Abstract
Purpose
To compare the in vitro contractile responses to oxytocin, ergonovine, prostaglandin F2 alpha (PGF2α), and misoprostol in isolated myometrium from non-labouring and labouring pregnant women.
Methods
Myometrial strips obtained from labouring (with or without oxytocin augmentation) and non-labouring women undergoing Cesarean deliveries were subjected to a dose-response testing with oxytocin, ergonovine, PGF2α, or misoprostol (10−10 M to 10−5 M). The amplitude and frequency of contractions, motility index (MI) (amplitude × frequency), and area under the curve during the dose-response period were recorded. The primary outcome was the motility index. Data were analyzed using linear regression models.
Results
We performed 130 experiments in myometrial strips obtained from 46 women. The overall MI (√gram·contractions·10 min−1 [√g·c·10 min−1]) was greatest for oxytocin (mean 5.10 √g·c·10 min−1; 95% confidence interval [CI] 4.70 to 5.50) than for ergonovine (mean 3.46 √g·c·10 min−1; 95% CI 3.13 to 3.80; P < 0.001), PGF2α (mean 2.64 √g·c·10 min−1; 95% CI 2.40 to 2.87; P < 0.001), and misoprostol (2.52 √g·c·10 min−1; 95% CI 2.22 to 2.82; P < 0.001). The MI for oxytocin was significantly lower in augmented labour (mean 4.11 √g·c·10 min−1; 95% CI 3.48 to 4.73) than in non-augmented labour (mean 5.19 √g·c·10 min−1; 95% CI 4.39 to 6.00; P = 0.04) or in absence of labour (mean 5.80 √g·c·10 min−1; 95% CI 5.36 to 6.24; P < 0.001). Nevertheless, in augmented labour, oxytocin still produced superior contractions compared with other uterotonic drugs. Responses to ergonovine, PGF2α, and misoprostol were unaffected by labour or prior exposure to oxytocin.
Conclusion
Oxytocin induces superior myometrial contractions compared with ergonovine, PGF2α, and misoprostol. The effect of oxytocin is reduced in myometrium of women with oxytocin-augmented labour; however, it is still superior to the other uterotonics. This trial was registered at ClinicalTrials.gov: NCT01689311.
Résumé
Objectif
Comparer les réponses contractiles in vitro à l’ocytocine, l’ergonovine, la prostaglandine F2 alpha (PGF2α), et au misoprostol sur des préparations isolées de myomètres provenant de femmes enceintes en cours de travail ou pas.
Méthodes
Des échantillons de myomètre prélevés sur des femmes enceintes, accouchant par césarienne, en cours de travail (avec ou sans accélération par ocytocine) et de femmes n’étant pas en travail, ont été soumis à un test dose-réponse avec de l’ocytocine, de l’ergonovine, de la PGF2α ou du misoprostol (10−10 M à 10−5 M). L’amplitude et la fréquence des contractions, l’indice de mobilité (MI) (amplitude × fréquence) ainsi que la surface sous la courbe au cours de la période dose-réponse, ont été enregistrés. Le principal critère d’évaluation était la racine carrée de l’indice de mobilité. Les données ont été analysées en utilisant des modèles de régression linéaire.
Résultats
Nous avons pratiqué 130 expériences sur des échantillons de myomètre prélevés sur 46 femmes. Le MI global (√gramme·contraction· 10 min−1 [√g·c·10 min−1]) a été plus élevé pour l’ocytocine (moyenne 5,10 √g·c·10 min−1; intervalle de confiance à 95 % [IC] 4,70 à 5,50) que pour l’ergonovine (moyenne 3,46 √g·c·10 min−1; IC à 95 % 3,13 à 3,80; P < 0,001), la PGF2α (moyenne 2,64 √g·c·10 min−1; IC à 95 % 2,40 à 2,87; P < 0,001), et le misoprostol (2,52 √g·c·10 min−1; IC à 95 % 2,22 à 2,82; P < 0,001). Le MI pour l’ocytocine a été significativement plus faible dans le travail accéléré (moyenne 4,11 √g·c·10 min−1; IC à 95 % 3,48 à 4,73) que dans le travail non accéléré (moyenne 5,19 √g·c·10 min−1; IC à 95 % 4,39 à 6,00; P = 0,04) ou qu’en absence de travail (moyenne 5,80 √g·c·10 min−1; IC à 95 % 5,36 à 6,24; P < 0,001). Néanmoins, dans le travail accéléré, l’ocytocine a quand même provoqué des contractions supérieures aux autres utérotoniques. Les réponses à l’ergonovine, à la PGF2α et au misoprostol n’ont pas été modifiées par le travail ou une exposition antérieure à l’ocytocine.
Conclusion
L’ocytocine induit des contractions du myomètre supérieures à celles de l’ergonovine, de la PGF2α, et du misoprostol. L’effet de l’ocytocine est diminué dans le myomètre des femmes en travail accéléré par l’ocytocine mais elle reste néanmoins supérieure aux autres utérotoniques. Cette étude a été enregistrée sur le site www.clinicaltrials.gov: NCT01689311.
Similar content being viewed by others
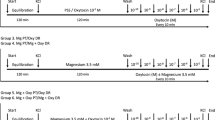
Postpartum hemorrhage (PPH) is one of the leading causes of maternal mortality and morbidity across the globe.1 Since uterine atony is the most common cause of PPH, the administration of uterotonic agents is an integral component of PPH management. Oxytocin is currently the first-line agent used in the prevention and treatment of uterine atony. The use of other uterotonics, such as ergonovine or prostaglandin analogues, is limited by insufficient evidence regarding their dose-dependent pharmacologic effects, their cost, and a perceived lack of safety. The current guidelines for the administration of these drugs are diverse and largely empiric,2-4 and thus, clinical practice varies significantly worldwide. Several publications in the Cochrane Collaboration of Systematic Review compared various uterotonic agents and found inconclusive evidence regarding their relative efficacy. This was due to considerable variability in the trials, insufficient information on the outcomes, and several other confounders that influence uterine tone and blood loss.5-9
Recent evidence suggests that there is an increased incidence of PPH in patients with oxytocin-augmented labours due to uterine atony.10,11 Investigators have suggested that this implies desensitization of oxytocin receptors (OTR) secondary to oxytocin exposure during labour.12-15 Consequently, in the third stage of labour, women may exhibit different responses to oxytocin as well as to other uterotonic drugs, such as ergonovine and prostaglandin analogues. The underlying mechanisms may include variability in receptor availability/affinity and altered intracellular signalling pathways.14,15 Scientific evidence for the comparative efficacy of these drugs in relevant human tissue can help better understand their use in clinical practice.
The objective of our study was to compare the in vitro contractile responses to oxytocin, ergonovine, prostaglandin F2 alpha (PGF2α), and misoprostol in isolated myometrial strips from term non-labouring women and to study the influence of labour (with or without oxytocin augmentation) on the contractile parameters.
Methods
After Research Ethics Board approval (MSH REB # 09-0053-E, dated May 5, 2009), the study was conducted in 46 term pregnant women undergoing Cesarean delivery. We obtained written informed consent from all women who were enrolled in the study. Inclusion criteria were women with gestational age 37-41 weeks requiring Cesarean delivery under regional anesthesia, either as a primary procedure for failure to progress in labour or as an elective procedure for breech presentation or first repeat Cesarean delivery. Women were classified into three different groups: i) non-labouring, ii) non-augmented labouring (those who did not receive oxytocin during labour), and iii) augmented labouring (those who received oxytocin for labour augmentation). Exclusion criteria were general anesthesia, multiple pregnancy, polyhydramnios, chorioamnionitis, placental abnormalities, previous uterine surgery except one previous Cesarean delivery, history or risk factors for PPH, and patients taking medications (such as labetalol, nifedipine, or magnesium sulfate, etc.) that could affect myometrial contractility.
Reagents
Drug solutions were prepared by serial dilutions in sterile double-distilled water. Oxytocin (lyophilized powder), PGF2α (tris salt; powder), ergonovine (maleate; powder), misoprostol (oil), and all salts and reagents used in the preparation of the physiological salt solution (PSS) and 3-(N-Morpholino)propanesulfonic acid hemisodium salt solution (MOPS) were obtained from Sigma-Aldrich Canada Ltd., Oakville, ON, Canada. The MOPS buffer (145 mM NaCl, 4.7 mM KCl, 1.5 mM CaCl2, 1.17 mM MgSO4.7H2O, 1.2 mM NaH2PO4.H2O, 3.0 mM MOPS, 5.0 mM glucose, 2.0 mM pyruvate) and PSS (112 mM NaCl, 25 mM NaHCO3, 1 mM KH2PO4, 5 mM KCl, 1.2 mM MgSO4.7H2O, 11.5 mM glucose, 2.5 mM CaCl2) were prepared in advance and stored at 4-8°C.16-18
Myometrial strip - isolation and preparation
After administration of regional anesthesia as per the routine in the hospital, Cesarean delivery was performed by the obstetrician via a Pfannenstiel incision. A small sliver of myometrium was excised from the upper border of the uterine incision after the delivery of the fetus, just before oxytocin administration. The collected myometrium was immediately immersed in the MOPS buffer, which was placed on ice for tissue dissection. From each sample, four longitudinal myometrial strips (2 × 2 × 10 mm each) were isolated and suspended vertically in individual temperature-controlled organ bath chambers containing 10 mL PSS at pH 7.4. Each strip was clipped between an isometric force transducer and the base of the organ bath chamber (Radnoti® 4-Unit Tissue-Organ Bath System, model 159920, Harvard Apparatus Canada, Saint-Laurent, QC, Canada). The organ bath solution was continuously aerated with 95% oxygen and 5% carbon dioxide at 37°C.16-18
Contractility analysis
As described in previous studies,16-18 each isolated myometrial strip was allowed to equilibrate in PSS at 1 g tension for at least 25 min of spontaneous regular contractions. If the spontaneous contractions did not start immediately, the PSS was changed by flushing every 10-15 min until spontaneous contractions were observed. Strips that failed to contract spontaneously during the initial 20-30 min of mounting were discarded and replaced. After equilibration, each myometrial strip was exposed to an isosmotic 120 mM potassium chloride (KCl) solution to induce the maximum contractile capacity of the tissue. The KCl solution was then drained from the organ baths, and any residual solution was removed by washing three times with PSS.
Thereafter, the myometrial strips were subjected to a dose-response testing with oxytocin, ergonovine, PGF2α, or misoprostol from 10−10 M to 10−5 M concentration (Fig. 1) in a cumulative increase pattern of one log molar increase every ten minutes. At the end of each experiment, the myometrial strips were washed with PSS and once again exposed to the KCl solution to confirm muscle viability. Non-viable strips (i.e., those that did not contract to KCl) were discarded and excluded from analysis. Viable strips were then removed from the apparatus, manually dried, and weighed.
The amplitude and frequency of myometrial contractions were continuously recorded by force displacement transducers connected to a data acquisition system (Biopac System Inc., MP 100 Goleta, CA, USA, with AcqKnowledge 3.9.0 software). After data sets from individual baths were discarded due to failure of the myometrium to contract or technical errors, if any, all other data sets were compared among groups. The data were recorded for 25 min for the equilibration period and for ten minutes for each step during the dose-response period.
Statistical analysis
The amplitude (g) and frequency (number of contractions in ten minutes) of contractions were measured in each group during the dose-response period, and the motility index (amplitude × frequency/10 min; gram·contractions·10 min−1 [g·c·10 min−1]) and the area under the curve (AUC) (integral force; g·sec) were derived to reflect the uterine activity and the strength of contractions, respectively.16 The primary outcome measure was the motility index of contractions across various study groups since it takes into account both the amplitude and the frequency of contractions.
Data analysis was performed with classic linear regression models (maximum likelihood method for estimating parameters) adjusted for repeated measures per sample (i.e., measurements at multiple concentrations) using a compound symmetry covariance structure. General estimating equations (GEE) were generated, including type of drug and patient population (non-labouring, non-augmented labouring, and augmented labouring) during the dose-response period. The outcomes were square-root transformed to adjust for their skewed distribution of both motility index and AUC. The models determined the effect of increasing concentration stratified by drug and were adjusted for baseline value, dry weight of sample, maximum amplitude after KCl before the dose-response period and measures of amplitude, frequency of contractions, motility index, and AUC of baseline contractions during equilibration.
The GEE models allowed for an unequal number of observations on each subject and re-weighted observations through a covariance structure to obtain a balanced regression model in which each subject had the same statistical weight regardless of the number of observations. For this study, a compound symmetry covariance structure was used, which assumes that all observations on a given subject are equally correlated with each other. All statistical analyses were performed using SAS® statistical software v9.2 (The SAS Institute, Cary NC, USA), and the statistician was blinded to various patient groups. All reported P values are two-sided.
Results
Patients were recruited for this study from May 2009 to August 2011. Fifty-three of the 103 eligible patients approached for participation consented to take part in the study, and myometrial samples were obtained from 46 patients. Overall, 130 successful experiments were performed on these samples (Fig. 2).
The clinical characteristics of the patients are shown in Table 1. There was no difference in the average age, height, body mass index, or gestational age among the three groups. The indications for Cesarean delivery were: a) non-labouring – repeat (n = 11), breech (n = 2); b) augmented labouring – failure to progress (n = 15); and c) non-augmented labouring – repeat Cesarean delivery (n = 5), breech (n = 2), fetal problems (n = 2), maternal genital herpes (n = 1); these women went into labour prior to their scheduled Cesarean delivery for the indications above. The duration of labour was almost double in women with augmented labour compared with those in spontaneous labour. All women with augmented labour had received epidural analgesia. The average (SD) maximum oxytocin dose in the augmented labouring patients was 17.9 (9.3) mU·min−1.
The baseline contractile data during the equilibration period and response to KCl are shown in Table 2. The overall (all three patient groups combined) motility index of contractions, when averaged for all concentrations during the dose-response experiments, was highest with oxytocin (mean 5.10 √g·c·10 min−1; 95% confidence interval [CI] 4.70 to 5.50) compared with a) ergonovine (mean 3.46 √g·c·10 min−1; 95% CI 3.13 to 3.80; regression-estimated difference +1.63 √g·c·10 min−1; 95% CI +1.11 to +2.16; P < 0.001); b) PGF2α (mean 2.64 √g·c·10 min−1; 95% CI 2.40 to 2.87; regression-estimated difference +2.46 √g·c·10 min−1; 95% CI +1.97 to +2.95; P < 0.001); and c) misoprostol (mean 2.52 √g·c·10 min−1; 95% CI 2.22 to 2.82; regression-estimated difference +2.58 √g·c·10 min−1; 95% CI +2.03 to +3.13; P < 0.001). A similar effect was observed within each individual group (Tables 3, 4). In all groups combined, ergonovine produced a significantly higher motility index of contractions (mean 3.46 √g·c·10 min−1; 95% CI 3.13 to 3.80) compared with PGF2α (mean 2.64 √g·c·10 min−1; 95% CI 2.40 to 2.87; regression-estimated difference +0.82 √g·c·10 min−1; 95% CI +0.40 to +1.25; P < 0.001) and with misoprostol overall (mean 2.52 √g·c·10 min−1; 95% CI 2.22 to 2.82; regression-estimated difference +0.95 √g·c·10 min−1; 95% CI +0.50 to +1.39; P < 0.001). The difference between PGF2α and misoprostol was not statistically significant (P = 0.49). Table 3 shows the motility index and AUC of contractions across various drugs, and Table 4 shows the estimated differences between them in non-labouring, non-augmented labouring, and augmented labouring women.
Figure 3 shows the modelized dose-response curves of all four drugs in individual non-labouring, non-augmented labouring, and augmented labouring patient groups. The slopes of the dose-response curves were similar in all groups (P = 0.11 for interaction between groups and the study drug).
Motility index of various drugs in non-labouring, non-augmented labouring, augmented labouring women. The dose-response curves for the motility index (√g·contractions·10 min−1) of contractions for oxytocin, ergonovine, PGF2α, and misoprostol in myometrial samples obtained from non-labouring, non-augmented labouring, and augmented labouring women based on modelized data. PGF2α = prostaglandin F2 alpha; Left panel = non-labouring group; middle panel = non-augmented labouring group; and right panel = augmented labouring group
Based on labour type, the motility index for all four drugs combined was significantly lower in augmented labouring (3.13 vs 3.58 √g·c·10 min−1; regression-estimated difference −0.45 √g·c·10 min−1; 95% CI −0.77-to +0.13; P = 0.006) than either non-augmented labouring or non-labouring women, with no differences between non-augmented labouring and non-labouring women (3.56 vs 3.59 √g·c·10 min−1, respectively; regression-estimated difference −0.12 √g·c·10 min−1; 95% CI −0.22 to +0.46; P = 0.49). The motility index for oxytocin was significantly lower in augmented labouring patients (4.11 √g·c·10 min−1; 95% CI 3.48 to 4.73) than in both non-labouring patients (5.80 √g·c·10 min−1; 95% CI 5.36 to 6.24; regression-estimated difference −1.69 √g·c·10 min−1; 95% CI −2.54 to −0.84; P < 0.001) and non-augmented labouring patients (5.19 √g·c·10 min−1; 95% CI 4.39 to 6.00; regression-estimated difference −1.08 √g·c·10 min−1; 95% CI −2.09 to −0.07; P = 0.04) (Table 3; Fig. 4). There was no significant difference in motility index between non-labouring, non-augmented labouring, and augmented labouring women for ergonovine (P = 0.51), PGF2α (P = 0.27), or misoprostol (P = 0.18).
Motility index for oxytocin in non-labouring, non-augmented labouring, and augmented labouring women. The dose-response curves for the motility index (√g·contractions·10 min−1) of contractions for oxytocin in non-labouring, non-augmented labouring; and augmented labouring women based on modelized data. NL = non-labouring; NAL = non-augmented labouring; AL = augmented labouring
Figure 5 shows representative contraction tracings of isolated myometrial strips from non-labouring women with increasing concentrations of various uterotonic drugs. Figure 6 shows contractions with oxytocin alone in the three different groups.
Discussion
Our in vitro study conducted on term pregnant human myometrial strips shows that oxytocin was the most effective amongst the currently available uterotonic drugs used in this investigation. The superior effect of oxytocin is observed in myometrial strips from non-labouring or labouring women, including those of women who received oxytocin for labour augmentation. Ergonovine was the next most potent agent, and its contractile effects were unaffected by labour or oxytocin augmentation. Prostaglandins produced relatively weaker contractions in all groups and were also not affected by prior exposure to oxytocin.
Oxytocin stimulates uterine contractility by binding with myometrial OTR via activation of phospholipase C and release of inositol 1,4,5-triphosphate, 1,2-diacylglycerol, and intracellular calcium.19 Ergonovine maleate directly stimulates contractions of the uterine and vascular smooth muscles via α adrenergic and serotonin receptors, while PGF2α mediates its action by activating the prostaglandin receptors and indirectly by increasing the oxytocin levels.20-22 Dinoprost tromethamine is the tromethamine salt of naturally occurring PGF2α, while 15-methyl-PGF2α, a synthetic analogue of PGF2α, has ten times the contractile effect of PGF2α23,24 and is used to treat refractory uterine atony.2 Misoprostol, a synthetic prostaglandin E1 analogue and an agonist, is used off-label to prevent or treat PPH.25 Misoprostol stimulates contractility via the EP1/ EP3 receptors through a G-protein-mediated increase in phosphoinositol turnover and calcium immobilization or decreased adenylate cyclase and cAMP levels.26 Due to their different mechanisms involving the activation of different receptors and signalling pathways, these agents are likely to induce variable contractility effects. It is not clear from the previous studies if these drugs exhibit dose-dependent contractile responses. Moreover, the changes in the biochemical and physiological adaptations during spontaneous and augmented labour can potentially affect response to these agents.
Oxytocin continues to be the most commonly used uterotonic drug in current clinical practice based on its efficacy and favourable side effect profile.8 Moreover, ergonovine, 15-methyl-PGF2α, and misoprostol have each been shown to reduce the incidence of PPH and/or the need for additional uterotonics when compared with placebo in low-risk women.6-8 In five trials from the Cochrane Collaboration of Systematic Review, prophylactic oxytocin was found to be superior to ergot alkaloids in preventing PPH greater than 500 mL; however, this benefit was not evident when randomized trials with only a low risk of methodologic bias were analyzed.8 Furthermore, based on the data available to date, neither intramuscular prostaglandins nor misoprostol have been shown to be preferable to conventional uterotonics (oxytocin or ergonovine) as part of the management of the third stage of labour.6 The literature is thus deficient and inconclusive regarding the relative uterotonic potencies of all these agents. Furthermore, the considerable variation, bias, and heterogeneity in the available trials do not support a robust recommendation on the choice of uterotonic agents during PPH. In clinical practice, when incrementally larger boluses and infusions of oxytocin have not controlled the hemorrhage secondary to uterine atony, ergonovine and prostaglandin analogues have been administered on an empirical basis. Existing guidelines are not explicit regarding the choice of a second-line agent if the uterus is unresponsive to oxytocin.
Our study clearly shows that oxytocin is the most effective agent in producing uterine contractions in vitro, both in the labouring and non-labouring myometrium. Except for misoprostol, the other uterotonic agents showed a concentration-dependent increase in the contractility responses. The extremely weak response to misoprostol seen in our study requires further evaluation, as misoprostol is currently used clinically to prevent or treat PPH. The dose-response contraction curve with oxytocin shows a plateau at high concentrations, suggesting that excessive doses may have no benefit. This is an important observation since oxytocin also has dose-related cardiovascular side effects.27 The contractility induced by PGF2α appears to be significantly weaker when compared with both oxytocin and ergonovine in equipotent doses. Although our in vitro data suggest that ergonovine may be more effective than PGF2α, how it compares with 15-methyl-PGF2α remains to be clarified. Our results suggest that higher concentrations of ergonovine and PGF2α may be required to produce responses that are equivalent to oxytocin; however, higher doses of these drugs may have unacceptable side effects, such as nausea, hypertension, myocardial ischemia, and bronchospasm.5,6
The myometrial response to oxytocin is determined by the concentration of OTRs, formation of gap junctions, and the sensitivity of the receptors to oxytocin, all of which increase with the onset and progression of labour.28,29 The OTR is a member of the G-protein-coupled receptor family and undergoes homologous desensitization in a time- and concentration-dependent manner following persistent agonist stimulation.12,14,15 The OTR and the corresponding messenger RNA down-regulate significantly, and the oxytocin binding decreases following prolonged oxytocin-augmented and oxytocin-induced labour.15 This oxytocin-induced desensitization phenomenon has been shown in the form of attenuation of oxytocin-induced contractions after pre-treatment with oxytocin in isolated term pregnant rats17 as well as in the human myometrium.30 In the human myometrium, the effect was found to be dependent on the concentration of oxytocin and the duration of pre-exposure and was observed with pre-exposure to oxytocin 10−5 M for at least two hours or 10−8 M for at least four hours.30
In vivo, in non-labouring women, a small loading dose of oxytocin (effective dose [ED]90 = 0.35 IU; 95% CI 0.18 to 0.52) has been shown to be sufficient for producing adequate uterine contractions during elective Cesarean delivery; however, in labouring women after oxytocin augmentation, the loading dose is nine times greater (ED90 = 2.99 IU; 95% CI 2.32 to 3.67).31,32 Despite receiving higher oxytocin doses, these women bleed more than non-labouring women, suggesting the desensitization effect and the need for an alternative drug. A greater incidence of severe PPH secondary to uterine atony is observed when oxytocin is used for labour augmentation in large doses and for long durations rather than with either low-dose or no oxytocin use during labour.10,11,33 These findings support the occurrence of OTR desensitization during prolonged oxytocin exposure in labour, leading to a decrease in oxytocin-mediated function.
Our findings are consistent with our previous in vitro contractility study in rat myometrium that showed a homologous desensitization phenomenon with oxytocin,18 indicating that this desensitization phenomenon is specific for oxytocin and does not affect contractions induced by other uterotonic agents, such as ergonovine, PGF2α or misoprostol, which act through different signalling pathways. Furthermore, despite oxytocin’s desensitization phenomenon, we showed that it provides superior uterine contractions compared with all other uterotonic agents. Whether this is also true in vivo has yet to be determined.
The reported oxytocin plasma levels during pregnancy, labour, and postpartum vary in the literature from 10−12 to 10−9 M.34-38 At our hospital, oxytocin is started at the rate of 2 mU·min−1 for labour augmentation and increased every 30 min by 2 mU·min−1. During delivery, we use infusions rather than boluses; however, 5 or 10 IU bolus administration is still practiced in several places. The concentrations of ergonovine and prostaglandins used in our study are many times higher than plasma levels found in the literature (10−14 to 10−18 M); however, these levels were measured either in non-pregnant women or after variable clinical doses in a population where sex and pregnancy status was not clearly mentioned.39-42 Moreover, none of these levels were obtained following the administration of these drugs after delivery.39-42 In our view, concentrations similar to those used in our study can be achieved with repeated parenteral administration of these uterotonic agents in the postpartum period as part of PPH prevention and treatment. It is possible, however, that in vitro concentrations do not reflect the exact in vivo levels and perhaps represent an overestimation in the experimental settings.
Furthermore, our study has other limitations. Despite the statistically significant observations of our study, they have been made in tissues donated by only 46 women. The in vitro preparation itself may not exhibit effects of the same magnitude as in in vivo settings. It is possible that we may not have achieved the maximum responsiveness for some of the drugs, and testing for a wider range of concentrations may well be required. Nevertheless, we chose this range considering the concentrations achieved with the clinically relevant doses.
In summary, ours is a novel study which provides the in vitro dose-response patterns of myometrial contractions of most of the commercially available uterotonic agents and lays a scientific foundation for future studies in this area. Furthermore, this study validates the oxytocin desensitization phenomenon.
References
Khan KS, Wojdyla D, Say L, Gulmezoglu AM, Van Look PF. WHO analysis of causes of maternal death: a systematic review. Lancet 2006; 367: 1066-74.
Leduc D, Senikas V, Lalonde AB, et al. Active management of the third stage of labour: prevention and treatment of postpartum hemorrhage. J Obstet Gynaecol Can 2009; 31: 980-93.
American College of Obstetricians and Gynecologists. ACOG Practice Bulletin: Clinical management guidelines for obstetrician-gynecologists number 76, October 2006: postpartum hemorrhage. Obstet Gynecol 2006; 108: 1039-47.
Royal College of Obstetricians and Gynaecologists. Prevention and Management of Postpartum Haemorrhage. Green-top Guideline No. 52; May 2009. Available from URL: http://www.rcog.org.uk/files/rcog-corp/GT52PostpartumHaemorrhage0411.pdf (accessed May 2014).
Liabsuetrakul T, Choobun T, Peeyananjarassri K, Islam QM. Prophylactic use of ergot alkaloids in the third stage of labour. Cochrane Database Syst Rev 2007; 2: CD005456.
Gulmezoglu AM, Forna F, Villar J, Hofmeyr GJ. Prostaglandins for preventing postpartum haemorrhage. Cochrane Database Syst Rev 2007; 3: CD000494.
Mousa HA, Alfirevic Z. Treatment for primary postpartum haemorrhage. Cochrane Database Syst Rev 2007; 1: CD003249.
Westhoff G, Cotter AM, Tolosa JE. Prophylactic oxytocin for the third stage of labour to prevent postpartum haemorrhage. Cochrane Database Syst Rev 2013; 10: CD001808.
McDonald S, Abbott JM, Higgins SP. Prophylactic ergometrine-oxytocin versus oxytocin for the third stage of labour. Cochrane Database Syst Rev 2004; 1: CD000201.
Grotegut CA, Paglia MJ, Johnson LN, Thames B, James AH. Oxytocin exposure during labour among women with postpartum hemorrhage secondary to uterine atony. Am J Obstet Gynecol 2011; 204: 56.e1-6.
Belghiti J, Kayem G, Dupont C, Rudigoz RC, Bouvier-Colle MH, Deneux-Tharaux C. Oxytocin during labour and risk of severe postpartum haemorrhage: a population-based, cohort-nested case-control study. BMJ Open 2011; 1: e000514.
Robinson C, Schumann R, Zhang P, Young RC. Oxytocin-induced desensitization of the oxytocin receptor. Am J Obstet Gynecol 2003; 188: 497-502.
Phaneuf S, Asboth G, Carrasco MP, et al. The desensitization of oxytocin receptors in human myometrial cells is accompanied by down-regulation of oxytocin receptor messenger RNA. J Endocrinol 1997; 154: 7-18.
Phaneuf S, Asboth G, Carrasco MP, et al. Desensitization of oxytocin receptors in human myometrium. Hum Reprod Update 1998; 4: 625-33.
Phaneuf S, Rodriguez Linares B, TambyRaja RL, MacKenzie IZ, Lopez Bernal A. Loss of myometrial oxytocin receptors during oxytocin-induced and oxytocin-augmented labour. J Reprod Fertil 2000; 120: 91-7.
Balki M, Kanwal N, Erik-Soussi M, Kingdom J, Carvalho JC. Contractile efficacy of various prostaglandins in pregnant rat myometrium pretreated with oxytocin. Reprod Sci 2012; 19: 968-75.
Magalhaes JK, Carvalho JC, Parkes RK, Kingdom J, Li Y, Balki M. Oxytocin pretreatment decreases oxytocin-induced myometrial contractions in pregnant rats in a concentration-dependent but not time-dependent manner. Reprod Sci 2009; 16: 501-8.
Balki M, Cristian AL, Kingdom J, Carvalho JC. Oxytocin pretreatment of pregnant rat myometrium reduces the efficacy of oxytocin but not of ergonovine maleate or prostaglandin F 2 alpha. Reprod Sci 2010; 17: 269-77.
Gimpl G, Fahrenholz F. The oxytocin receptor system: structure, function, and regulation. Physiol Rev 2001; 81: 629-83.
de Groot AN, van Dongen PW, Vree TB, Hekster YA, van Roosmalen J. Ergot alkaloids. Current status and review of clinical pharmacology and therapeutic use compared with other oxytocics in obstetrics and gynaecology. Drugs 1998; 56: 523-35.
Mirando MA, Prince BC, Tysseling KA, et al. A proposed role for oxytocin in regulation of endometrial prostaglandin F2 alpha secretion during luteolysis in swine. Adv Exp Med Biol 1995; 395: 421-33.
Friel AM, O’Reilly MW, Sexton DJ, Morrison JJ. Specific PGF(2alpha) receptor (FP) antagonism and human uterine contractility in vitro. BJOG 2005; 112: 1034-42.
Hayashi RH, Castillo MS, Noah ML. Management of severe postpartum hemorrhage due to uterine atony using an analogue of prostaglandin F2 alpha. Obstet Gynecol 1981; 58: 426-9.
Takaki N, Tredway D, Toomer P, Murray W, Daane T. Therapeutic abortion of early human gestation with intramuscular 15-methyl prostaglandin F2 alpha. Contraception 1976; 13: 319-32.
Tang OS, Gemzell-Danielsson K, Ho PC. Misoprostol: pharmacokinetic profiles, effects on the uterus and side-effects. Int J Gynaecol Obstet 2007; 99(Suppl 2): S160-7.
Coleman RA, Smith WL, Narumiya S. International Union of Pharmacology classification of prostanoid receptors: properties, distribution, and structure of the receptors and their subtypes. Pharmacol Rev 1994; 46: 205-29.
Pinder AJ, Dresner M, Calow C, Shorten GD, O’Riordan J, Johnson R. Haemodynamic changes caused by oxytocin during caesarean section under spinal anaesthesia. Int J Obstet Anesth 2002; 11: 156-9.
Garfield RE, Kannan MS, Daniel EE. Gap junction formation in myometrium: control by estrogens, progesterone, and prostaglandins. Am J Physiol 1980; 238: C81-9.
Fuchs AR, Fuchs F, Husslein P, Soloff MS. Oxytocin receptors in the human uterus during pregnancy and parturition. Am J Obstet Gynecol 1984; 150: 734-41.
Balki M, Erik-Soussi M, Kingdom J, Carvalho JC. Oxytocin pretreatment attenuates oxytocin-induced contractions in human myometrium in vitro. Anesthesiology 2013; 119: 552-61.
Balki M, Ronayne M, Davies S, et al. Minimum oxytocin dose requirement after cesarean delivery for labor arrest. Obstet Gynecol 2006; 107: 45-50.
Carvalho JC, Balki M, Kingdom J, Windrim R. Oxytocin requirements at elective cesarean delivery: a dose-finding study. Obstet Gynecol 2004; 104: 1005-10.
Crane JM, Young DC. Meta-analysis of low-dose versus high-dose oxytocin for labour induction. J Soc Obstet Gynaecol Can 1998; 20: 1215-23.
Feldman R, Weller A, Zagoory-Sharon O, Levine A. Evidence for a neuroendocrinological foundation of human affiliation: plasma oxytocin levels across pregnancy and the postpartum period predict mother-infant bonding. Psychol Sci 2007; 18: 965-70.
Seitchik J, Amico J, Robinson AG, Castillo M. Oxytocin augmentation of dysfunctional labor. IV. Oxytocin pharmacokinetics. Am J Obstet Gynecol 1984; 150: 225-8.
Kuwabara Y, Takeda S, Mizuno M, Sakamoto S. Oxytocin levels in maternal and fetal plasma, amniotic fluid, and neonatal plasma and urine. Arch Gynecol Obstet 1987; 241: 13-23.
Rahm VA, Hallgren A, Hogberg H, Hurtig I, Odlind V. Plasma oxytocin levels in women during labor with or without epidural analgesia: a prospective study. Acta Obstet Gynecol Scand 2002; 81: 1033-9.
Yamaguchi ET, Cardoso MM, Torres ML, et al. Serum oxytocin concentrations in elective caesarean delivery: a randomized comparison of three infusion regimens. Int J Obstet Anesth 2011; 20: 224-8.
Edlund PO. Determination of ergot alkaloids in plasma by high-performance liquid chromatography and fluorescence detection. J Chromatogr 1981; 226: 107-15.
Powers BL, Wing DA, Carr D, Ewert K, Di Spirito M. Pharmacokinetic profiles of controlled-release hydrogel polymer vaginal inserts containing misoprostol. J Clin Pharmacol 2008; 48: 26-34.
Rayburn WF, Powers BL, Plasse TF, Carr D, Di Spirito M. Pharmacokinetics of a controlled-release misoprostol vaginal insert at term. J Soc Gynecol Investig 2006; 13: 112-7.
Bygdeman M. Pharmacokinetics of prostaglandins. Best Pract Res Clin Obstet Gynaecol 2003; 17: 707-16.
Acknowledgements
The authors acknowledge the Canadian Anesthesiologists’ Society and the Canadian Anesthesia Research Foundation (CARF) for their financial support for this study. The authors also acknowledge Cedric Manlhiot, BSc, Clinical Research Program Manager, Labatt Family Heart Centre, The Hospital for Sick Children, Toronto for statistical analysis of this study. We sincerely thank Dr. Samah Hassan for assisting us with experimentation as well as Dr. Stephen Lye (Associate Director, Research) and Dr. Lee Adamson (Senior Investigator) from the Samuel Lunenfeld Research Institute, Toronto for their continued guidance and support for our research.
Funding
This study was funded by the Canadian Anesthesiologists’ Society Research Award supported by the Canadian Anesthesia Research Foundation (CARF), Canada; and also by Merit Award, Department of Anesthesia, University of Toronto, Ontario, Canada.
Conflicts of interest
The authors report no conflicts of interest.
Disclosure
This paper was presented, in part, in the Best Paper Competition at the 42nd Annual Society for Obstetric Anesthesia and Perinatology Meeting, San Antonio, TX, USA, May 12-16, 2010. The paper was also presented at the Annual Shield’s Research Day, University of Toronto, Toronto, ON, May 7, 2010.
Author information
Authors and Affiliations
Corresponding author
Additional information
Author contributions
Mrinalini Balki, Magda Erik-Soussi, Jose C.A. Carvalho, and John Kingdom participated in the research design and reviewed the manuscript. Mrinalini Balki, Magda Erik-Soussi, and Jose C.A. Carvalho participated in data collection and interpretation and manuscript writing.
Rights and permissions
About this article
Cite this article
Balki, M., Erik-Soussi, M., Kingdom, J. et al. Comparative efficacy of uterotonic agents: in vitro contractions in isolated myometrial strips of labouring and non-labouring women. Can J Anesth/J Can Anesth 61, 808–818 (2014). https://doi.org/10.1007/s12630-014-0190-1
Received:
Accepted:
Published:
Issue Date:
DOI: https://doi.org/10.1007/s12630-014-0190-1