were made. The images or other third party material in this article are included in the article's Creative Commons license, unless indicated otherwise in a credit line to the material. If material is not included in the article's Creative Commons license and your intended use is not permitted by statutory regulation or exceeds the permitted use, you will need to obtain permission directly from the copyright holder. To view a copy of this license, visit http://creativecommons.org/licenses/by/4.0/.
About this article
Cite this article
Generozova, I.P., Vasilyev, S.V., Butsanets, P.A. et al. Combined Action of Melatonin and Water Deficiency on Growth and MDA Content of Hypocotyls and Roots of Lupine and Respiration of Mitochondria Isolated from These Organs. Russ J Plant Physiol 69, 93 (2022). https://doi.org/10.1134/S1021443722050077
Received:
Revised:
Accepted:
Published:
DOI: https://doi.org/10.1134/S1021443722050077