Abstract
The behavior of groundwater and physical properties of bottom sediment in a riverine-type mangrove forest which is composed of a tidal creek and fringing mangrove swamps were investigated through field observations at Iriomote Island, Japan. After the tidal water ebbed from the swamp surface to the creek, groundwater levels at swamp sites near the creek fell by up to 15 cm by the next flood tide, although the fall was negligible at sites far from the creek and at the open coast outside the mangrove forest. The amount of groundwater discharged to the creek from the swamp depended strongly both on the tidal range and the presence of the steep bank which separates the tidal creek from the fringing mangrove swamp. Based on the fall of groundwater level, the bulk hydraulic conductivity of the swamp was estimated to be 1.5×10−2 cm/s. This value is two to three orders of magnitude larger than that measured in a laboratory using small scale sediment core samples collected in the swamp. These results suggest that the presence of crowded, intricate and large animal burrows as well as sediment layers rich in mangrove humus increases permeability in the mangrove swamp. Further, it is suggested that the mangrove topography with the steep bank of the tidal creek plays an important role which enhances material exchanges through groundwater between the mangrove swamp and the adjacent offshore waters.
Similar content being viewed by others
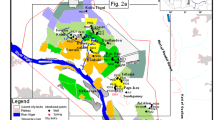
References
Boto K.G. and Bunt J.S. (1981). Tidal export of particulate organic matter from a northern Australian mangrove system. Estuar. Coast. Shelf Sci. 13: 247–255
Childs E.C. 1969. Soil Water Phenomena. Jhon Wiley & Sons Ltd, pp. 493.
Cintron G. and Novelli Y.S. 1984. Methods for studying mangrove structure. In: Snedaker S.C. and Snedaker J.G. (eds), The Mangrove Ecosystem: Research Methods. UNESCO, pp. 91–113.
Gorelick S.M., Freeze R.A., Donahue D. and Keely J.F. (1993). Groundwater Contamination. Lewis Publishers, London, pp.385
Hughes C.E., Binning P. and Willgoose G.R. (1998). Characterization of the hydrology of an estuarine wetland. J. Hydrol. 211: 34–49
Kikkawa H. 1976. The Formulary of Hydraulics. Japan Society of Civil Engineering, Tokyo, pp. 616.
Komiyama A., Moriya H. and Ogino K. (1989). A quantitative analysis of root system of mangrove tree species in Iriomote Island, southern Japan. Galaxea 8: 89–96
Mazda Y., Sato Y., Sawamoto S., Yokochi H. and Wolanski E. (1990a). Links between physical, chemical and biological processes in Bashita-minato, a mangrove swamp in Japan. Estuar. Coast. Shelf Sci. 31: 817–833
Mazda Y., Yokochi H. and Sato Y. (1990b). Groundwater flow in the Bashita-Minato mangrove area, and its influence on water and bottom mud properties. Estuar. Coast. Shelf Sci. 31: 621–638
Mazda Y., Wolanski E., King B., Sase A., Ohtsuka D. and Magi M. (1997). Drag force due to vegetation in mangrove swamps. Mangr. Salt Marsh. 1: 193–199
Mazda Y., Okada S. and Kobashi D. (2005). Tidal-scale hydrodynamics within mangrove swamps. Wetl. Ecol. Manage. 13: 647–655
Ridd P.V. (1996). Flow through animal burrows in mangrove swamps. Estuar. Coast. Shelf Sci. 43: 617–625
Stieglitz T., Ridd P.V. and Muller P. (2000). Passive irrigation and functional morphology of crustacean burrows in a tropical mangrove swamp. Hydrobilogia 421: 69–76
Susilo A. 2004. Groundwater flow in arid tropical tidal wetlands and estuaries. Ph.D Thesis in the School of Mathematical and Physical Sciences, James Cook University, pp.␣152.
Susilo A. and Ridd P.V. (2005). The bulk hydraulic conductivity of mangrove soil perforated with animal burrows. Wetl. Ecol. Manage. 13: 123–133
Wada H. and Takagi T.(1988). Soil–water–plant relationships of mangroves in Thailand. Galaxea 7: 257–270
Wolanski E. and Gardiner R. (1981). Flushing of salt from mangrove swamps. Aust. J. Mar. Freshwater Res. 32: 681–683
Acknowledgements
The authors would like to thank Mr. Tamoo Yamanaka, Manager of the Iriomote Laboratory, Okinawa International Association for Mangroves, for his assistance in the laboratory during our field work at Iriomote Island. We also thank Dr. Peter V. Ridd, James Cook University, Dr.␣Adi Susilo, Brawijaya University, Emeritus Prof. John Allen, University of Reading, Prof. Masaharu Fukue, Tokai University and Paul Canosa, Bilingual Japan for their valuable discussion and advice to improve the manuscript.
Author information
Authors and Affiliations
Corresponding author
Appendices
Appendix A
Cintron and Novelli (1984) have classified the mangrove landform into three types based on their topographical features, i.e. the riverine forest, the fringe forest and the basin forest.
The riverine forest (R-type) is defined as a floodplain along a river drainage or a tidal creek, which is inundated by most high tides and dry up at most low tides. Almost tidal creeks meander sharply and intertwine with each other. Sea waves are hardly found in the swamp, because of reduction due to bottom resistance through the long tidal creek. The fringe forest (F-type) is defined as a shore facing an open sea and is directly exposed to the action not only of tidal water but also of sea waves. The bottom slope of this area continues gradually to the adjacent open coast. The basin forest (B-type) is defined as a partially impounded depression, which is inundated by few high tides during dry season, but high tides during wet season. During dry season, the water level in the basin like a pond continues to decrease very slowly caused by the groundwater flow discharging to open sea due to water level difference between the basin and the open sea.
Appendix B
The specific yield in this study was determined in the laboratory as follows.
At a given site in the field, a cylindrical sediment core was sampled by a pipe sampler (ca. 7 and 15 cm in diameter and height, respectively) so as to be undisturbed and unconsolidated (see Figure B1(a)). Since the specific yield generally varies in time, the core samples above the water table in the field were collected just before the flood tide comes again. The volume (v) of the sample wrapped by a thin film was measured by immersion in a water tank and noting the change in water level from a 0 to a 1 (Figure B1(b)). After the air in the sample was removed, the volume (v′) of the sample was measured by noting the water level a 2 (Figure B1(c)). The specific yield, γE, is calculated by Equation (B1).

Appendix C
When a soil is uniform, the following formulae for hydraulic conductivity are well known (Kikkawa 1976).
The hydraulic conductivity by Zunker’s formula: K Z
The hydraulic conductivity by Slichter’s formula: K S
The hydraulic conductivity by Terzaghi’s formula: K T
where ν is the kinematic viscosity in cm2/s, ɛ the porosity, D 10 the grain-size in cm at which 10% by weight of the grain particles are finer and 90% are coarser, μ t the viscosity in g/cm/s at groundwater temperature (t °C), and χ is given as a function depending on ɛ.
Rights and permissions
About this article
Cite this article
Mazda, Y., Ikeda, Y. Behavior of the groundwater in a riverine-type mangrove forest. Wetlands Ecol Manage 14, 477–488 (2006). https://doi.org/10.1007/s11273-006-9000-z
Received:
Accepted:
Published:
Issue Date:
DOI: https://doi.org/10.1007/s11273-006-9000-z