Abstract
Background
The impact of metabolic resistance to insecticides on malaria transmission remains poorly characterised notably through application of entomological parameters. The lack of resistance markers has been one of the limiting factors preventing a robust assessment of such impact. To this end, the present study sought to investigate how the L119F-Gste2 metabolic gene influences entomological parameters underpinning mosquitos’ propensity to transmit Plasmodium spp.
Methods
Longitudinal studies were carried out in Mibellon and Elende, two different eco-climatic settings in Cameroon and mosquitoes were collected using Human Landing Catch (HLC), Centre for Disease Control Light Trap (CDC-LT) and Pyrethrum Spray Catch (PSC) technics. Plasmodium sporozoite parasites were detected by TaqMan and Nested PCR, and blood meal origin by ELISA. The allele-specific PCR (AS-PCR) method was used to genotype the L119F-GSTe2 marker and association with malaria transmission was established by comparing key transmission parameters such as the Entomological Inoculation Rate (EIR) between individuals with different L119F-GSTe2 genotypes.
Results
An. funestus s.l was the predominant malaria vector collected during the entomological survey in both sites (86.6% and 96.4% in Elende and Mibellon, respectively) followed by An. gambiae s.l (7.5% and 2.4%, respectively). Sporozoite infection rates were very high in both collection sites (8.7% and 11% in Elende and Mibellon, respectively). An. funestus s.s exhibited a very high entomological inoculation rate (EIR) (66 ib/h/month and 792 ib/h/year) and was responsible for 98.6% of all malaria transmission events occurring in both sites. The Human Blood Index was also high in both locations (HBI = 94%). An. funestus s.s. mosquitoes with both 119 F/F (RR) and L119F (RS) genotypes had a significantly higher transmission intensity than their susceptible L/L119 (SS) counterparts (IRR = 2.2, 95%CI (1.1–5.2), p = 0.03; IRR = 2.5, 95% CI (1.2–5.8), p = 0.01 respectively).
Conclusion
This study highlights the major role that An. funestus s.s plays in malaria transmission in Cameroon with an aggravation from GSTe2-based metabolic resistance.
Similar content being viewed by others
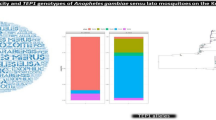
Background
Malaria remains a leading public health concern in Africa, accounting for 29.1% of health facility consultations and 17.2% of deaths in Cameroon [1]. Children under 5 years of age and pregnant women are disproportionately vulnerable; in 2020, hospital morbidity due to malaria in Cameroon was 40.1% among children under 5 and 22.5% for pregnant women [1]. Malaria transmission is highly heterogeneous in the country, with high and perennial parasite transmission occurring in the forest, coastal and humid savanna areas; low parasite transmission in the highlands; and seasonal parasite transmission in the sahelian and dry savanna areas [1]. Plasmodium falciparum is the main parasite responsible for more than 95% of the cases [2] followed by other human-infecting Plasmodium species including P. malariae, P. ovale and P. vivax [3]. Up to 52 Anopheles species have been recorded across the eco-epidemiological zones of the country [4]. Around 16 species have been demonstrated to support the development and spread the human Plasmodium spp., among which 6 main vectors, namely An. gambiae (s.s.), An. coluzzii, An. arabiensis, An. funestus, An. nili and An. moucheti [3, 5].
In Cameroon, over the last decade, significant reduction in malaria burden has been recorded. Morbidity and mortality decreased from 36 to 28% and from 31 to 18.3% respectively [1], mainly due to the scale-up of long-lasting insecticide nets (LLINs), which significantly reduce the number of female malaria vectors population that takes blood meals and subsequently rest indoor [1, 6]. Unfortunately, from 2015 to date, a significant increase in malaria incidence has been observed due to widespread physiological resistance and behavioural changes, with the country still being one of the 11 high worldwide burden countries [7,8,9]. In 2017, all 10 high burden African countries reported an increase in malaria cases compared to the previous year, ranging from an estimated 131 000 more cases in Cameroon to 1.3 million additional cases in Nigeria [9].
Insecticide resistance (IR) is undermining current vector control interventions [10]. In insect populations, IR is predominantly based on enzymatic detoxification as well as by the alteration of insecticide target sites leading to insecticide insensitivity [6, 11]. Metabolic mechanisms are the result of over-expression, either by amplification and/or up-regulation of detoxification genes (Cytochrome P450s, glutathione S-transferases and esterases) [12]. This type of resistance has been consistently reported to be the main driver of pyrethroids and DDT resistance in the malaria vector An. funestus s.l, since no kdr mutation has been detected so far in this species [13, 14]. Despite increasing reports of pyrethroid resistance in malaria vectors, little is known about the impact of metabolic resistance mechanisms on malaria transmission. The lack of resistance markers, particularly for metabolic resistance, has been one of the limiting factors preventing a robust assessment of the resistance impact, notably through application of entomological parameters. The detection of a single amino acid change (L119F) in the glutathione S-transferase epsilon 2 (GSTe2) gene conferring DDT/pyrethroid resistance in An. funestus [15] helped to start assessing the impact of metabolic resistance on An. funestus infectivity [14]. The study revealed that field-collected mosquitoes homozygous resistant for the L119F mutation were more likely to harbour the Plasmodium parasite and develop it to the infective stage, suggesting that, this gene could exacerbate malaria transmission in the field, with important public health consequences. However, it is still unclear how this marker could affect the mosquito vectorial capacity, including other important malaria transmission indices such as mosquito biting rate, parity and the entomological inoculation rate (EIR).
Mosquitoes have also been shown to adapt to a changing environment through behavioural avoidance undermining vector control tools efforts and resulting in the increase of residual transmission [7]. Behavioural modifications include changes in biting time and place, changes in host choice and a shift from endophilic to exophilic behaviour [8]. Vector behavioural changes and resistance selection are important parameters that could play a greater role in the persistent malaria transmission and can only be captured by real-time surveillance [16]. It is now recommended that the deployment of vector control tools is followed by rigorous resistance monitoring and routine entomological and epidemiological surveillance activities [4, 7]. Undertaking such research studies could help to better understand the insecticide resistance status; ecology, behaviour and dynamics of local vector populations; mosquito biting rates; entomological inoculation rates (EIR) and changes in malaria incidence, which could explain residual/persistent malaria transmission and provide avenues for the development of appropriate resistance management strategies. The present study sought to assess how L119F-Gste2 Conferring metabolic resistance influences entomological parameters underpinning An. funestus’ propensity to transmit Plasmodium spp.
Methods
Study site
The study was conducted in two different eco-epidemiological settings in Cameroon: Elende (3°41’57.27’’N, 11°33’28.46’’E) in the humid equatorial forest domain and Mibellon (6o 460 N, 11o 700 E) in the humid savannah (Fig. 1). Both villages are located in the Centre Region, Nyong-Et-Soo Division, Nkolmefou I SubDivision; and in the Adamawa Region, Mayo Banyo Division, Bankim Subdivision, respectively.
Elende is a rural village located about 50 km South-East of Yaoundé (the country’s capital city) and about 2 km from the Yaoundé-Nsimalen International Airport. Located close to the Mefou River, this village is characterised by a classic Guinean equatorial climate with four distinct seasons: a short rainy season from mid-March to the end of June; a short dry season from late June to mid-August; a long rainy season which runs from mid-August to mid-November and a long dry season which runs from mid-November to mid-March [17]. The average temperature is about 21 °C. Most of the agricultural assets are used for subsistence farming, with the main crops being cassava, cocoa, plantain, maize and vegetables. The yield of these agricultural practices is largely enhanced by the intensive use of pesticides [17]. Most houses are made mainly of brick followed by mud walls, with iron sheets and sometimes thatched roofs. Some parameters, such as the strong human influence on the vegetation and the closeness of the village to marshy lands and streams joining major rivers, create temporary and permanent breeding sites for malaria vectors such as An. funestus s.l and Anopheles gambiae s.l which represent the predominant malaria vectors. Pyrethroids- containing LLINs are the main prevention method with a coverage of around 60% [17]. Before the scale up of LLINs in the Region, EIR was known to vary between 100 and 350 infective bites/person/year [4].
Mibellon is a rural village located 350 km from Yaoundé the capital city of Cameroon. The climate is Sudano-Guinean, characterised by a five-month rainy season from March to April and from August to October, and a seven-month dry season extending from November to February and from May to July [18]. The average temperature is about 23 °C. Human activities are mainly fishing, hunting and subsistence farming, including maize, watermelon and coffee plantations. Most houses are made mainly of mud and brick walls, with thatched or iron sheet roofs [19]. Mibellon is close to permanent water bodies, including a lake and swamps, which provide suitable breeding sites for mosquitoe. Two main malaria vector species namely An. gambiae s.l and An. funestus s.l are routinely found in the village, with the latter being the most abundant throughout the year and playing a greater role in malaria transmission [18]. The main vector control approach is LLINs, and the villages benefited from the universal LLIN coverage campaigns in the recent years (2015) before this study. A survey in the area revealed a high use of insecticides in the coffee and watermelon farms [18]. These insecticides are mainly pyrethroids, neonicotinoids and carbamates. The main malaria vectors found there are resistant to pyrethroids and DDT [18]. The region could be classified as belonging to a mesoendemic stratum with perennial malaria parasite transmission.
Household choice
The inhabited households where mosquito collection was undertaken were randomly selected after obtaining the consent from the head of the household. They were assembled according to trapping methods [Human Landing Catches (HLCs, 5 houses), CDC Light Traps (CDC-LTs, 5 houses), Pyrethrum Spray Catches (PSCs, 20 houses)], and a distance of 50–200 m was maintained between each collection point. These houses were made of mud or cement with traditional roof or sheet metal and were representative of the local habitat.
Anopheles species sampling
Wild mosquitoes were caught every month during one year in Elende [from December 2019 to November 2020 except 3 months (from April to June) due to the Covid-19 pandemic]; and bi-monthly the following year in Mibellon (January, March, May, July, September and November 2021) using three collection methods including HLCs, CDC-LTs and PSCs.
Human landing catches
Monthly HLCs were performed inside and outside five houses during three consecutive nights to collect adult mosquitoes landing on human baits. Collections were carried-out between 6:00 pm and 6:00 am to study the change in vector behaviour. A team of four trained volunteers per house (two working during the first half of the night and the others during the second half of the night) carried out the collection, one sitting inside the house and the other on the veranda, with their legs exposed to attract host-seeking mosquitoes. A flashlight was used to locate and dazzle mosquitoes landing on the exposed lower limbs of the collectors, while haemolysis tubes were used to collect mosquitoes before they could bite. The tubes were covered with cotton after individual collection and transferred every hour to custom-made bags for a total of 12 h. Volunteers were offered treatment if they developed symptoms of malaria during the study.
CDC light traps
Ten light traps were installed each month during three consecutive nights, one indoors and another outdoors of five houses in each site at each collection period between 6:00 p.m. and 6:00 a.m. In each bedroom, the trap was hung 1.5 m above the floor near a bed (on the foot side) covered with a mosquito net and where at least one household member was spending the night. Another trap was hung in the same way outside near the window of the same house. Two supervisors were recruited to ensure that the traps set remained operational during all the collection period. Collection boxes were retrieved from traps in each house in the morning from 7:00 am to 8:00 am by the collection team.
Pyrethrum spray catches
The PSCs were carried out each month during morning hours, between 6:00 a.m. and 10:00 a.m. to collect indoor resting mosquitoes for two consecutive days in 10 sleeping rooms. Walls and roofs were sprayed with the commercial pyrethroid + piperonyl butoxide (PBO) insecticide. Houses with open eaves were sprayed from outside through the eaves before entering and spraying indoors. After 10 min, all female anopheline species knocked down by the chemical were collected from the white sheets, transferred inside petri dishes and then sorted according to the physiological state of their abdomen (unfed, blood-fed, half-gravid and gravid). To determine blood meal source, blood-fed female anophelines mosquitoes were kept individually in labelled Eppendorf tubes for further laboratory analysis.
Ovary dissection
Ovaries of unfed Anopheles mosquitoes (about 20% of the total) collected at hourly night intervals with HLC technique were dissected directly after collection on the field, on a sterile microscope slide in distilled water. Binocular dissection microscope at 10X magnification were used to look for the presence of tightly coiled skeins or loose coils, indicative of a nulliparous or parous ovary, respectively.
Mosquito species identification
Collected mosquitoes were sorted into anophelines and culicines genus. All Anopheles mosquitoes were identified morphologically on the field using appropriate identification keys [19]. Once in the laboratory, genomic DNA was extracted from head/thorax and abdomen separately of all collected mosquitoes following the Livak method [20]. The DNA extracted from the head/thorax was quantified using NanoDrop™ spectrophotometer (Thermo Scientifc, Wilmington, USA) and species-specific polymerase chain reaction (PCR) based on the protocols of Koekemoer et al. [21], Santomalazza et al. [22] and Kengne et al. [23] were used to identify sibling species of An. funestus group, An. gambiae complex and An. nili goup respectively.
Estimation of Plasmodium infection rate
The gDNA extracted from the head and thoraces of Anopheles vectors collected indoor and outdoor through HLC method were analysed by the TaqMan assay described by Bass et al. [24], using MX 3005 (Agilent, Santa Clara, CA, USA) to detect the presence of Plasmodium parasites. All the positive samples by TaqMan were used to confirm and discriminate Plasmodium species according to the Nested PCR based of Snounou et al. [25], with slight modifications consisting in using kappa Taq enzyme instead of Ampli Taq [26] and a multiplex detection of Plasmodium species by mixing specific-species primers during the second cycle of amplification.
Genotyping of L119F-GSTe2 mutation in An. funestus s.s
The allele-specific PCR (AS-PCR) method was used for the detection of the L119F-GSTe2 marker (associated with cross-resistance to DDT and pyrethroids) in field collected An. funestus s.s [15, 27]from HLC technic only. This choice was to clearly establish the impact of the marker on all the transmission parameters because mosquitoes collected in the CDC-LT in the morning were usually dead and dissection was not possible. The amplified products corresponding to different genotypes were separated by agarose gel electrophoresis (2%) following the procedure described by Tchouakui et al. [27]. In addition, the potential association between this marker and malaria transmission entomological parameters was assessed using odds ratio and Fisher exact test.
Detection of blood meal source
The engorged abdomens of field mosquitoes collected using PSCs method were used for ELISA blood meal origins. Monoclonal antibodies against human, cow, cattle, pig, horse, chicken, sheep, dog and rat blood were used following the procedure described by Beier et al. [28]
Data analysis
The GraphPad Prism (version 8.0.2) and Stata 16.1 software were used to analyse the data. Pearson’s Chi square tests and Incidence Rate Ratio were used to compare proportions or rates. Approximately 50% of the An. funestus s.s collected from the HLC technique were used to determine the association between the GSTe2 mutation and malaria transmission parameters during each collection month. The other field-collected anopheline species were all analysed only for their entomological parameters (the human biting rate, human blood index, sporozoite infection rate and the entomological inoculation rate), which were estimated using formulas described in the Additional file 1 (Table S1). Association between the GSTe2 mutation and malaria transmission parameters was assessed by comparing the Odds Ratio/Incidence Rate Ratio of malaria transmission parameter between the homozygous resistant (119 F/F), heterozygote (L119F-RS) and homozygous susceptible (L/L119) individuals.
Results
Anopheles mosquito species composition and abundance
A total of 15,946 mosquitoes belonging to the Anopheles genus were sampled in both sites using HLC, CDC_LT and PSC methods. HLC was by far the best collection method regardless the collection site, with 82 and 57% of the total number of Anopheles mosquitoes collected in Elende and Mibellon, respectively. The Anopheline fauna collected in Elende was dominated by An. funestus s.l (8088/9341 [86.6%]) followed by An. gambiae s.l (701/9341 [7.5%]). This was the same in Mibellon (6364/6604 [96.4%]; 158/6604 [2.4%] for the same species, respectively) (Table 1). Others species included An. nili (527/9341 [5.6%] and An. ziemanni (25/9341 [0.3%] in Elende; then An. rufipes (50/6604 [0.7%]) and An. ziemanni (32/6604 [0.5%]) in Mibellon (Table 1). In Elende, molecular identification using PCR revealed the presence of An. funestus s.s (2050/2074 [99%]) and An. leesoni (24/2074 [1%]) as members of the An. funestus group; then An. gambiae s.s (344/403 [85%]) and An. coluzzii (59/403 [15%]) as members of the An. gambiae complex; and An. nili (313/313 [100%]) as the sole member of the An. nili group. In Mibellon, An. funestus s.s (344/347 [99%]), An. leesoni (2/347 [0.6%]) and An. rivulorum (1/347 [0.4%]) were members of the An. funestus group; whereas An. gambiae s.s (89/93 [96%]) and An. arabiensis (4/93 [4%]) were identified as members of the An. gambiae complex (Fig. 2).
Anopheline mosquito species composition after longitudinal survey in Elende and Mibellon from 2019–2021. a & e: Global species composition. b & f: species composition within the Anopheles funestus group. c & g: species composition within the Anopheles gambiae complex. d: species composition within the Anopheles nili group
Parity rate
Regardless of collection site and months, the parity rate of all anopheline mosquitoes collected was very high, ranging from 52 to 100% in Elende (Fig. 3a) and from 58 to 75% in Mibellon (Fig. 3b), with an overall parity rate of 66.1%. An. funestus s.l from Mibellon was significantly more parous than the same species (IRR (Incidence Rate Ratio) = 1.2, 95%CI (1.02–1.3), p = 0.02) or all species combined in Elende (IRR = 1.1, 95%CI (1.01–1.3), p = 0.04) (Additional file 1, Table S2).
Human blood source
The 87.8% (252/287) of mosquitoes tested for blood meal origin were positive for the presence of vertebrate’s blood antigens. In Mibellon, the most frequent blood meal was human (60.2%) followed by dog (33.8%), pig (3.5%) and sheep (2.5%) (Fig. 4b). Mixed blood from 2, 3 and 4 different vertebrate’s host were detected in An. funestus s.l and An. gambiae s.l (Additional file 1, Table S3). In Elende, mosquitoes showed preference only for two hosts, human (94%) the predominant one, and sheep (6%) (Fig. 4a). Unlike to Mibellon, no multiple blood meal source was detected in An. gambiae s.l and An. funestus s.l. All the Anopheles mosquitoes analysed fed mainly on human with a very high human blood index (HBI = 94%) in each site (Table 2). In Mibellon, the highest HBI was found in An. rufipes (100%) followed by An. funestus s.l (97.6%) and An. gambiae s.l (50%). Beside this, a relatively high feeding rate on dog (53%) was also detected (Table 2). In Elende, the highest HBI was found in An. funestus s.l (98%) followed by An. gambiae s.l (75%) (Table 2). No significant difference was observed between HBIs of An. gambiae s.l (IRR = 1.5, 95%CI (0.4–4.7), p = 0.4) and An. funestus s.l (IRR = 1.002, 95%CI (0.7–1.4), p = 0.9) across the two collection sites.
Hourly biting pattern and monthly human biting rates (MHBRs) of anopheline mosquitoes
All Anopheles species collected were aggressive throughout the night both indoors and outdoors. Anopheles biting activity started early in the evening (6 p.m.) and ended very late in the morning (7 a.m.), with some bites recorded until 8 a.m. for An. funestus s.l. irrespective of the collection site (Fig. 5a & d). In Elende, the peak biting activity of An. funestus s.l was recorded during the first part of the night (between 11p.m.-00a.m.), while that of An. gambiae s.l and An. nili s.l was recorded during the second part of the night (both between 00-01a.m.) (Fig. 5b & c). In contrast to Elende, the peak biting activity of An. funestus s.l from Mibellon was recorded during the second part of the night (between 04-05a.m.). Overall, the hourly biting activity of An. funestus s.l., An. gambiae s.l. from Elende; and An. funestus s.l. from Mibellon was significantly higher (IRR = 2, 95%CI [1.7–2.8], p < 0.0001; IRR = 1. 7, 95%CI [1.4-2], p < 0.0001 and IRR = 2.5, 95%CI [2.3–2.7], p < 0.0001 respectively) in the second part of the night (from 00a.m. to 08a.m.) compared to the first part (from 6p.m. to 00a.m.).
In Elende, the highest MHBRs of An. funestus s.l. were observed in January and February 2020 (Fig. 6c), and the mean MHBR was significantly higher indoors (891 b/h/m) compared to outdoors (600 b/h/m) (IRR = 1.5, 95%CI [1.4–1.6], p < 0.001). However, there was no significant difference between the mean indoor (60 b/h/m) and outdoor (63 b/h/m) MHBR of An. gambiae s.l. at the same site (IRR = 1.1, 95%CI [0.9–1.3], p = 0.5). In Mibellon, the highest MHBRs of An. funestus s.l. were observed in September and November 2021 (Fig. 6d). As observed in Elende, the mean MHBRs of An. funestus s.l. from Mibellon were significantly higher indoors (639 b/h/m) compared to outdoors (570 b/h/m) (IRR = 1.2, 95%CI [1.1-3. 6], p = 0.005) and there was no significant difference between the mean indoor (21 b/h/m) and outdoor (12 b/h/m) MHBR of An. gambiae s.l. at the same site (IRR = 1.2, 95%CI [0.8–1.8], p = 0.3). Globally, the MHBR of An. funestus s.l. was significantly higher in Elende (750 b/h/m) than in Mibellon (606 b/h/m) (IRR = 1.2, 95%CI [1.25–1.3], p < 0.001). The same result was observed for An. gambiae s.l. (36 b/h/m and 18 b/h/m in the same site, respectively) (IRR = 3.6, 95%CI [3-4.5], p < 0.001) (Table 3).
Main malariometric indices associated with An. funestus s.l. in Elende and Mibellon from 2019–2021. a & c: Sporozoite infection rate, Monthly human biting rate, and Entomological inoculation rate in Elende; b & d: Sporozoite infection rate, Monthly human biting rate and Entomological inoculation rate in Mibellon
Sporozoite infection rates (SIRs) and entomological inoculation rates (EIRs) of Anopheles funestus s.l. and Anopheles gambiae s.l. mosquitoes collected by human-landing catch
The overall infection rates of An. funestus s.l. mosquitoes by sporozoites of different plasmodial species were very high in both collection sites (8.7 and 11% in Elende and Mibellon, respectively) (Fig. 6a&b), whereas those of An. gambiae s.l. were much lower (2.6 and 2.2% in the same sites, respectively) (Table 3). The comparison between indoor and outdoor SIRs showed that there was no significant difference for An. funestus s.l. (IRR = 1.6, 95%CI [0.9-3], p = 0.07) and An. gambiae s.l. (IRR = 2.9, 95%CI [0.3–146], p = 0.3) from Elende. However, An. funestus s.l. from Mibellon was significantly more infected indoors than outdoors (IRR = 1.5, 95%CI [1.1–2.1], p = 0.01) (Table 3). The SIRs of An. funestus s.l. varied too much according to the months of the survey. In Elende, the SIRs ranged from 1.2 to 23%, with the highest rate detected in July (23%) and March 2020 (17%), whereas in Mibellon, the SIRs varied from 5 to 20%, with the highest rate detected in September (20%) and November 2021 (16%) (Additional file 1, Table S4). However, despite these variations by survey month, the SIR of An. funestus s.l. was not significantly different between the wet and dry seasons (IRR = 1.4, 95%CI [0.8–2.3], p = 0.2; IRR = 1.02, 95%CI [0.7–1.4], p = 0.9 in Elende and Mibellon, respectively). Plasmodium falciparum was by far the predominant species infecting An. funestus s.l. in both sites, accounting for 92 and 70% of total infections in Elende and Mibellon respectively, while P. malariae accounted for 5 and 21% in the same sites. Other species, including P. ovale infection (3%) and P. falciparum/P. malariae co-infection (9%), were detected only in Elende and Mibellon (Fig. 6a&b). Despite differences in the Plasmodium species infecting An. funestus s.l. between sites, there was no significant difference in the overall SIR of An. funestus s.l. between the two study sites (IRR = 1.2, 95%CI [0.9–1.6], p = 0.1). P. falciparum was the only species infecting the salivary gland of An. gambiae s.l. in both sites, in contrast to An. funestus s.l. Globally, in Elende, An. funestus s.l. supported 93% (66/71) of all sporozoite infection events, while An. gambiae s.l. supported only 7% (5/71). However, in Mibellon, 98.8% of sporozoite infection events were supported by An. funestus s.l. while only 1.2% were supported by An. gambiae s.l. Regardless of the site, 97% (236/243) of all sporozoite infection events at both sites were supported by An. funestus s.l. while only 3% were supported by An. gambiae s.l. No infection was detected in the other Anopheles species collected during the study period.
The overall monthly EIR of An. funestus s.l. was very high and exactly equal at both sites (66 ib/h/m), while An. gambiae s.l. was associated with very low monthly malaria transmission intensities at both sites (1.5 and 0.3 ib/h/m in Elende and Mibellon, respectively). Regardless of the site, indoor monthly EIRs (93 and 81 ib/h/m) associated with An. funestus s.l. were significantly higher than outdoor monthly EIR (39 and 41 ib/h/m) (IRR = 2.3, 95%CI [1.9–2.9], p < 0.001; IRR = 1.7, 95%CI [1.4–2.1], p < 0.001 in Elende and Mibellon, respectively) (Table 3). This was not applied to An. gambiae s.l. as no significant difference were observed between indoor and outdoor transmission intensities (Table 3). As with HBRs, EIRs of An. funestus s.l. were also subject to monthly variations, with the highest EIRs observed in the same months as the monthly HBRs in Mibellon, but in different months in Elende, notably July (98 ib/h/m) and March 2020 (94 ib/h/m) (Fig. 6c&d). Despite this latter observation, there was no significant difference between the malaria transmission intensities of An. funestus s.l. recorded during the wet and dry seasons, irrespective of the site (IRR = 1.2, 95%CI [0.9–1.4], p = 0.09; IRR = 1.1, 95%CI [0.9–1.3], p = 0.3 in Elende and Mibellon, respectively). Overall, An. funestus s.l. was responsible for 98.6% of all malaria transmission events at both sites, while An. gambiae s.l. played a minor role (1.4%).
Distribution of the L119F-GSTe2 gene in An. funestus s.s mosquitoes and its influence on malaria transmission in Elende and Mibellon
Following monthly collections, a total of 3630 mosquitoes (1744 and 1886 from Elende and Mibellon respectively) were genotyped for the L119F-Gste2 gene and the annual distribution of each genotype and allele is presented in Fig. 7. Data revealed a significant lower frequency of the 119 F resistant allele in the An. funestus s.s. population from Mibellon (35%) compared to Elende (56%) (IRR = 1.8, 95%IC [1.7-2], p < 0.001).
Association between the L119F-GSTe2 resistant marker and An. funestus’ parity
In Elende, the 69% (399/579) of parous mosquitoes were comprised of 35% (140/399) 119 F/F-RR homozygous resistant, 42% (168/399) L119F-RS heterozygous, and 23% (91/399) L/L119-SS homozygous susceptible, with no significant difference observed in the distribution of the three genotypes in nulliparous mosquitoes (χ2 = 1.6, p = 0.4). The same observation was made in Mibellon (χ2 = 0.1, p = 0.9) (Fig. 8a & b). However, in Mibellon, 119 F/F-RR homozygous resistant vectors showed a tendency to be more nulliparous, whereas the L/L119-SS homozygous susceptible were more parous (Fig. 8b). The same trend was observed in Elende only for 119 F/F-RR mosquitoes (Fig. 8a).
Influence of L119F GSTe2 metabolic resistance on some malariometric indices in Elende and Mibellon. a & c: Association between L119F-Gste2 genotypes and Anopheles funestus’s biting behavior, Parity status, sporozoite infection and biting pattern in Elende; b & d: Association between L119F-Gste2 genotypes and An. funestus’ biting behavior, Parity status, sporozoite infection and biting pattern in Mibellon. RR: 119 F/F homozygous resistant; RS: L119F heterozygous; SS: L/L119 homozygous susceptible
Association between the L119F-GSTe2 resistant marker and An. funestus’ biting behaviour and aggressivity
In Elende, the 48% (837/1744) of mosquitoes collected outdoors were comprised of 35% (297/837) 119 F/F-RR homozygous resistant, 41% (345/837) L119F-RS heterozygous, and 24% (195/837) L/L119-SS homozygous susceptible. A similar distribution of the three genotypes was observed for mosquitoes collected indoor showing no significant difference (χ2 = 0.08, p = 0.9) in biting preference (Fig. 8a). In Mibellon, a similar trend was observed with no significant association between L119F-GSTe2 genotypes and the biting behaviour (χ2 = 0.3, p = 0.86) although 119 F/F-RR homozygous resistant individuals tended to bite more outdoor than indoor (Fig. 8b).
Irrespective to the site, the aggressivity of mosquitoes with all the three genotypes started very early in the evening (06:00 p.m) till late in the morning, with a higher proportion of 119 F/F-RR and L119F-RS mosquitoes recorded at 07:00 a.m compared to the L/L119-SS mosquitoes (Fig. 8c & d). The RR and RS individuals had a similar peak biting time around 04–05 a.m. in both locations whereas it was between 02 and 04 am for the SS mosquitoes.
Association between the L119F-GSTe2 resistant marker and An. funestus’ infectivity and Entomological inoculation rate (EIR)
Regarding the infectious status, in Elende, the 8.7% (66/755) of infected mosquitoes included 40% (26/66) 119 F/F-RR homozygous resistant, 42% (28/66) L119F-RS heterozygous and only 18% (12/66) L/L119-SS homozygous susceptible. As observed with the two first entomological indices, similar distribution of the three genotypes was observed with the opposite index (non-infected status) (χ2 = 0.8, p = 0.6). In Mibellon, there was also no significant association between L119F-GSTe2 genotypes and infectious status (χ2 = 0.3, p = 0.86). Surprisingly, whatever the site, 119 F/F-RR homozygous resistant vectors showed a tendency to be more infected (Fig. 8a & b), whereas the L/L119-SS homozygous susceptible were more often non-infected in Elende (Fig. 8a).
Analysis of the association between L119F-GSTe2 and Entomological Inoculation Rate (EIR) was performed only in Elende because of the high frequency of the 119 F-GSTe2 resistant allele in this location enabling a better comparison. In this location, monthly malaria transmission intensities associated to the 119 F/F-RR (6.6 ib/h/m) and L119F-RS (7.5 ib/h/m) mosquitoes were significantly higher compared to that of L/L119-SS mosquitoes (3 ib/h/m) (IRR = 2.2, 95%CI (1.1–5.2), p = 0.03; IRR = 2.5, 95%CI (1.2–5.8), p = 0.01 respectively). However, there was no significant difference between 119 F/F-RR and L119F-RS mosquitoes (IRR = 0.8, 95%CI (0.5–1.6), p = 0.6) (Table 4).
Discussion
The present study aimed to investigate the impact of GST-based metabolic resistance on the vectorial capacity of the major malaria vector An. funestus through a longitudinal study. The study highlighted the predominant role of An. funestus in the studied locations, a high level of malaria transmission and also revealed that GST-based resistance is increasing malaria transmission.
An. funestus was perennially the predominant species in the two collection sites
Anopheles mosquitoes collected by HLC from both sites were almost exclusively made up of An. funestus s.l. following by An. gambiae s.l confirming previous reports [17, 18]. High abundance of An. funestus s.l was reported in other locations in Cameroon [17, 26, 29], in Kenya [30], Tanzania [31] and could be linked to the presence of permanent large pools of water in both sites known to be their favourite breeding site. The high predominance of An. funestus s.s as shown by molecular identification is similar to the previous studies carried out in the same collection sites [18, 26], and in Tibati and Gounougou (Northern Region) [14, 32]. The dominance of An. funestus s.s in those localities further confirms the extremely high anthropophilic status of this species and its strong potential involvement in disease transmission. With regard to the An. gambiae complex, in Elende, the presence of both An. gambiae s.s. (85%) and An. coluzzii (15%) was consistent with the standard pattern which shows that both species are widely distributed in the country [32, 33]. The presence of An. arabiensis (4%) in Mibellon (Adamawa Region) contrasts the general trend showing so far that this species was restricted to the Northern arid and semi-arid zone of the country [32, 33]. The presence of An. nili s.s as the sole species of the group is similar to the studies conducted in Nyabessang (Southern Region) [34], in the Northern part of the Benin [35], in the Southern and Western forest areas of Côte d’Ivoire [36] and in the Sudano-Guinean Zone of Senegal [37]. Secondary malaria vectors such as An. ziemanni, An. nili s.l and An. rufipes which are better-known as zoophilic species [38, 39] collected in these sites indicate high vector diversity and potential increase risk of malaria transmission.
Entomological indices recorded support a high malaria transmission level in both location
An. funestus s.l, An. gambiae s.l and An. nili s.l all had very high parity rates ranging from 52 to 100%, showing that vectors lived long enough to complete the sporogony cycle of the parasite to become infectious. Similar observations were highlighted by Fondjo et al. [34] in different localities of the country. This could indicate a limited efficacy of vector control tools in these locations potentially associated with escalation of pyrethroid resistance [40].
Malaria transmission dynamic is highly influenced by host preference and feeding behaviour of mosquito vectors. The very high overall HBI (single + mixed) of 94% in each site highlights the strong anthropophagic tendency of the main malaria vectors An. funestus s.s. and An. gambiae s.s. in Cameroon and corroborates with previous reports of Antonio-Nkondjio et al. [41], Tanga et al. [42] and Tabue et al. [39] in the Central, South-west and Northern part of the country. Surprisingly, An. rufipes, previously known as a zoophilic species [19], was found in this study as the only species taking the blood meal solely on humans. The small sample size of An. rufipes in this study could be a limitation of the observed result, therefore no strong conclusions could be drawn. However, studies conducted in the Northern part of the country showed the highly opportunistic role of this species regarding its feeding behaviour associated with a pronounced zoophilic tendency [43, 44]. Considering the fact that its epidemiological role in malaria transmission has been recently highlighted in the country [39, 43], our results suggest that routine entomological surveillance in the area where this species is most abundant should be carried out to update its vectorial capacity. The relatively high feeding on Dog (Index = 53%) observed in Mibellon, suggests that use of insecticide-treated dogs could be a control approach in such areas as other authors have done previously using cattle [45]. The proportion of mixed blood meals was higher than that of single blood, with An. gambiae s.l. and An. funestus s.s. feeding on several hosts. It is becoming recurrent to find species of the An. gambiae complex and the An. funestus group with human and animal blood in Cameroon [39, 44] or in other tropical African countries [46, 47]. Finally, our results also showed an opportunistic behaviour of these species in terms of their propensity to feed on four different hosts (human, dog, pig and sheep). This multiple feeding could be attributed to the low nutritional content of the first meal ingested, defensive behaviour or host movement, and environmental factors such as wind movement [48].
Anopheles species from both sites were aggressive throughout the night both indoors and outdoors, with biting activity noticeably starting early in the evening (6p.m) and ending late in the morning, up to 8a.m in the specific case of An. funestus s.l. This early and late biting activities of Anopheles mosquitoes which is consistent with human behaviour, correlates with findings from different parts of the country [32, 49] and other tropical African countries [49,50,51] and may be due to the use of insecticide-treated bednets, which may induce mosquitoes to blood-feed at places and at times when humans are not protected. This result also suggests that studies are needed to investigate a possible daytime malaria as recently reported in Central African Republic (Bangui) where 20–30% of indoor bites occurred during the day and the prevalence of Plasmodium falciparum in night and day biting mosquitoes was the same [51]. The overall hourly activity of An. funestus s.l and An. gambiae s.l was significantly higher in the second part of the night (00–08 a.m) in both sites when people are supposed to be asleep. The Human Biting Rate (HBR) of An. funestus s.l. was significantly higher indoor than outdoor at both sites confirming its high endophagic tendency.
An. funestus sustains a very high entomological inoculation rate (EIR) in both locations
This study revealed a very high Plasmodium sporozoite infection rate across the two sites in An. funestus s.l mosquitoes. This infection rate was similar to some reports across Cameroon in the same mosquito species by Nkemngo et al. [26], Ndo et al. [52], Mieguim et al. [53] and was higher than recent reports across Africa by Osse et al. [35] in Benin, Mapua et al. [31] in Tanzania, Wolie et al. [54] in Côte D’Ivoire and Bamou et al. [49] in Kenya and in Ethiopia. The variations between these rates could be attributed to the differences in the detection methods used (TaqMan, ELISA and Nested-PCR). An. funestus s.l and An. gambiae s.l, were the only species found infected, confirming their key role as major malaria vectors and in Cameroon [17, 26]. Among Plasmodium species infecting the salivary glands of An. funestus s.l mosquitoes, P. falciparum was by far the predominant species counting for 92 and 70% of the global infection respectively in Elende and Mibellon. This result corroborates recent reports carried-out by Nkemngo et al. [26], Tchouakui et al. [29], Mbama-Ntabi et al. [55] (using human blood) and give right to the importance that malaria control strategies attributes against this species. The high proportion of P. malariae in Mibellon suggests that control programs should also pay attention to secondary parasite P. malariae and P. ovale in the prospect of malaria elimination efforts.
An. funestus populations from forested area (Elende) exhibited the same malaria transmission intensity than those from the humid savannah (Mibellon) with 66 ib/h/m (792 ib/p/year) on each site. This malaria transmission intensity represents one of the highest across the continent [4, 31, 35, 42, 49, 53] highlighting the high transmission of malaria in Cameroon, which is still part of the 11 countries with the highest burden in the world. This high implication of An. funestus s.l mosquitoes in malaria transmission corroborates with results obtained recently in Tanzania by Mapua et al. [31] and Kaindoa et al. [56] where An. funestus contributed for 97.7 and 86.26% of all malaria transmission respectively. This could be strongly correlated to the high affinity of An. funestus s.l mosquitoes to humans over other vertebrates as demonstrated previously by the very high HBI; their high resistance to pyrethroid insecticides used in LLINs which allow them to live much longer and become more infected [57,58,59], and their preference for permanent and semi-permanent aquatic habitats which last far longer than the rainy season [31]. Hence, our results suggest that persistent residual transmission is strongly associated with An. funestus s.l implying that targeted, high-impact, species-specific interventions could improve control of this vector. This further shows that the residual malaria transmission is not only related to zoophilic “secondary” vectors [51, 60], but often involves the “usual vector suspects” exhibiting opportunistic feeding and resting behaviors [61]. This opportunistic feeding and resting behaviors of ‘’usual vector suspects’’ is further confirmed by the very high malaria transmission intensity observed outdoor for An. funestus s.l mosquitoes (48 and 39 ib/h/m (576 and 468ib/h/y) in Mibellon and Elende respectively), supported by the fact that in Elende, transmission intensity was significantly equal indoor and outdoor. In the context of malaria elimination in the coming years, this latter observation illustrates the imperative of designing externally based vector control interventions complementary to the in-house interventions (LLINs, IRS).
Non-uniform distribution of L119F-GSTe2 resistance allele and high biting pattern of 119 F/F resistant An. funestus s.s at dawn
The L119F mutation was detected in both localities with a significantly higher frequency of the resistant allele in Elende (56%). The frequency of the resistant allele observed in Mibellon (35%) is almost similar to those obtained at the same site 3–4 years ago by Ndo et al. [62] (29.95%) and Tchouakui et al. [29] (26.3%), the slight differences observed being attributed to the study protocol used, the latter authors not having conducted longitudinal surveys and used only indoor resting female mosquitoes. This difference in terms of 119 F resistance allele frequency at both sites could be attributed to different insecticides selection pressures.
The biting pattern of 119 F/F-RR homozygous resistant mosquitoes showed that the 04–05 a.m. time slot represents their prime activity range at both sites. The result is quite similar with the one of Githinji et al. (2020) [63] in the western Kenya which showed that the 03–04 a.m was the prime activity range of kdr resistant An. gambiae mosquitoes. Together with a high proportion of mosquitoes carrying this genotype biting after 6 a.m. compared to other genotypes, these observations imply a high activity of 119 F/F resistant mosquitoes at dawn, especially when people are likely to be awake and out of their chemical or physical lethal barrier (LLIN). This result may be explained by the fact that the increased expression of GSTe2 in these mosquitoes helps them to avoid exposure at least partially to insecticide-treated nets during the hours when people are asleep and protected. This observation is in accordance with Menze et al. [57] during an experimental hut evaluation of treated nets against An. funestus s.s, where they showed that mosquitoes carrying the L119F-GSTe2 mutation were significantly associated with increased exophily to Olyset Plus and PermaNet 2.0 nets. This change in the biting pattern of 119 F/F resistant An. funestus s.s mosquitoes, with a heavy biting at dawn, could also explain residual malaria transmission.
The L119F-GSTe2 metabolic gene is associated with the high malaria transmission intensity
This assessment of the impact of L119F-GSTe2 metabolic gene on the malaria transmission intensity in Africa revealed that the L119F-GSTe2 mutation was strongly associated with increased EIR mainly by increasing Plasmodium infection rate in Elende. In fact, mosquitoes bearing 119 F-GSTe2 resistant allele (RR and RS) were significantly more likely to transmit malaria compared to their susceptible counterparts (SS). Similar observation showing that the L119F-GSTe2 metabolic gene increases An. funestus vector competence was reported in the central part of the country by Tchouakui et al. (2019) [14] and Ndo et al. (2019) [62] and could have four main explanations. Firstly, the resistance status conferred by the L119F-GSTe2 gene could explain this phenomenon, as mosquitoes carrying the resistant 119 F-GSTe2 allele live longer due to their ability to tolerate the effects of insecticide exposure in the field, and subsequently being more likely to enable parasites to complete their extrinsic incubation period than homozygous susceptible mosquitoes [29]. The second explanation is that, the increased susceptibility of mosquitoes to infection may be related to the overproduction of GSTs that could protect the parasites from the effects of reactive oxygen species (ROS) known to play a role in the innate immune responses of insects as a potent pathogen-killing agent [64]. The third is that resource-based trade-offs could have affected mosquito immune-competency in such a way that, overproduction of detoxifying enzymes such as GSTs is likely to deplete the resource pool, limiting the vector’s ability to mount a strong immune response, therefore favouring the development of the parasite [64]. The fourth is related to the change of the biting pattern of L119F-GSTe2 resistant An. funestus s.s mosquitoes as mentioned above, leading to an increased biting at times when peoples are likely to be awake and out of their chemical or physical lethal barrier (LLINs) as compared to the susceptible mosquitoes. The fact that L119F- GSTe2 resistance marker increases malaria transmission intensity is of great concern for the epidemiology of malaria in sub-Saharan Africa and in Cameroon in particular, further explaining the high epidemiological role of An. funestus s.s and the priority to also design species-specific interventions. This result is in agreement with the fact that the continuous increase of insecticide resistance under field conditions has a serious impact on malaria transmission, in particular, when this resistance is mediated by resistance markers like L119F-GSTe2 known to affect vector longevity [65, 66]. Owing to the fact that mosquitoes sometimes wear more than one insecticide resistance markers, additional work need to be done to further assess the combined effect of insecticide resistance marker on EIR across the continent.
Conclusion
This study reveals a predominance of An. funestus s.l. sustaining a high and perennial malaria transmission intensity both indoor and outdoor in two locations in Cameroon. Furthermore, the L119F-GSTe2 metabolic gene, one of the main resistance markers in An. funestus s.s., was significantly associated with an increase of malaria transmission intensity in this species. This shows that the GST-based metabolic resistance potentially increases malaria transmission in resistant An. funestus mosquitoes which could lead to an exacerbation of malaria transmission in areas with high GSTe2-based metabolic resistance to insecticides.
Data availability
The datasets generated and/or analysed during the current study are presented in the manuscript and in Additional file 1 openly available in figshare at https://doi.org/10.6084/m9.figshare.23297975.
Abbreviations
- PCR:
-
Polymerase Chain Reaction
- AS-PCR:
-
Allele Specific PCR
- ELISA:
-
Enzyme-Linked Immuno-sorbent Assay
- HLC:
-
Human Landing Catch
- CDC-LT:
-
Centre for Diseases Control Light Trap
- PSC:
-
Pyrethrum Spray Catch
- MHBR:
-
Monthly Human Biting Rate
- SIR:
-
Sporozoite Infection Rate
- EIR:
-
Entomological Inoculation Rate
- ib/h:
-
infected bites / human
- HBI:
-
Human Blood Index
- LLINs:
-
Long Lasting Insecticide Nets
- IR:
-
Insecticide Resistance
- GSTe2 :
-
Glutathione S-Transferase epsilon 2
- CYP450 :
-
Cytochrome P450
- RR:
-
Homozygous Resistant
- RS:
-
Heterozygous
- SS:
-
Homozygous Susceptible
References
NMCP., National Strategic Plan (2019–2023). 2020.
WHO. World Malaria report 2020: 20 years of global progress and challenges. World Health Organization Geneva; 2020. pp. 1–151.
Tabue RN et al. Case definitions of clinical malaria in children from three health districts in the north region of Cameroon BioMed Research International, 2019. 2019.
Antonio-Nkondjio C, et al. Review of Malaria situation in Cameroon: technical viewpoint on challenges and prospects for Disease elimination. Parasites & Vectors. 2019;12(1):1–23.
PNLP, Plan Strategique nationale de lutte contre le paludisme 2011–2015. Rapport Minsante Cameroun. 2012: p. 1–82.
Spillings BL, et al. The effect of a single blood meal on the phenotypic expression of insecticide resistance in the major Malaria vector Anopheles Funestus. Malar J. 2008;7(1):1–9.
Killeen GF. Characterizing, controlling and eliminating residual Malaria transmission. Malar J. 2014;13(1):1–22.
Sokhna C, Ndiath M, Rogier C. The changes in mosquito vector behaviour and the emerging resistance to insecticides will challenge the decline of Malaria. Clin Microbiol Infect. 2013;19(10):902–7.
Organization WH. High burden to high impact: a targeted Malaria response. World Health Organization; 2018.
Organization WH. Global plan for insecticide resistance management in Malaria vectors. World Health Organization; 2012.
Hemingway J, et al. The molecular basis of insecticide resistance in mosquitoes. Insect Biochem Mol Biol. 2004;34(7):653–65.
Ranson H, et al. Identification of a point mutation in the voltage-gated sodium channel gene of Kenyan Anopheles gambiae associated with resistance to DDT and pyrethroids. Insect Mol Biol. 2000;9(5):491–7.
Irving H, Wondji CS. Investigating knockdown resistance (kdr) mechanism against pyrethroids/DDT in the Malaria vector Anopheles Funestus across Africa. BMC Genet. 2017;18(1):1–11.
Tchouakui M, et al. Investigation of the influence of a glutathione S-transferase metabolic resistance to pyrethroids/DDT on mating competitiveness in males of the African Malaria vector, Anopheles Funestus. Wellcome open research; 2019. p. 4.
Riveron JM, et al. A single mutation in the GSTe2 gene allows tracking of metabolically based insecticide resistance in a major Malaria vector. Genome Biol. 2014;15(2):1–20.
Govella NJ, Chaki PP, Killeen GF. Entomological surveillance of behavioural resilience and resistance in residual Malaria vector populations. Malar J. 2013;12(1):1–9.
Nkemngo FN, et al. Elevated Plasmodium sporozoite Infection and multiple insecticide resistance in the principal Malaria vectors Anopheles Funestus and Anopheles gambiae in a forested locality close to the Yaoundé airport, Cameroon. Wellcome Open Research; 2020. p. 5.
Menze BD, et al. Bionomics and insecticides resistance profiling of Malaria vectors at a selected site for experimental hut trials in central Cameroon. Malar J. 2018;17(1):1–10.
Gillies MT, Coetzee M. A supplement to the Anophelinae of Africa South of the Sahara. Publ S Afr Inst Med Res. 1987;55:1–143.
Livak KJ. Organization and mapping of a sequence on the Drosophila melanogaster X and Y chromosomes that is transcribed during spermatogenesis. Genetics. 1984;107(4):611–34.
Koekemoer L, et al. A cocktail polymerase chain reaction assay to identify members of the Anopheles funestus (Diptera: Culicidae) group. Am J Trop Med Hyg. 2002;66(6):804–11.
Santolamazza F, et al. Insertion polymorphisms of SINE200 retrotransposons within speciation islands of Anopheles gambiae molecular forms. Malar J. 2008;7(1):1–10.
Kengne P, et al. Molecular identification of the Anopheles Nili group of African Malaria vectors. Med Vet Entomol. 2003;17(1):67–74.
Bass C, et al. PCR-based detection of Plasmodium in Anopheles mosquitoes: a comparison of a new high-throughput assay with existing methods. Malar J. 2008;7(1):1–9.
Snounou G, et al. High sensitivity of detection of human Malaria parasites by the use of nested polymerase chain reaction. Mol Biochem Parasitol. 1993;61(2):315–20.
Nkemngo FN, et al. Xeno-monitoring of molecular drivers of artemisinin and partner drug resistance in P. falciparum populations in Malaria vectors across Cameroon. Gene. 2022;821:146339.
Tchouakui M, et al. Fitness costs of the glutathione S-transferase epsilon 2 (L119F-GSTe2) mediated metabolic resistance to insecticides in the major African Malaria vector Anopheles Funestus. Genes. 2018;9(12):645.
Beier JC, et al. Bloodmeal identification by direct enzyme-linked immunosorbent assay (ELISA), tested on Anopheles (Diptera: Culicidae) in Kenya. J Med Entomol. 1988;25(1):9–16.
Tchouakui M, et al. A marker of glutathione S-transferase-mediated resistance to insecticides is associated with higher Plasmodium Infection in the African Malaria vector Anopheles Funestus. Sci Rep. 2019;9(1):1–12.
Kinya F, et al. Outdoor Malaria vector species profile in dryland ecosystems of Kenya. Sci Rep. 2022;12(1):1–10.
Mapua SA, et al. Persistently high proportions of plasmodium-infected Anopheles Funestus mosquitoes in two villages in the Kilombero valley, South-Eastern Tanzania. Parasite Epidemiol Control. 2022;18:e00264.
VectorLink-Cameroon P. The President’s Malaria Initiative (PMI)/VectorLink Project. The PMI VectorLink Project Cameroon Annual Entomology Report (October 2019-September 2020). Rockville, MD. The PMI VectorLink Project, Abt Associates. 2022, USAID. p. 57.
Wondji C, et al. Species and populations of the Anopheles gambiae complex in Cameroon with special emphasis on chromosomal and molecular forms of Anopheles gambiae Ss. J Med Entomol. 2005;42(6):998–1005.
FONDJO E et al. High Vector Diversity and Malaria Transmission Dynamics in Five Sentinel Sites in Cameroon 2022.
Ossè RA, et al. Involvement of Anopheles Nili in Plasmodium Falciparum transmission in North Benin. Malar J. 2019;18(1):1–10.
Adja AM, et al. Contribution of Anopheles Funestus, an. Gambiae and An. Nili (Diptera: Culicidae) to the perennial Malaria transmission in the southern and western forest areas of Côte d’Ivoire. Ann Trop Med Parasitol. 2011;105(1):13–24.
Dia I, et al. Bionomics of Anopheles gambiae Giles, an. Arabiensis Patton, an. Funestus Giles and An. Nili (Theobald)(Diptera: Culicidae) and transmission of Plasmodium Falciparum in a sudano-guinean zone (Ngari, Senegal). J Med Entomol. 2003;40(3):279–83.
Bigoga JD, et al. Malaria vectors and transmission dynamics in coastal south-western Cameroon. Malar J. 2007;6(1):1–12.
Tabue RN, et al. Role of Anopheles (Cellia) rufipes (Gough, 1910) and other local anophelines in human Malaria transmission in the northern savannah of Cameroon: a cross-sectional survey. Parasites & Vectors. 2017;10(1):1–11.
Tepa A, et al. Molecular drivers of multiple and Elevated Resistance to insecticides in a Population of the Malaria Vector Anopheles gambiae in Agriculture Hotspot of West Cameroon. Genes. 2022;13(7):1206.
Antonio-Nkondjio C, et al. High Malaria transmission intensity in a village close to Yaounde, the capital city of Cameroon. J Med Entomol. 2002;39(2):350–5.
Tanga M, Ngundu W, Tchouassi P. Daily survival and human blood index of major Malaria vectors associated with oil palm cultivation in Cameroon and their role in Malaria transmission. Tropical Med Int Health. 2011;16(4):447–57.
Awono-Ambene PH, et al. The bionomics of the Malaria vector Anopheles rufipes Gough, 1910 and its susceptibility to deltamethrin insecticide in North Cameroon. Volume 11. Parasites & vectors; 2018. pp. 1–11. 1.
Ekoko WE, et al. Patterns of anopheline feeding/resting behaviour and Plasmodium Infections in North Cameroon, 2011–2014: implications for Malaria control. Parasites & Vectors. 2019;12(1):1–12.
Njoroge MM, et al. Exploring the potential of using cattle for Malaria vector surveillance and control: a pilot study in western Kenya. Parasites & Vectors. 2017;10(1):1–16.
Adugna T, Yewhelew D, Getu E. Bloodmeal sources and feeding behavior of anopheline mosquitoes in Bure district, northwestern Ethiopia. Volume 14. Parasites & Vectors; 2021. pp. 1–12. 1.
Massebo F, et al. Blood meal origins and insecticide susceptibility of Anopheles arabiensis from Chano in South-West Ethiopia. Volume 6. Parasites & vectors; 2013. pp. 1–10. 1.
Jeyaprakasam NK, et al. Blood meal analysis of Anopheles vectors of simian Malaria based on laboratory and field studies. Sci Rep. 2022;12(1):1–13.
Bamou R, et al. Entomological and anthropological factors contributing to persistent Malaria transmission in Kenya, Ethiopia, and Cameroon. J Infect Dis. 2021;223(Supplement2):S155–70.
Keïta M, et al. Indoor and outdoor Malaria transmission in two ecological settings in rural Mali: implications for vector control. Malar J. 2021;20(1):1–11.
Sangbakembi-Ngounou C, et al. Diurnal biting of Malaria mosquitoes in the Central African Republic indicates residual transmission may be “out of control. Proc Natl Acad Sci. 2022;119(21):e2104282119.
Ndo C, et al. Elevated Plasmodium Infection rates and high pyrethroid resistance in major Malaria vectors in a forested area of Cameroon highlight challenges of Malaria control. Parasites & Vectors. 2018;11(1):1–7.
Mieguim Ngninpogni D, et al. Insights into factors sustaining persistence of high Malaria transmission in forested areas of sub-saharan Africa: the case of Mvoua, South Cameroon. Volume 14. Parasites & Vectors; 2021. pp. 1–10. 1.
Wolie RZ, et al. Entomological indicators of Malaria transmission prior to a cluster-randomized controlled trial of a ‘lethal house lure’intervention in central Côte d’Ivoire. Malar J. 2022;21(1):1–11.
Mbama Ntabi JD, et al. Prevalence of non-plasmodium falciparum species in southern districts of Brazzaville in the Republic of the Congo. Volume 15. Parasites & vectors; 2022. pp. 1–13. 1.
Kaindoa EW, et al. Interventions that effectively target Anopheles Funestus mosquitoes could significantly improve control of persistent Malaria transmission in south–eastern Tanzania. PLoS ONE. 2017;12(5):e0177807.
Menze BD, et al. An experimental hut evaluation of PBO-based and pyrethroid-only nets against the Malaria vector Anopheles Funestus reveals a loss of bed nets efficacy associated with GSTe2 metabolic resistance. Genes. 2020;11(2):143.
Tchouakui M, et al. Pyrethroid resistance aggravation in Ugandan Malaria vectors is reducing bednet efficacy. Pathogens. 2021;10(4):415.
Kumala J et al. Intensity of Insecticide Resistance in the Major Malaria Vector Anopheles Funestus From Chikwawa, Rural Southern Malawi 2022.
Degefa T, et al. Patterns of human exposure to early evening and outdoor biting mosquitoes and residual Malaria transmission in Ethiopia. Acta Trop. 2021;216:105837.
Wagman J, Fornadel C, Okumu F. Some residual Malaria transmission may be out of control but within reach of current tools. Proc Natl Acad Sci U S A. 2022;119(38):e2210568119.
Ndo C et al. Exploring the impact of glutathione S-transferase (GST)-based metabolic resistance to insecticide on vector competence of Anopheles Funestus for Plasmodium Falciparum. Wellcome Open Research, 2019. 4.
Githinji EK et al. Impact of insecticide resistance on P. falciparum vectors’ biting, feeding, and resting behaviour in selected clusters in Teso North and South Subcounties in Busia County, western Kenya Journal of parasitology research, 2020. 2020.
Rivero A, et al. Insecticide control of vector-borne Diseases: when is insecticide resistance a problem? PLoS Pathog. 2010;6(8):e1001000.
Ngongang-Yipmo ES, et al. Reduced performance of community bednets against pyrethroid-resistant Anopheles funestus and Anopheles gambiae, major Malaria vectors in Cameroon. Volume 15. Parasites & vectors; 2022. pp. 1–15. 1.
Tchakounte A, et al. Exposure to the insecticide-treated bednet PermaNet 2.0 reduces the longevity of the wild African Malaria vector Anopheles Funestus but GSTe2-resistant mosquitoes live longer. PLoS ONE. 2019;14(3):e0213949.
Acknowledgements
Authors are grateful to the research team members at CRID particularly Herve R. Tazokong, Mahamat Gadji and Riccado Thiomela for their assistance during mosquitoes sampling in Mibellon; Micareme Tchoupo, Nafissatou Monde and Ines Watsop for their assistance in laboratory work. We also thank the community leaders and members of Elende and Mibellon villages for allowing access to their homes for mosquito sampling purposes.
Funding
This work was supported by a Wellcome Trust Senior Research Fellowships in Biomedical Sciences (217188/Z/19/Z to C.S.W.) and Bill and Melinda Gates foundation Investment (ID INV-006003 to C.S.W.)
Author information
Authors and Affiliations
Contributions
C.S.W. conceived and designed the study; J.T. and L.N provided scientific supervision. B.N.S.F, M.T, D.F, B.D.M, L.M.J.M., and D.N.N collected the samples on the field; B.N.S.F, D.F,. performed the molecular experiments; B.N.S.F, M.T. and C.S.W analyzed the data; B.N.S.F, M.T., B.D.M, L.M.J.M and C.S.W. wrote the manuscript with contribution from all the authors. All authors have read and agreed to the published version of the manuscript.
Corresponding authors
Ethics declarations
Ethics approval and consent to participate
This work was reviewed and approved by the National Ethics Committee for Health Research (CNERSH) of Cameroon (ID: 2021/07/1372/CE/CNERSH/SP). Written, informed and signed consent was obtained from sleepers before starting the survey. The consent form provided all the information and the evaluation process about the study. Information was translated in local language when needed. All methods were carried out in accordance with relevant guidelines and regulations outlined in the Declaration of Helsinki.
Consent for publication
Not applicable.
Competing interests
The authors declare no competing interests.
Additional information
Publisher’s Note
Springer Nature remains neutral with regard to jurisdictional claims in published maps and institutional affiliations.
Electronic supplementary material
Below is the link to the electronic supplementary material.
Rights and permissions
Open Access This article is licensed under a Creative Commons Attribution 4.0 International License, which permits use, sharing, adaptation, distribution and reproduction in any medium or format, as long as you give appropriate credit to the original author(s) and the source, provide a link to the Creative Commons licence, and indicate if changes were made. The images or other third party material in this article are included in the article’s Creative Commons licence, unless indicated otherwise in a credit line to the material. If material is not included in the article’s Creative Commons licence and your intended use is not permitted by statutory regulation or exceeds the permitted use, you will need to obtain permission directly from the copyright holder. To view a copy of this licence, visit http://creativecommons.org/licenses/by/4.0/. The Creative Commons Public Domain Dedication waiver (http://creativecommons.org/publicdomain/zero/1.0/) applies to the data made available in this article, unless otherwise stated in a credit line to the data.
About this article
Cite this article
Natchema S. Fonkou, B., Tchouakui, M., Menze, B.D. et al. Entomological longitudinal surveys in two contrasted eco-climatic settings in Cameroon reveal a high malaria transmission from Anopheles funestus associated with GSTe2 metabolic resistance. BMC Infect Dis 23, 738 (2023). https://doi.org/10.1186/s12879-023-08698-8
Received:
Accepted:
Published:
DOI: https://doi.org/10.1186/s12879-023-08698-8