Abstract
The diversity of vascular plants in temperate floodplain forests varies between biogeographical regions of Europe. Our study aims to identify the key environmental drivers of plant species richness in forests dominated by black alder (Alnus glutinosa) in Central Europe with four regions: Pannonian lowland, Matricum as the southern part of the Western Carpathians, High Western Carpathians and Polish Plain. We analysed plant species richness and quantified 15 environmental characteristics (soil, climatic and landscape characteristics) in 140 vegetation plots (35 per region). We used model-based regression trees to test the influence of predictors on the richness of both native and alien species. The regression tree analysis identified eight significant variables controlling species richness in three regions and all bioregions together but found no significant predictor in Matricum. The analysis of the joint dataset indicates that native plant richness was controlled by the effects of catchment slope, soil reaction and precipitation of the warmest quarter. In contrast, the richness of alien species was influenced by the precipitation of the warmest quarter, soil phosphorous and temperature. The species richness of native plants in the High Western Carpathians was driven by soil reaction and the presence of artificial surfaces around the plots, while the richness trend in the Pannonain lowland was determined by annual temperature. Alien richness was affected by the proportion of agricultural areas in the High Western Carpathians, by the stream power index in the Polish Plain and by soil reaction in the Pannonian lowland. The explanatory power of the tree models ranged from 22 to 36%. Our results suggest that the predictability of the richness patterns is contingent upon the specific regions, which differ in the length of environmental gradients.
Similar content being viewed by others
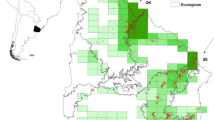
Avoid common mistakes on your manuscript.
Introduction
Species diversity is one of the key measures of ecosystem functioning used in ecological sciences. It is a fundamental feature of biotic communities and an attractive topic in the long-lasting debate of community ecology, as it effectively integrates multiple ecosystem processes and drivers (Wardle et al. 2001; Carli et al. 2018). Determining the number of species present in a plant community and identifying the causal factors behind this pattern is crucial from both theoretical and management perspectives.
Temperate forests range from plant species-poor to species-rich, but these diversity values commonly depend on the geographical scale and habitats analysed (Huntley 1993; Večeřa et al. 2019). Plant species richness in these forests reflects the effects of abiotic factors, biotic interactions, management and human pressures, but the number of plant species is also driven by evolutionary processes. The role of species dispersal strategies for local plant assemblages is also frequently discussed in biodiversity observational studies and syntheses (Zobel 1997; Paillet et al. 2010; Corlett 2016; Schulze et al. 2016; Večeřa et al. 2019; Šipoš et al. 2020). Plant species diversity in floodplain and swamp forests strongly vary, with locally higher richness than in other types of forests such as beech, oak or ravine forests (e.g., Pielech 2021; Hrivnák et al. 2022).
Floodplain forests and alder carrs are dynamic ecosystems with high ecological and conservation values. While riparian forests belong to habitats with strong conservation status according to the European Habitats Directive 92/43/EEC, alder carrs do not share a similar legislative position (see Evans 2010). However broadleaved swamp woodland on non-acidic peat, including alder carrs (EUNIS habitat G1.4), are considered vulnerable habitats at the European level (Janssen et al. 2016). Riparian and swamp alder forests are included in several Central European habitat Red lists (e.g. Rennwald 2000; Chytrý et al. 2019). Both forest types are generally threatened by the synergistic effects of human-induced activities. They are mainly subjected to modifications of natural hydrological regime and associated plant invasions, whose negative effects could be amplified by ongoing climate change (Nilsson & Berggren 2000; Richardson et al. 2007, Attore et al. 2011). These habitats cover a broad scale of flooded and permanently waterlogged broad-leaved forests occurring along water courses, in spring areas and river valleys, as well as in swamps, mires and edges of water reservoirs from lowlands to montane areas (Ellenberg 2009; Douda et al. 2016). Their species richness is influenced by a complex of ecological drivers represented by both local and regional factors, including site heterogeneity and environmental history (Douda et al. 2016; Slezák et al. 2017; Pielech 2021). Furthermore, plant invasions may be increasingly influential in shaping the patterns of species richness within different forest vegetation types. This trend seems to be pronounced especially in riparian forests, which have been identified as one of the most invaded plant communities in Europe (Chytrý et al. 2009; Pyšek et al. 2010; Wagner et al. 2017), including Slovakia (Medvecká et al. 2014; Slezák et al. 2022).
Robust vegetation-plot and ecological data sets of black alder-dominated forests recently collected throughout biogeographical regions of Central Europe provide an opportunity to explore the link between plant species richness and ecological variables. It has been previously found that the High Western Carpathians situated on the border between Slovakia and Poland (belonging to the Alpine bioregion) are the hotspot of native plants in these stands. In contrast, low-altitudinal regions, the Pannonian lowland and southern part of the Western Carpathians (Hungary and Slovakia) and the Polish Plain were considered to be the richest in alien species (Hrivnák et al. 2022). However, the environmental background of these patterns was not evaluated and mechanisms affecting variability in species richness remain poorly understood. Therefore, the present study aimed to identify the environmental factors controlling the species richness in the studied area and especially in the given regions, separately for native and alien plants. In particular, knowledge of the factors that support the species richness of alien plants can help manage activities to limit their occurrence.
Materials and methods
Study sites
The study area encompasses a latitudinal gradient ranging from 45.8085° to 52.6115 °N, with four regions: Pannonian lowland (Pannonia; 45.8085°–47.1271 °N), Matricum as the southern part of the Western Carpathians (MatrWeCa; 47.8565°–48.2861 °N), the High Western Carpathians (HighWeCa; 48.8737°–49.7251 °N) and the Polish Plain (PolPla; 50.9123°–52.6115 °N; Fig. 1). Elevation increases from the Polish and Pannonian lowlands through the Matricum to the (sub)montane areas of the High Western Carpathians. The latter region has the highest amount of precipitation and the lowest air temperature values, while the climatic characteristics of the other regions vary considerably (Table 1). Pannonian lowland is a part of the Pannonian biogeographical region (hereafter referred to only as bioregions) and PolPla refers to the Continental bioregion. MatrWeCa and HighWeCa belong to the Matricum and Carpaticum occidentale within the Alpine bioregion (for more details see Futák 1984; EEA 2019; Cervellini et al. 2020).
The study was conducted in floodplain forests (phytosociological alliance Alnion incanae Pawłowski et al. 1928; class Alno glutinosae-Populetea albae P. Fukarek et Fabijani 1968) and alder carrs (alliance Alnion glutinosae Malcuit 1929; Alnetea glutinosae Br.-Bl. et Tx. ex Westhoff et al. 1946), both dominated by Alnus glutinosa. These forests cover the vegetation of periodically flooded floodplains and waterlogged sites.
Field sampling and laboratory analyses
Vegetation data sampling was carried out in physiognomically and structurally homogeneous old-growth forest stands with a dominant cover of Alnus glutinosa in the tree layer (i.e. canopy cover of more than 50%). We used a uniform sampling size (400 m2) and square or rectangular shape that was driven mainly by the river valleys’ morphology. All vascular plants were recorded in each plot for three layers (tree, shrub and herb). The geographical position and elevation of the plot centre were measured using a Global positioning system receiver.
Altogether, we sampled 173 plots using the traditional European phytosociological approach (Dengler et al. 2008) during the vegetation season (June–August) of 2010–2019. Field sampling was carried out with respect to spatial, environmental and vegetational heterogeneity (Hrivnák et al. 2015, 2022; Slezák et al. 2017). The distance between the two nearest plots of the same vegetation type was usually at least 4 km.
Vegetation records were stored in Turboveg database software (Hennekens & Schaminée 2001) and exported to Juice software (Tichý 2002). Then, 35 plots per region (140 plots in total) were randomly selected to create equal datasets for the regions. Alder carrs with 64% of plots prevailed in the whole dataset, but each region was composed of both types of black alder forests. The proportions of vegetation plots were as follows: Pannonia 71% and 29% (alder carrs and floodplain forests), MatrWeCa 57% and 43%, HighWeCa 46% and 54%, and PolPla 83% and 17%. Species nomenclature was standardised according to the Euro + Med (2006). Vascular plants not determined to species level were deleted (i.e. 25 taxa at the genus level with 57 occurrences), and few taxa were merged into aggregates or a broadly defined level (for details see Hrivnák et al. 2022). The remaining plant species were classified as native and aliens (archaeophytes and neophytes) according to Medvecká et al. (2012) for the MatrWeCa and HighWeCa, Zając and Zając (2011), and Tokarska-Guzik et al. (2012, 2014) for the Polish Plain, and Csiky et al. (2023) for the Pannonian region. Only presence-absence data were used in the analyses. Local alpha (α) diversity (species richness) was defined as the number of plant taxa recorded in a single sampling plot.
Three soil samples were taken from the topsoil mineral horizon without the litter layer at a depth of 0–10 cm and pooled into a single sample per plot to reduce soil heterogeneity. Soil samples were air-dried at laboratory temperature, then crushed and passed through a 2 mm sieve. Soil pH was measured in distilled water (soil/water ratio of 1/5) using a pH meter (WTW Inolab pH 720). Total carbon (C) and nitrogen (N) contents were determined using an NCS-FLASH 1112 analyzer (CE Instruments, UK), and plant-available phosphorus (P) was extracted in the Mehlich II solution and measured by spectrophotometry (AES-ICP). Cations of calcium (Ca), magnesium (Mg) and sodium (Na) were extracted in the Mehlich III solution and determined using an atomic absorption spectro-photometer (SensAA, GBC; UK). Mean annual temperature, total annual precipitation and precipitation of the warmest quarter were retrieved from WorldClim version 2 [http://www.worldclim.org] on a grid background with a spatial resolution of 30 arc-seconds. The catchment slope, stream power index (SPI) and topographic wetness index (WI) were derived from a digital elevation model and calculated in GRASS GIS (Neteler et al. 2012). CORINE Land Cover 2000 basic types in the surroundings of the plots (circle with a radius of 500 m) were obtained from GeoModel Solar (2014). Subsequently, the number of these habitats for a given plot was counted and the proportion of the following habitats, artificial surfaces, agricultural areas, forests and semi-natural habitats, and wetlands, were identified. Altogether 20 environmental variables were selected (Table 1).
Data analysis
To identify significant environmental drivers of native and alien species richness in Central European regions, we used model-based regression trees (Zeileis et al. 2008). Trees with Poisson distribution were used to fit species count data. To avoid overfitting and selection bias during recursive splitting, we applied a conditional inference approach where the results of structural change tests (5% significance level) were employed for variable selection and as the stopping criterion for growing the trees (Hothorn et al. 2006). Measured environmental characteristics were used as potential predictors in the tree models. Initially, we screened the environmental data for collinearity using pairwise Spearman correlations (Supplementary information S1). In cases where two predictors exhibited high collinearity (r ˃ 0.65), only one predictor was retained for further analyses. Elevation, annual precipitation, soil C and Mg, and cover of forests were excluded from the analyses due to collinearity. Finally, 15 environmental (explanatory) variables (Table 1) were used to examine the relationships between species richness and the environment. Trees were independently developed for native and alien species, both for all regions (pooled dataset) and individually for each specific region (Pannonia, MatrWeCa, HighWeCa, and PolPla). Residuals of each model were screened for spatial autocorrelation using correlograms (Bjørnstad & Falck 2001); no significant spatial dependence pattern was observed. The analysis was performed in R (R Core Team 2022) using the packages ggparty (Borkovec and Madin 2019), ncf (Bjørnstad and Falck 2001; Bjørnstad 2022) and partykit (Hothorn and Zeileis 2015).
Results
Altogether 432 plant taxa were recorded in all regions including 57 alien plants (~13%). Native plant species richness was the highest in the region HighWeCa (mean value 45.3, min. 20 species—max. 68 species), followed by MatrWeCa (39.5, 19–80), PolPla (35.6, 13–54) and Pannonian (29.6, 13–50). Alien plant species richness showed an opposite pattern. The highest values in the Matricum (3.4, 0–9) contrasted with the lowest values of the HighWeCa (0.7, 0–3), while species richness in the PolPla (2.4, 0–7) and the Pannonian (2.1, 0–6) was similar.
Soil P and C/N ratio showed similar values across all regions. Soil reaction, concentrations of Ca and Mg were higher in the southern regions (MatrWeCa and Pannonian) compared to the northern regions (HighWeCa and PolPla), but the opposite trend was found for C and N. Cover of artificial surfaces was equally low in all regions. PolPla and Pannonian had higher values than the other regions for agricultural areas and wetlands, respectively. Forests showed the highest and lowest values in MatrWeCa and PolPla. The variability of habitats was similar across regions. The mean catchment slope decreased from HighWeCa and MatrWeCa to Pannonian and PolPla regions. The catchment area increased from PolPla to Pannonia, while MatrWeCa and HighWeCa had similar values for this predictor. Topographic indices differed between the regions studied, i.e. SPI increased from PolPla, Pannonia and HighWeCa to MatrWeCa, whereas WI showed much more homogeneous values (Table 1).
The regression tree analysis identified five significant predictors of species richness in the whole dataset. The diversity of native species was divided into two groups based on slope (6.4°) (Fig. 2a). Steeper plots were further subdivided by soil pH into communities with lower and higher diversity on alkaline and acidic soils, respectively. Plots with gentler slopes were partitioned into richer communities of drier areas (precipitation of the warmest quarter ≤ 267 mm) and more humid sites with lower diversity. Alien species also showed the highest richness under drier climatic conditions (precipitation of the warmest quarter ≤ 227 mm) (Fig. 2b). More humid conditions favoured a higher number of alien species in plots with soil phosphorus levels lower than 13 mg/kg. Almost no aliens dwelled on soils with higher P concentrations, and if they did, they were restricted to plots with annual temperatures above 6.5 °C.
Poisson regression trees partitioning the native species richness (a) and alien species richness (b) in all studied regions based on significant environmental predictors. Threshold values for each partition, probabilities based on structural change tests (p), number of plots in a given group (n) and model determination coefficients (R2) are displayed
Regression tree analysis identified significant predictors of plant species richness in all regions except MatrWeCa. In HighWeCa, soil reaction (≤ 6.2) and the presence of artificial surfaces around the plots correlated with the highest number of native plant species (Fig. 3a). In contrast, alien richness significantly increased with the high proportion of agricultural areas (> 68%) (Fig. 4a). In PolPla, higher alien plant richness was associated with a higher stream power index (> 1.54; Fig. 4b). Native species richness was driven by air temperature (threshold value 10.6 °C) in the Pannonian region, where lower temperature increased the richness pattern (Fig. 3b). Richness of alien plants was higher at sites with soil reaction values below 7.2 (Fig. 4c).
Poisson regression trees partitioning the native species richness in High Western Carpathians (a) and Pannonian lowland (b) based on significant environmental predictors. Threshold values for each partition, probabilities based on structural change tests (p), number of plots in a given group (n) and model determination coefficients (R2) are displayed
Poisson regression trees partitioning the alien species richness in High Western Carpathians (a), Polish Plain (b) and Pannonian lowland (c) based on significant environmental predictors. Threshold values for each partition, probabilities based on structural change tests (p), number of plots in a given group (n) and model determination coefficients (R2) are displayed
Discussion
The use of ecological predictors and regression tree analyses to assess species richness in black alder-dominated forests in Central Europe yielded only partially satisfactory outcomes, especially when considering individual regions. Our models explained between 22 and 36% of the variation in species richness data using recent landscape cover, soil and climate as explanatory variables. These results are in line with the range of variation explained in other recent biodiversity studies conducted in floodplain forests (e.g. Slezák et al. 2017, 2022). However, we are aware that the predictive power of the statistical models is strongly controlled by the set of predictors and the modelling algorithm used (Večeřa et al. 2019).
Predictors responsible for variation in species richness patterns slightly overlapped between the full dataset and the regional subsets. The richness of native and alien plant species was controlled by at least a single predictor in almost all regions studied, with a more structured model recognised for the native richness in the HighWeCa. Our results did not find significant variables only for the richness of native and alien plants in the MatrWeCa, and for the richness of native species in the PolPla. There are several mostly methodological explanations for these findings in individual regions. Although we focused on environmental characteristics known to shape community diversity in the floodplain and swamp forests (e.g. Douda 2010; Slezák et al. 2017, 2020; Hrivnák et al. 2020; Pielech 2021), several additional and not analysed factors may also be important for overall species richness. For example, water regime and associated site productivity measures (e.g. light conditions) contribute to micro-site heterogeneity of floodplain forests (Douda et al. 2012), which in turn could play a significant role in plant species assemblages (Pielech 2021; Slezák et al. 2022). Generally, environmental heterogeneity has been recognized as another important factor governing species richness variation (Stein et al. 2014). We also suppose that among-site heterogeneity in species composition (beta diversity), the length of the environmental gradients studied and the limited number of plots sampled per region may influence our results. These may affect the explanatory power of statistical models and hinder the ability to detect more complex species richness-environmental relationships. Beta diversity explained by Jaccard´s dissimilarity of black alder stands is relatively high in all studied regions, with the lowest value found in the HighWeCa (Hrivnák et al. 2022). The combination of community heterogeneity and broad gradients of several environmental variables in this region, compared with other studied regions (Table 1), leads us to assume that ecological thresholds of species diversity are relatively well-defined in the HighWeCa. In addition, environmental prediction of local-richness patterns could be limited by the number of sampling plots.
Eight environmental variables identified as determinants of thresholds for plant species richness helped us to interpret previous diversity trends in both plant groups, alien and native species. In our case, the effects of four landscape characteristics, namely artificial surfaces, agricultural areas, catchment slope and SPI were accompanied by the well-established role of climate (air temperature and precipitation in the warmest quarter) and two soil characteristics (reaction and concentration of P). The artificial surfaces and agricultural areas are closely related to human activities. Intensely used human-made habitats such as arable land/permanent crops, gardens or grasslands are frequently reported sources of the spread of alien plants into native forest habitats (Chytrý et al. 2005, 2009; Wagner et al. 2017). The plant species composition of secondary habitats considerably differs from plant assemblages in floodplain forests (Chytrý et al. 2020). On the other hand, river corridors promote a rapid species migration (Holeštová and Douda 2022) and many plant species of the artificial habitats thus can successfully colonise various microhabitats developed in riparian areas (Havrdová et al. 2023). For example, the native plants Bellis perennis, Rubus fruticosus s. lat. or the alien species Bidens frondosa, Echinocystis lobata, Impatiens glandulifera, Solidago canadensis and S. gigantea are common species of secondary, man-made habitats and European floodplain forests (Douda et al. 2016; Skokanová et al. 2023), and were also included in our vegetation-plot dataset.
Catchment slope and SPI were important predictors for alien (PolPla) and native (all regions together) plant richness. Both characteristics are proxies for the erosive power of flowing water (Moore et al. 1991) and both are frequently mentioned as predictors of plant richness and composition in riparian forests (e.g. Parker and Bendix 1996; Slezák et al. 2017; Pielech 2021). Large catchment areas with shallow slopes in the continental part of Poland are associated with low erosion activity. This commonly indicates human-altered riparian areas and regulated river networks (e.g. Richardson et al. 2007; Perkins et al. 2016; Slezák et al. 2022), which facilitate the spreading of alien plants. On the contrary, steep slopes in mountainous landscapes compared to flat areas in lowlands, positively influence the richness of native species by creating new habitats (e.g. gravel bars, exposed soil bottoms) that are suitable for different, mainly native stress-tolerant and annual plants. Slopes in river segments also overlap with elevational (climatic) gradients (Supplemetary information S1), which provide additional explanation for richness patterns, for example in the case of alien species (Medvecká et al. 2018). Climate and soil reaction are generally recognised as fundamental ecological drivers shaping plant diversity in forests (Večeřa et al. 2019; Wagner et al. 2021), including forest ecosystems in riparian areas (Douda et al. 2016; Slezák et al. 2017). Plots characterized by low precipitation in the warmest quarter of the year were found to be richer in alien plant species, while plots with low temperatures had higher richness of native plant species. Nevertheless, this fact can be underlined by other environmental characteristics, the biogeography of the territory or by history of landscape utilization, which differ in HighWeCa and Polish Plain (e.g. Lieskovský et al. 2018). Soil reaction plays different roles in richness patterns depending on gradient length, geographical areas, habitat types and evolutionary history (Pärtel 2002; Chytrý et al. 2003). In our study, soil reaction values higher than 6.2 reduced the richness of native species in HighWeCa, while values higher than 7.2 decreased alien plant richness in Pannonia. The pH threshold was set to 7.0 for all regions evaluated together. For individual regions, the threshold values were slightly higher than their mean in the given region and observed richness data thus peaked at mid-values of the pH gradient. Apparently, a broader range of soil pH values recorded in HiWeCa (threshold ± min—max: 6.2 ± 4.3—7.2) and Pannonia (7.2 ± 4.7—7.8) favour abundant groups of plants, native and alien, respectively (see Table 1, Figs. 3a and 4c). It has been previously found that the relationship between species richness and productivity or soil reaction frequently showed an unimodal pattern (Mittelbach et al. 2001; Duprè et al. 2008), with a decreasing trend towards both ends of the gradient (i.e. acidic and highly productive sites). However, this non-linear response of diversity can be confounded by the role of other environmental drivers (Schuster and Diekmann 2003; Palpurina et al. 2017; Slezák et al. 2017). Soil reaction values in the margins of the gradient are associated with eco-physiological constraints that limit numerous vascular plants (see intermediate stress hypothesis, Grime 1979 or physiological tolerance hypothesis, Currie et al. 2004). Soil phosphorus, as an important nutrient with direct implications for plant growth, showed a diversity-reducing effect on alien species in the whole dataset. Biodiversity studies from temperate forests, including floodplain forests, suggest that herb richness decreased with increasing P values. Higher soil P concentrations promote the development and high abundance of only a few strong plant competitors in the local community, whose high biomass production and dominance superiority suppress the richness of co-occurring species (Dumortier et al. 2002; Hrivnák et al. 2015).
Our findings indicate that the predictability of plant richness in black alder-dominated forests is regionally-specific. Species richness was controlled by landscape characteristics, climate and soil-induced drivers, with better predictability found for the richness of native than alien plants. However, the observed environmental thresholds for species diversity patterns should be interpreted with the caution due to discrepancies in the number of vegetation plots corresponding to floodplain and swamp forests in each region. More robust vegetation and environmental datasets would therefore provide more complex insights into the role of predictors operating at local and regional scales. Another shortcoming arises from the geographical framework of our sampling, which was conducted only in the river networks of temperate regions. Arid or semi-arid areas with different water-energy dynamics would likely produce other important variables and thresholds responsible for the variation in species richness data. In spite of these limitations of our study, the present results would be useful tools for a conservation strategy that could guide landscape management in the vicinity of riparian and swamp forests.
Data availability
The datasets generated and/or analysed during the current study are available from the corresponding author on reasonable request.
References
Attorre F, Alfò M, De Sanctis M, Francesconi F, Valenti R, Vitale M, Bruno F (2011) Evaluating the effects of climate change on tree species abundance and distribution in the Italian peninsula. Appl Veg Sci 14:242–255. https://doi.org/10.1111/j.1654-109X.2010.01114.x
Bjørnstad ON, Falck W (2001) Nonparametric spatial covariance functions: estimation and testing. Environ Ecol Stat 8:53–70. https://doi.org/10.1023/A:1009601932481
Bjørnstad ON (2022) ncf: Spatial covariance functions. R package version 1.3–2
Borkovec M, Madin N (2019) ggparty: ‘ggplot’ Visualizations for the ‘partykit’ Package. R package version 1.0.0
Carli E, Frondoni R, Pinna MS, Bacchetta G, Fenu G, Fois M, Marignani M, Puddu S, Blasi C (2018) Spatially assessing plant diversity for conservation: a Mediterranean case study. J Nature Conserv 41:35–43. https://doi.org/10.1016/j.jnc.2017.11.003
Cervellini M, Zannini P, Di Musciano M, Fattorini S, Jiménez-Alfaro B, Rocchini D, Field R, Vetaas OR, Irl SDH, Beierkuhnlein C, Hoffmann S, Fischer J, Casella L, Angelini P, Genovesi P, Nascimbene J, Chiarucci A (2020) A grid-based map for the Biogeographical Regions of Europe. Biodiv Data J 8:e53720. https://doi.org/10.3897/BDJ.8.e53720
Chytrý M, Tichý L, Roleček J (2003) Local and regional patterns of species richness in Central European vegetation types along the pH/calcium gradient. Folia Geobot 38:429–442. https://doi.org/10.1007/BF02803250
Chytrý M, Pyšek P, Tichý L, Knollová I, Danihelka J (2005) Invasions by alien plants in the Czech Republic: a quantitative assessment across habitats. Preslia 77:339–354
Chytrý M, Pyšek P, Wild J, Pino J, Maskell LC, Vilá M (2009) European map of alien plant invasions based on the quantitative assessment across habitats. Divers Distrib 15:98–107. https://doi.org/10.1111/j.1472-4642.2008.00515.x
Chytrý M, Hájek M, Kočí M, Pešout P, Roleček J, Sádlo J, Šumberová K, Sychra J, Boublík K, Douda J, Grulich V, Härtel H, Hédl R, Lustyk P, Navrátilová J, Novák P, Peterka T, Vydrová A, Chobot K (2019) Red list of habitats of the czech republic. EcolIndic 106:e105446. https://doi.org/10.1016/j.ecolind.2019.105446
Chytrý M, Tichý L, Hennekens SM, Knollová I, Janssen JAM, Rodwell JS, Peterka T, Marcenò C, Landucci F, Danihelka J, Hájek M, Dengler J, Novák P, Zukal D, Jiménez-Alfaro B, Mucina L, Abdulhak S, Aćić S, Agrillo E, Attorre F, Bergmeier E, Biurrun I, Boch S, Bölöni J, Bonari G, Braslavskaya T, Bruelheide H, Campos JA, Čarni A, Casella L, Ćuk M, Ćušterevska R, De Bie E, Delbosc P, Demina O, Didukh Y, Dítě D, Dziuba T, Ewald J, Gavilán RG, Gégout J-C, del Galdo GPG, Golub V, Goncharova N, Goral F, Graf U, Indreica A, Isermann M, Jandt U, Jansen F, Jansen J, Jašková A, Jiroušek M, Kącki Z, Kalníková V, Kavgacı A, Khanina L, Korolyuk AY, Kozhevnikova M, Kuzemko A, Küzmič F, Kuznetsov OL, Laiviņš M, Lavrinenko I, Lavrinenko O, Lebedeva M, Lososová Z, Lysenko T, Maciejewski L, Mardari C, Marinšek A, Napreenko MG, Onyshchenko V, Pérez-Haase A, Pielech R, Prokhorov V, Rašomavičius V, Rojo MPR, Rūsiņa S, Schrautzer J, Šibík J, Šilc U, Škvorc Ž, Smagin VA, Stančić Z, Stanisci A, Tikhonova E, Tonteri T, Uogintas D, Valachovič M, Vassilev K, Vynokurov D, Willner W, Yamalov S, Evans D, Lund MP, Spyropoulou R, Tryfon E, Schaminée JHJ (2020) EUNIS habitat classification: expert system, characteristic species combinations and distribution maps of European habitats. Appli Veg Sci 23:648–675. https://doi.org/10.1111/avsc.12519
Corlett RT (2016) Plant diversity in a changing world: Status, trends, and conservation needs. Plant Divers 38:10–16. https://doi.org/10.1016/j.pld.2016.01.001
Csiky J, Balogh L, Dancza I, Gyulai F, Jakab B, Király G, Lehoczky É, Mesterházy A, Pósa P, Wirth T (2023) Checklist of alien vascular plants in Hungary and their invasion biological characteristics. Acta Bot Hung 65:53–72. https://doi.org/10.1556/034.65.2023.1-2.3
Currie DJ, Mittelbach GG, Cornell HV, Field R, Guegan JF, Hawkins BA, Kaufman DM, Kerr JT, Oberdorff T, O’Brien E, Turner JRG (2004) Predictions and tests of climate-based hypotheses of broad-scale variation in taxo-nomic richness. Ecol Letters 7:1121–1134
Dengler J, Chytrý M, Ewald J (2008) Phytosociology. In: Jørgensen SE, Fath BD (eds) Encyclopedia of ecology, vol 4. Elsevier, Oxford, pp 2767–2779
Douda J (2010) The role of landscape configuration in plant composition of floodplain forests across different physiographic areas. J Veg Sci 21:1110–1124. https://doi.org/10.1111/j.1654-1103.2010.01213.x
Douda J, Doudová-Kochánková J, Boublík K, Drašnarová A (2012) Plant species coexistence at local scale in temperate swamp forest: test of habitat heterogeneity hypothesis. Oecologia 169:523–534. https://doi.org/10.1007/s00442-011-2211-x
Douda J, Boublík K, Slezák M, Biurrun I, Nociar J, Havrdová A, Doudová J, Aćić S, Brisse H, Brunet J, Chytrý M, Claessens H, Csiky J, Didukh Y, Dimopoulos P, Dullinger S, Fitzpatrick Ú, Guisan A, Horchler PJ, Hrivnák R, Jandt U, Kącki Z, Kevey B, Landucci F, Lecomte H, Lenoir J, Paal J, Paternoster D, Pauli H, Pielech R, Rodwell JS, Roelandt B, Svenning J, Šibík J, Šilc U, Škvorc Ž, Tsiripidis I, Tzonev RT, Wohlgemuth T, Zimmermann NE (2016) Vegetation classification and biogeography of European floodplain forests and alder carrs. Appl Veg Sci 19:147–163. https://doi.org/10.1111/avsc.12201
Dumortier M, Butaye J, Jacquemyn H, van Camp N, Lust N, Hermy M (2002) Predicting vascular plant species richness of fragmented forests in agricultural landscapes in central Belgium. For Ecol Manag 158:85–102. https://doi.org/10.1016/S0378-1127(00)00674-5
Duprè C, Kolb A, Metzing D (2008) Forest vascular plants as indicators of plant species richness: a data analysis of a flora atlas from northwestern Germany. Plant Biosystems 142:584–593. https://doi.org/10.1080/11263500802410934
EEA (2019) Biogeographical Regions. https://www.eea.europa.eu/data-and-maps/data/biogeographical-regions-europe-3#tab-metadata. Accessed 24 April 2020
Ellenberg H (2009) Vegetation ecology of Central Europe, 4th edn. Cambridge University Press, New York
Euro+Med (2006+) [continuously updated] Euro+Med PlantBase – the information resource for Euro-Mediterranean plant diversity. http://www.europlusmed.org. Accessed 20 February 2024
Evans D (2010) Interpreting the habitats of Annex I: past, present and future. Acta Bot Gallica 157:677–686
Futák J (1984) Fytogeografické členenie Slovenska. In: Bertová L (ed) Flóra Slovenska IV/1. Veda, Bratislava, pp 418–419
Grime JP (1979) Plant strategies and vegetation processes. Wiley, Chichester
Havrdová A, Douda J, Doudová J (2023) Threats, biodiversity drivers and restoration in temperate floodplain forests related to spatial scales. Sci Total Environ 854:158743. https://doi.org/10.1016/j.scitotenv.2022.158743
Hennekens S, Schaminée JJ (2001) TURBOVEG, a comprehensive data base management system for vegetation data. J Veg Sci 12:589–591. https://doi.org/10.2307/3237010
Holeštová A, Douda J (2022) Plant species over-occupancy indicates river valleys are natural corridors for migration. Plant Ecol 223:71–83. https://doi.org/10.1007/s11258-021-01191-9
Hothorn T, Zeileis A (2015) partykit: A Modular toolkit for recursive partytioning in R. J Mach Learn Res 16:3905–3909. https://doi.org/10.5555/2789272.2912120
Hothorn T, Hornik K, Zeileis A (2006) Unbiased recursive partitioning: a conditional inference framework. J Comput Graph Stat 15:651–674. https://doi.org/10.1198/106186006X133933
Hrivnák R, Slezák M, Jarčuška B, Jarolímek I, Kochjarová J (2015) Native and alien plant species richness response to soil nitrogen and phosphorus in temperate floodplain and swamp forests. Forests 6:3501–3513. https://doi.org/10.3390/f6103501
Hrivnák R, Svitok M, Kochjarová J, Jarolímek I, Machava J, Senko D, Slezák M (2020) Drivers of plant species composition in alder-dominated forests with contrasting connectivity. Wetlands Ecol Manag 28:137–150. https://doi.org/10.1007/s11273-019-09700-4
Hrivnák R, Jarčuška B, Jarolímek I, Kochjarová J, Májeková J, Hegedüšová Vantarová K, Slezák M (2022) Comparative diversity of vascular plants in black alder floodplain and swamp forests of Central European biogeographical regions. Biodiv Data J 10:e90281. https://doi.org/10.3897/BDJ.10.e90281
Huntley B (1993) Species richness in North-temperate zone forests. J Biogeogr 20:163–180. https://doi.org/10.2307/2845669
Janssen JAM, Rodwell JS, García Criado M, Gubbay S, Haynes T, Nieto A, Sanders N, Landucci F, Loidi J, Ssymank A, Tahvanainen T, Valderrabano M, Acosta A, Aronsson M, Arts G, Attorre F, Bergmeier E, Bijlsma R-J, Bioret F, Biţă-Nicolae C, Biurrun I, Calix M, Capelo J, Čarni A, Chytrý M, Dengler J, Dimopoulos P, Essl F, Gardfjell H, Gigante D, Giusso del Galdo G, Hájek M, Jansen F, Jansen J, Kapfer J, Mickolajczak A, Molina JA, Molnár Z, Paternoster D, Piernik A, Poulin B, Renaux B, Schaminée JHJ, Šumberová K, Toivonen H, Tonteri T, Tsiripidis I, Tzonev R, Valachovič M (2016) European red list of habitats: part 2. terrestrial and freshwater habitats. Publications Office of the European Union, Luxembourg
Lieskovský J, Kaim D, Balázs P, Boltižiar M, Chmiel M, Grabska E, Király G, Konkoly-Gyuró É, Kozak J, Antalová K, Kuchma T, Mackovčin P, Mojses M, Munteanu C, Ostafin K, Ostapowicz K, Shandra O, Stych P, Radeloff VC (2018) Historical land use dataset of the Carpathian region (1819–1980). J Maps 14:644–651. https://doi.org/10.1080/17445647.2018.1502099
Medvecká J, Kliment J, Májeková J, Halada Ľ, Zaliberová M, Gojdičová E, Feráková V, Jarolímek I (2012) Inventory of alien species of Slovakia. Preslia 84:257–309
Medvecká J, Jarolímek I, Senko D, Svitok M (2014) Fifty years of plant invasion dynamics in Slovakia along a 2,500 m altitudinal gradient. Biol Invasions 16:1627–1638. https://doi.org/10.1007/s10530-013-0596-7
Medvecká M, Jarolímek I, Hegedüšová K, Škodová I, Bazalová D, Botková K, Šibíková M (2018) Forest habitat invasions – Who with whom, where and why. For Ecol Manag 409:468–478. https://doi.org/10.1016/j.foreco.2017.08.038
Mittelbach GG, Steiner CF, Scheiner SM, Gross KL, Reynolds HL, Waide RB, Willig MR, Dodson SI, Gough L (2001) What is the observed relationship between species richness and productivity? Ecology 82:2381–2396. https://doi.org/10.1890/0012-9658
Moore ID, Grayson RB, Ladson AR (1991) Digital terrain modelling: a review of hydrological, geomorphological, and biological applications. Hydrol Process 5:3–30. https://doi.org/10.1002/hyp.3360050103
Neteler M, Bowman MH, Landa M, Metz M (2012) GRASS GIS: a multi-purpose open source GIS. Environl Model Softw 31:124–130. https://doi.org/10.1016/j.envsoft.2011.11.014
Nilsson C, Berggren K (2000) Alterations of riparian ecosys-tems caused by river regulation. Bioscience 50:783–792
Paillet Y, Bergès L, Hjältén J, Ódor P, Avon C, Bernhardt-Römermann M, BIulsma RJ, de Bruyn L, Fuhr M, Grandin U, Kanka R, Lundin L, Luque S, Magura T, Matesanz S, Mészáros I, Sebastià M-T, Schmidt W, Standovár T, Tóthmérész B, Uotila A, Valladares F, Vellak K, Virtanen R (2010) Biodiversity differences between managed and unmanaged forests: meta-analysis of species richness in Europe. Conserv Biol 24:101–112. https://doi.org/10.1111/j.1523-1739.2009.01399.x
Palpurina S, Wagner V, von Wehrden H, Hájek M, Horsák M, Brinkert A, Hölzel N, Wesche K, Kamp J, Hájková P, Danihelka J, Lustyk P, Merunková K, Preislerová Z, Kočí M, Kubešová S, Cherosov M, Ermakov N, German D, Gogoleva P, Lashchinsky N, Martynenko V, Chytrý M (2017) The relationship between plant species richness and soil pH vanishes with increasing aridity across Eurasian dry grasslands. Global Ecol Biogeogr 26:425–434. https://doi.org/10.1111/geb.12549
Parker KC, Bendix J (1996) Landscape-scale geomorphic influences on vegetation patterns in four environments. Phys Geogr 17:113–141
Pärtel M (2002) Local plant diversity patterns and evolutionary history at the regional scale. Ecology 83:2361–2366. https://doi.org/10.1890/0012-9658
Perkins DW, Scott ML, Naumann T (2016) Abundance of invasive, non-native riparian herbs in relation to river regulation. River Res Applic 32:1279–1288. https://doi.org/10.1002/rra.2981
Pielech R (2021) Plant species richness in riparian forests: comparison to other forest ecosystems, longitudinal patterns, role of rare species and topographic factors. For Ecol Manag 496:e119400. https://doi.org/10.1016/j.foreco.2021.119400
Pyšek P, Bacher S, Chytrý M, Jarošík V, Wild J, Celesti-Grapow L, Gassó N, Kenis M, Lambdon PW, Nentwig W, Pergl J, Roques A, Sádlo J, Solarz W, Vilà M, Hulme PE (2010) Contrasting patterns in the invasions of European terrestrial and freshwater habitats by alien plants, insects and vertebrates. Global Ecol Biogeogr 19:317–331. https://doi.org/10.1111/j.1466-8238.2009.00514.x
R Core Team (2022) R: A language and environment for statistical computing. R Foundation for Statistical Computing, Vienna, Austria
Rennwald E (ed.) (2000) Verzeichnis der Pflanzengesellschaften Deutschlands mit Synonymen und Formationseinteilung. Schriftenreihe Vegetationskunde 35: 121–391
Richardson DM, Holmes PM, Esler KJ, Galatowitsch SM, Stromberg JC, Kirkman SP, Pyšek P, Hobbs RJ (2007) Riparian vegetation: degradation, alien plant inva-sions, and restoration prospects. Divers Distrib 13:126–139
Schulze ED, Aas G, Grimm GW, Grossner MM, Walentowski H, Ammer C, Kühn I, Bouriad O, von Gadow K (2016) A review on plant diversity and forest management of European beech forests. Eur J for Res 135:51–67. https://doi.org/10.1007/s10342-015-0922-y
Schuster B, Diekmann M (2003) Changes in species density along the soil pH gradient – evidence from German plant communities. Folia Geobot 38:367–379. https://doi.org/10.1007/BF02803245
Šipoš J, Chudomelová M, Vild O, Macek M, Kopecký M, Szabó P, Hédl R (2020) Plant diversity in deciduous temperate forests reflects interplay among ancient and recent environmental stress. J Veg Sci 31:53–62. https://doi.org/10.1111/jvs.12816
Skokanová K, Španiel S, Šingliarová B, Mereďa P Jr, Hodálová I, Svitok M (2023) Contrasting invasion patterns of two closely related Solidago alien species. J Biogeogr. https://doi.org/10.1111/jbi.14785
Slezák M, Hrivnák R, Machava J (2017) Environmental controls of plant species richness and species composition in black alder floodplain forests of central Slovakia. Tuexenia 37:79–94. https://doi.org/10.14471/2017.37.006
Slezák M, Jarolímek I, Kochjarová J, Hrivnák R (2020) Floodplain forest vegetation in the northern part of the Western Carpathians. Biologia 75:1789–1799. https://doi.org/10.2478/s11756-020-00527-6
Slezák M, Douda J, Šibíková M, Jarolímek I, Senko D, Hrivnák R (2022) Topographic indices predict the diversity of Red List and non-native plant species in human-altered riparian ecosystems. Ecol Indicators 139:e108949. https://doi.org/10.1016/j.ecolind.2022.108949
GeoModel Solar (2014). GIS Data and Maps for Europe, Turkey and North Africa. Technical Report No. 121–01/2014. GeoModel Solar, Bratislava, pp. 1–58.
Stein A, Gerstner K, Kreft H (2014) Environmental heterogeneity as a universal driver of species richness across taxa, biomes and spatial scales. Ecol Lett 17:866–880. https://doi.org/10.1111/ele.12277
Tichý L (2002) JUICE, software for vegetation classification. J Veg Sci 13:451–453. https://doi.org/10.1111/j.1654-1103.2002.tb02069.x
Tokarska-Guzik B, Bzdęga K, Nowak T, Lewandowska A, Gancarek M, Frelich M (2014) Alien plants in Poland: Research directions and putting the results into practice. Biodiv Res Conserv 35:57–74. https://doi.org/10.2478/biorc-2014-0021
Tokarska-Guzik B, Dajdok Z, Zając M, Zając A, Urbisz A, Danielewicz W, Hołdyński C (2012) Rośliny obcego pochodzenia w Polsce ze szczególnym uwzględnieniem gatunków inwazyjnych [Alien plants in Poland with particular reference to invasive species]. Generalna Dyrekcja Ochrony Srodowiska, Warszawa
Večeřa M, Divíšek J, Lenoir J, Jiménez-Alfaro B, Biurrun I, Knollová I, Agrillo E, Campos JA, Čarni A, Jiménez GC, Ćuk M, Dimopoulos P, Ewald J, Fernández-González F, Gégout J, Indreica A, Jandt U, Jansen F, Kącki Z, Rašomavičius V, Řezníčková M, Rodwell JS, Schamineé JHJ, Šilc U, Svenning J, Swacha G, Vassilev K, Venanzoni R, Willner W, Wohlgemuth T, Chytrý M (2019) Alpha diversity of vascular plants in European forests. J Biogeogr 46:1919–1935. https://doi.org/10.1111/jbi.13624
Wagner V, Chytrý M, Jiménez-Alfaro B, Pergl J, Hennekens S, Biurrun I, Knollová I, Berg C, Vassilev K, Rodwell JS, Škvorc Ž, Jandt U, Ewald J, Jansen F, Tsiripidis J, Botta-Dukát Z, Casella L, Attorre F, Rašomavičius V, Ćušterevska R, Schaminée JHJ, Brunet J, Lenoir J, Svenning J-C, Kącki Z, Petrášová-Šibíková M, Šilc U, García-Mijangos I, Campos JA, Fernández-González F, Wohlgemuth T, Onyshchenko V, Pyšek P (2017) Alien plant invasions in European woodlands. Divers Distrib 23:969–981. https://doi.org/10.1111/ddi.12592
Wagner V, Večeřa M, Jiménez-Alfaro B, Pergl J, Lenoir J, Svenning J-C, Pyšek P, Agrillo E, Biurrun I, Campos JA, Ewald J, Fernández-González F, Jandt U, Rašomavičius V, Šilc U, Škvorc Ž, Vassilev K, Wohlgemuth T, Chytrý M (2021) Alien plant invasion hotspots and invasion debt in European woodlands. J Veg Sci 32:e13014. https://doi.org/10.1111/jvs.13014
Wardle DA, Tilman D, Schmid B, Raffaelli D, Huston MA, Hooper DU, Hector A, Grime JP, Bengtsson J, Inchausti P, Naeem S, Loreau M (2001) Biodiversity and ecosystem functioning: current knowledge and future challenges. Science 294(5543):804–808. https://doi.org/10.1126/science.1064088
Zając A, Zając M (2011) Methodical problems in distinguishing the group of archaeophytes. Acta Bot Silesiaca 6:55–62
Zeileis A, Hothorn T, Hornik K (2008) Model-based recursive partitioning. J Comput Graph Stat 17:492–514. https://doi.org/10.1198/106186008X319331
Zobel M (1997) The relative role of species pools in determining plant species richness: an alternative explanation of species coexistence? Trends Ecol Evol 12:266–269. https://doi.org/10.1016/S0169-5347(97)01096-3
Acknowledgements
We would like to thank Dušan Senko and Matúš Hrivnák for the preparation of the climatic, landscape characteristics for the plots and the map, respectively. The study was supported by projects of VEGA grant agency no. 2/0016/19 and 2/0053/23. M. Svitok was also supported by the Operational Programme Integrated Infrastructure (OPII), funded by the ERDF [ITMS 313011T721].
Funding
Open access funding provided by The Ministry of Education, Science, Research and Sport of the Slovak Republic in cooperation with Centre for Scientific and Technical Information of the Slovak Republic. Funding was supported by Science Grant Agency of the Ministry of Education, Science, Research and Sport of the Slovak Republic and the Slovak Academy of Sciences (Vega no. 2/0053/23) and by Operational Programme Integrated Infrastructure (OPII), funded by the ERDF, ITMS 313011T721.
Author information
Authors and Affiliations
Contributions
Richard Hrivnák and Michal Slezák conceived the original idea, designed the study and together with Marek Svitok led the writing, but all co-authors commented on the original and revised version of the manuscript. Marek Svitok performed data analyses. All co-authors contributed to data collection, except Marek Svitok.
Corresponding author
Ethics declarations
Conflict of interests
The authors declare no conflict of interest.
Additional information
Publisher's Note
Springer Nature remains neutral with regard to jurisdictional claims in published maps and institutional affiliations.
Supplementary Information
Below is the link to the electronic supplementary material.
11273_2024_9997_MOESM1_ESM.jpg
Supplementary file1 (JPG 2502 KB)—S1 Correlation matrix of the environmental variables. Pairwise Spearman correlation coefficients are displayed along with correlation ellipses
Rights and permissions
Open Access This article is licensed under a Creative Commons Attribution 4.0 International License, which permits use, sharing, adaptation, distribution and reproduction in any medium or format, as long as you give appropriate credit to the original author(s) and the source, provide a link to the Creative Commons licence, and indicate if changes were made. The images or other third party material in this article are included in the article's Creative Commons licence, unless indicated otherwise in a credit line to the material. If material is not included in the article's Creative Commons licence and your intended use is not permitted by statutory regulation or exceeds the permitted use, you will need to obtain permission directly from the copyright holder. To view a copy of this licence, visit http://creativecommons.org/licenses/by/4.0/.
About this article
Cite this article
Hrivnák, R., Svitok, M., Hegedüšová Vantarová, K. et al. Environmental thresholds for plant species richness of black alder (Alnus glutinosa) forests in Central Europe. Wetlands Ecol Manage (2024). https://doi.org/10.1007/s11273-024-09997-w
Received:
Accepted:
Published:
DOI: https://doi.org/10.1007/s11273-024-09997-w