Abstract
Background
Late Embryogenesis Abundant (LEA) proteins are a class of proteins associated with plant stress resistance. Two Juglans species, Juglans regia and J. mandshurica, are both diploid (2n = 32), monoecious perennial economic tree species with high edible, pharmaceutical, and timber value. The identification, characterization, and expression patterns of LEA proteins in J. regia and its wild relative, J. mandshurica, would not only provide the genetic basis of this gene family, but it would also supply clues for further studies of the evolution and regulating mechanisms of LEA proteins in other tree species.
Results
In this study, we identified 25 and 20 members of the LEA gene family in Juglans regia and its wild relative, Juglans mandshurica, respectively. The results of phylogenetic analysis showed that the LEA members were divided into eight main subgroups. Predictions of their physicochemical properties showed the variable characteristics of LEA proteins, and the subcellular localization analysis indicated that most LEA proteins are localized in the nucleus. Chromosomal localization analysis and gene replication pattern prediction indicated that WGD is the predominant duplication mode of LEA genes. The results of the comparative analysis indicated a high level of collinearity between the two Juglans species. Analysis of cis-acting elements indicated that LEA genes had a relatively wide range of responses to abiotic stresses and phytohormonal processes, particularly in two phytohormones, methyl jasmonate and abscisic acid. Transcriptome profiling and qRT-PCR experiments showed that JrLEAs are commonly expressed in leaves, green husks, and male and female flowers, and most JmLEAs are more highly expressed in male flowers. We also hypothesized that JrLEAs are involved in the process of anthracnose resistance. Anthracnose-resistant varieties of JrLEAs presented relatively high expression levels at later stages.
Conclusion
In this study, we provide a theoretical basis for the functional study of LEA genes in J. regia and J. mandshurica. Analysis of cis-acting elements and gene expression indicated that JrLEAs and JmLEAs play important roles in resistance to biotic stresses in these species.
Similar content being viewed by others
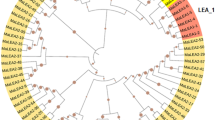
Background
Plants have unique molecular response mechanisms and physiological reactions that mitigate the effects of external stresses upon themselves when negatively affected by the external environment, a process known as plant resilience [1]. External stresses usually include abiotic and biotic stresses, such as drought [2], temperature extremes [3, 4], land salinization [5], UV irradiation [6], pathogenic bacteria, and harmful insects [7]. Water stress is one of the most common environmental stresses that plants suffer.
As osmoprotective and desiccation damage repair agents, Late Embryogenesis Abundant (LEA) proteins, existing widely in the plant kingdom, are a class of dehydration-resistance-associated glycine-rich proteins with low molecular weights (10–30 kDa) [8]. LEA proteins accumulate in seeds with large quantities at later stages of plant embryogenesis development, responding to a variety of abiotic stresses and protecting higher plants from extreme environmental stresses, especially playing a non-negligible role in resisting drought stress. Although the debate is still ongoing regarding the classification of LEA proteins among various species [9], they can be generally classified into the following eight groups according to their conserved structural domains, as follows: LEA_1, LEA_2, LEA_3, LEA_4, LEA_5, LEA_6, Dehydrin, and Seed Maturation Protein (SMP) [10]. The study of the regulatory mechanisms of LEA gene expression is of great importance for modern plant molecular biology. LEA proteins are highly tolerant to dry environments in cotton [11]. Subsequently, more and more studies [8] had shown that LEAs play a key role in the tolerance response to drought stress. The overexpression of OsLEA can increase the sensitivity and osmotic tolerance of rice to abscisic acid under drought stress [12]. Similarly, the overexpression of CaLEA1 enhances stomatal sealing and the expression of the related downstream genes in response to drought and salt stress [13]. Furthermore, AtLEA14 [14] and SiLEA14 [15] genes have been shown to be salt-tolerant. Recently, TaLEA [16], ZmLEA3 [17], and SmLEA [18] have also been shown to be resistant to drought stress.
The Persian (English) walnut (Juglans regia L., 2n = 32), also known as the common walnut, belonging to the genus Juglans in the family Juglandaceae and the monoecious perennial tree [19], is the second most valuable nut crop in the world, being surpassed only by almonds (Prunus dulcis) [20]. In China, J. regia is mainly grown and cultivated in the provinces of Xinjiang, Shaanxi, Yunnan, and Hebei [21]. Walnuts have important edible and medicinal value, owing to their dense canopies and leafy branches, their kernel fat content of 65–83%, their protein content of more than 15%, and a variety of essential trace elements. Juglans mandshurica (2n = 32), a closely related wild species of the Persian walnut, is an ecologically important, wind-pollinated, and endemic species that grows in northern and northeastern China, Korea, Japan, and the far eastern section of Russia [22,23,24,25]. The LEA gene family is important for plant growth and developmental processes, and so far, LEA genes have been identified in many plants, such as Arabidopsis [14], poplar [26], cucumber [27], and oilseed rape [28]. However, the identification, distribution, and characterization of LEA gene family members in Juglans, as well as their expression patterns, are still unknown. Hence, Juglans, identifying and characterizing LEA gene family members across the genomes of the two Juglans species and conducting a comprehensive comparative study are necessary.
In the present study, we identified and analyzed the LEA gene family for the first time in J. regia and its wild relative, J. mandshurica. We determined their phylogenetic relationships, predicted their physicochemical properties, identified their conserved structural domains, investigated their chromosomal localization and gene duplication patterns, and performed gene structure analysis and cis-acting element analysis for LEA gene members in the J. regia and J. mandshurica genomes. Additionally, we produced LEA gene expression profiles using transcriptome sequencing and qRT-PCR based on multi-tissue organs and biotic stress treatment conditions. The results not only provide a fundamental basis for studying LEA gene function in J. regia and J. mandshurica, but they also provide clues for further studies of the evolution and regulating mechanisms of LEA proteins in other tree species.
Materials and methods
Identification of LEA gene members
To identify the candidate members of the LEA gene family in two Juglans species, 51 LEA protein sequences of Arabidopsis thaliana (https://www.arabidopsis.org/) were adopted as query sequences to perform the genome-wide BLASTP in the J. regia and J. mandshurica [29]. Subsequently, the protein structural domains were analyzed using the CDD (https://www.ncbi.nlm.nih.gov/Structure/bwrpsb/bwrpsb.cgi), Pfam (http://pfam.xfam.org/), and SMART (http:/ /smart.embl.de/ /smart.embl.de/) databases, respectively. The candidate genes which contained any of the LEA_1, LEA_2, LEA_3, LEA_4, LEA_5, LEA_6, SMP, or Dehydrin structural domains were considered to be members of LEA gene family. Afterward, the identified JrLEAs and JmLEAs were submitted to the online website of GSDs (http://gsds.gao-lab.org/) for gene structure analysis and visualized using TBTOOLS software [30].
Prediction of physicochemical properties and subcellular localization
Physicochemical properties of all identified LEA proteins in J. regia and J. mandshurica were predicted using the ExPASy online tools (http://www.expasy.org/tools/protparam.html). Subcellular localization of all identified LEA genes in J. regia and J. mandshurica were predicted using the WoLF PSORT online tools (https://wolfpsort.hgc.jp/).
Characteristics and comparative analysis of the identified LEA genes
Chromosomal localization of all identified LEA genes in J. regia and J. mandshurica were performed using TBTOOLS software according to the gene annotation [30]. For subsequent investigation, candidate genes were renamed corresponding to their position on the chromosomes. Gene duplication events and collinearity analysis were predicted for JrLEAs and JmLEAs using MCScanX software [31]. Moreover, the KAKS_CALCULATOR 2.0 software [32] was utilized to calculate Ka/Ks values to judge the selective pressure among the identified gene pairs. Furthermore, cis-acting element prediction was executed by using 2000 bp sequences upstream of the identified LEA genes via PlantCARE (https://bioinformatics.psb.ugent.be/webtools/plantcare/html/).
Phylogenetic analysis
The maximum likelihood phylogenetic tree of the LEA members in Arabidopsis thaliana, J. regia, and J. mandshurica was constructed by using the IQTREE software [33], in which the VT + F + G4 was adopted as the best-fit substitution model according to the BIC score with 1000 times ultra-fast bootstraps. The phylogenetic trees were visualized using the iTOL online website (https://itol.embl.de/login.cgi).
Protein-protein interactions and microRNA targeting analysis
Uploading LEA protein sequences of two Juglans species to the STRING online website (https://cn.string-db.org) to predict protein-protein interactions. All genomic sequences of identified LEA members were submitted as candidates to predict of potential miRNAs, using the default parameters of psRNATarget online website (https://www.zhaolab.org/psRNATarget/) [34]. Visualization using Cytoscape software default parameters [35].
Transcriptome profile analysis and qRT-PCR verification
For the transcriptome analysis, multi-tissue gene expression data were obtained from our previously determined transcriptome data [36, 37]. Meanwhile, the walnut disease resistance gene expression data were obtained from the public Sequence Read Archive database (https://www.ncbi.nlm.nih.gov/geo/query/acc.cgi?acc=GSE147083) [38], in which the F26 indicated anthracnose-resistant varieties, while F423 indicated anthracnose-susceptible varieties. Firstly, the raw data were filtered by using the FASTAP software [39], after which the clean reads were mapped to the reference genome of J. regia using HISAT2 software [40]. Finally, the expression of genes was calculated using FEATURECOUNTS [41].
Subsequently, the qRT-PCR were implemented to furtherly explore the expression pattern of the identified LEA genes. The leaves, green husks, male and female flowers of the two species in the same developmental period (maturity) were selected for the qRT-PCR verification. A total of 3 biological replicates were present per species/tissue organ. This study was approved by the Chinese government and carried out according to the laws of the People’s Republic of China. All participants had a license approval letter from the College of Life Sciences, Northwest University. Both male and female flowers were harvested in mid-April from the Zhuque National Forest Park (ZNFP) in Xi’an, Shaanxi Province (108°E, 33°N, Altitude 1340.8 m), while the leaves and green husks were harvested from ZNFP in late August. All samples were immediately frozen in liquid nitrogen after collection for further utilization. In this study, Prof. Peng Zhao identified individual J. mandshurica and J. regia trees according to the following botanical characteristics: leaves, buds, male flowers, female flowers, stems, and fruits. We obtained the permissions to collect those plant samples from ZNFP. The voucher specimen of J. regia and J. mandshurica (deposition accession numbers: NWU2020016 and NWU2020036) were stored at the Evolutionary Botany Laboratory, College of Life Sciences, Northwest University (Xi’an, Shaanxi, China).
Total RNA was extracted from each sample using an RNA extraction kit (plant RNA Kit (50) OMEGA, USA). Complementary DNA (cDNA) was synthesized using 5× PrimeScript RT Master Mix (Takara) reverse transcriptase. The above cDNA was diluted 5-fold as qRT-PCR template. Subsequently, qRT-PCR experiments were performed on a Bio-Rad CFX96 fluorescent quantitative PCR instrument using 2× Plus SYBR real-time PCR mixture (Biotec) as a fluorescent dye. Each sample had 3 biological replicates and 3 technical replicates. J. regia β-Actin was selected as the internal reference gene and primers were designed using the Primer3Plus online website (https://www.primer3plus.com). The primer design sequences are shown in Table S1. qRT-PCR results were analyzed by the 2-ΔΔCT method [42].
Results
Genome-wide identification and phylogenetic analysis of LEA genes in Juglans
A total of 25 LEA gene family members in J. regia and 20 LEA gene family members in J. mandshurica were present in the samples, and we renamed all members identified in two Juglans species according to their positions on the chromosomes. Details regarding the names, gene IDs, and protein sequences of all identified J. regia and J. mandshurica LEA members were shown in Table S2.
The maximum likelihood (ML) tree was reconstructed for the LEA members in J. regia and J. mandshurica. All members of the LEA family of Arabidopsis, J. regia and J. mandshurica could be divided into eight groups with high bootstraps value (=1000), as follows: LEA_1, LEA_2, LEA_3, LEA_4, LEA_5, LEA_6, SMP, and Dehydrin, each of which corresponded to a unique domain (Fig. 1). Therein, the largest subgroup was LEA_4, which contained 2 JrLEAs, 2 JmLEAs, and 18 AtLEAs, followed by the Dehydein subgroup, which contained 5 JrLEAs, 4 JmLEAs, and 19 AtLEAs. Both the LEA_2 and SMP groups had 13 LEA members, with the former containing 5 JrLEAs, 5 JmLEAs and 3 AtLEAs, and the latter included 3 JrLEAs, 4 JmLEAs, and 6 AtLEAs, respectively. The rest members of LEA were divided into other groups, in which 5 JrLEAs, 3 JmLEAs, and 4 AtLEAs were distributed in group LEA_3, 2 JrLEAs, 1 JmLEA, and 3 AtLEAs were distributed in group LEA_1, 2 JrLEAs, 1 JmLEA, and 2 AtLEAs were distributed in group LEA_5, and 1 JrLEA and 3 AtLEAs were distributed in group LEA_6. No mixture was present among each identified group, indicating that the LEA gene family members were relatively conserved within different groups of these three species.
Physicochemical properties and subcellular localization
The length of the LEA proteins in J. regia ranged from 88 amino acids (JrLEA5) to 534 amino acids (JrLEA4), with an average length of 186.28 amino acids (Table 1). LEA proteins in J. mandshurica were longer, ranging from 92 amino acids (JmLEA3) to 773 amino acids (JmLEA15), with an average length of 223.45 amino acids. The molecular weights of LEA proteins in J. regia ranged from 9659.57 Da (JrLEA5) to 59,259.16 Da (JrLEA4), with an average of 20,219.93 Da. Similarly, the molecular weights of JmLEAs were greater than those of J. regia, which ranged from 9815.01 Da (JmLEA3) to 59,504.39 Da (JmLEA19), with an average of 24,325.8545 Da. In addition, 17 and 13 LEA proteins in J. regia (JrLEA2, JrLEA3, JrLEA4, JrLEA5, JrLEA6, JrLEA7, JrLEA8, JrLEA11, JrLEA15, JrLEA16, JrLEA18, JrLEA20, JrLEA21, JrLEA22, JrLEA23, JrLEA24, and JrLEA25) and J. mandshurica (JmLEA1, JmLEA2, JmLEA3, JmLEA4, JmLEA5, JmLEA6, JmLEA7, JmLEA8, JmLEA11, JmLEA12, JmLEA17, JmLEA18, and JmLEA19) were acidic proteins (Isoelectric point < 7), respectively. A total of 11 and 7 LEA proteins were present in J. regia and J. mandshurica, respectively, with instability index values > 40. Almost all identified LEA proteins in J. regia and J. mandshurica had a positive Grand Average of Hydropathicity (GRAVY) with only five exceptions (JrLEA16, JrLEA20, JmLEA1, JmLEA5, and JmLEA6), which suggested that the majority of LEA proteins in J. reiga and J. mandshurica were hydrophobic. In addition, the identified LEA members were distributed in mitochondria, chloroplasts, the nucleus, the cytoskeleton, peroxisomes, and the extracellular matrix. Most of the identified LEA members were located in the nucleus (Table 1).
Chromosome location and duplication patterns
For J. regia, a total of 25 LEA genes were distributed on 10 different chromosomes (Fig. 2a). Chromosome 7 contained the greatest number of LEA genes (JrLEA5, JrLEA6, JrLEA7, JrLEA8, JrLEA9, and JrLEA10), followed by chromosome 8 (containing JrLEA11, JrLEA12, JrLEA13, JrLEA14, and JrLEA15). For J. mandshurica (Fig. 2b), chromosome 1 contained the greatest number of LEA genes (JmLEA1, JmLEA2, JmLEA3, JmLEA4, and JmLEA5). Chromosome 2 (JmLEA6, JmLEA7, JmLEA8, and JmLEA9) and chromosome 8 (JmLEA14, JmLEA15, JmLEA16, and JmLEA17) both possessed four LEA genes, and chromosome 7 had two LEA genes (JmLEA12 and JmLEA13). The remaining LEA genes were located in unique chromosomes.
Chromosomal distribution and duplication types of LEA genes. a Chromosomal localization and duplication types of the LEA genes in J. regia; b Chromosomal localization and duplication types of the LEA genes in J. mandshurica. The orange triangles, purple circles, blue circles, yellow circles, and green circles represent Singleton, Whole genome duplication (WGD), Tandem duplication (TD), Dispersed duplication (DSD), and Proximal duplication (PD), respectively
The results of gene duplication analysis showed four patterns of LEA gene duplication, of which Whole Genome Duplication (WGD) was the major mode in Juglans (Fig. 2; Table 2). A total of 14 out of 25 LEA genes (56%) in J. regia and 10 out of 20 LEA genes (50%) in J. mandshurica experienced WGD. Furthermore, the Tandem Duplication (TD) mode was found in JrLEA9, JrLEA10, JrLEA14, and JmLEA1. Four JrLEAs (JrLEA4, JrLEA15, JrLEA16, and JrLEA17) and five JmLEAs (JmLEA4, JmLEA10, JmLEA14, JmLEA19, and JmLEA20) underwent Dispersed Duplication (DSD). Notably, several LEA genes, identified as singleton genes, did not appear to show duplication, and the JrLEA13 and JmLEA19 were considered to have Proximal Duplication (PD).
Conserved structural domains and gene structure analysis
To precisely analyze the protein structural domains and gene structures of J. regia and J. mandshurica, we first constructed a phylogenetic tree of the two species using their LEA protein sequences (Fig. 3a). The reconstructed ML tree based on the two species presented similar topologies compared to those when plusing the LEA members of Arabidopsis (Fig. 1). The results show that LEA proteins contained highly conserved LEA protein structural domains and kinase structural domains (Fig. 3b). All members of LEA in J. regia and its wild relative, J. mandshurica, contained an LEA signature protein structural domain and a corresponding kinase structural domain. The LEA domain was usually located at the C-terminus of the LEA protein, and the kinase structural domain was usually located at the N-terminal. These results may be related to the functions of LEA proteins. However, the results of gene structure analysis (Fig. 3c) show that the LEA gene structures of J. regia and J. mandshurica were highly divergent. Notably, all LEA genes contained multiple exons except for JrLEA5 and JmLEA12, which contained only one exon. JmLEA15 contained 12 exons; JmLEA4 and JmLEA14 contained 6 exons; JrLEA17, JmLEA2, and JmLEA20 contained 4 exons; JrLEA4, JrLEA7, JrLEA8, JrLEA15, JrLEA20, JrLEA21, JrLEA22, JmLEA1, JmLEA10, JmLEA19, and JmLEA20 contained 3 exons; and the remaining LEA genes contained 2 exons. Some individual LEA genes had long introns, especially JmLEA15.
The gene structures and protein domains of LEA members. a Maximum likelihood phylogenetic tree of LEAs in two Juglans species; b Protein domains of LEAs in two Juglans species. Different domains were represented by different colored boxes; c Gene structures of LEAs in two Juglans species. Green boxes indicated exons, and gray lines indicate introns
Collinearity and selective pressure analysis
The collinearity predictions showed six and five LEA paralogous gene pairs in J. regia and J. mandshurica, respectively, and 24 LEA orthologous gene pairs between the two Juglans species (Fig. 4; Table S3). The number of identified orthologous gene pairs was greater than that of paralogous gene pairs, indicating a high degree of collinearity in LEA members between two Juglans species and indicating that most members of the LEA gene family likely existed in the ancestors of the two Juglans species rather than being formed separately after their divergence. Between the two Juglans species, seven JrLEAs (JrLEA1, JrLEA5, JrLEA9, JrLEA10, JrLEA13, JrLEA14, and JrLEA16) without collinearity and five JmLEAs (JmLEA4, JmLEA10, JmLEA11, JmLEA17, and JmLEA20) without collinearity were present. Therefore, these genes may be specific to J. regia and J. mandshurica. In addition, 23 orthologous gene pairs were present between Arabidopsis and J. regia, and 15 orthologous gene pairs were present between Arabidopsis and J. mandshurica (Table S4). In each of the two Juglans species, 10 genes without collinearity were related to AtLEAs.
To further explore the selection pressure of the homologous gene pairs of the two Juglans species, we subsequently analyzed the Ka/Ks values of these homologous gene pairs (Table S3). The Ka/Ks ratios of most LEA homologous gene pairs were less than 1, suggesting that these gene pairs were subject to purifying selection during evolution. The Ka/Ks value of more than 1 for an orthologous gene pair, JmLEA12 and JrLEA18, indicated that it experienced positive selection and might have a relatively fast evolutionary rate.
Analysis of Cis-acting elements in J. regia and J. mandshurica
To investigate the potential functions of LEA genes in J. regia and J. mandshurica, we analyzed cis-acting elements in their upstream promoter regions. We divided the cis-acting elements into four main categories, namely those that respond to plant development and growth, plant hormones, abiotic stresses, and light (Fig. 5). The promoter regions of most LEAs contained cis-acting elements associated with abiotic stress, suggesting that they may play an important role in abiotic stress resistance in the two Juglans species. In addition, the promoter regions of JrLEAs contained more cis-acting elements than those of JmLEAs, suggesting that LEAs in J. regia may be involved in more complex signaling and pathways than those of its wild relative. We also found that cis-acting elements in the promoter regions of the LEAs of two Juglans species contained a large number of elements associated with three phytohormone responses, namely the CGTCA and TGACG motifs (associated with the methyl jasmonate hormone response) and ABRE (associated with the abscisic acid hormone response). JmLEAs’ response to light involves more components than that of JrLEAs; therefore, J. mandshurica is presumably more sensitive to light.
Protein–protein interactions, microRNA targeting, and expression analysis of LEA genes in J. regia and its wild relative, J. mandshurica
A total of 19 JrLEAs, exclulding JrLEA9, JrLEA10, JrLEA13, JrLEA15, JrLEA17, and JrLEA25, interacted with at least one LEA protein in the two Juglans species. Among them, JrLEA1, JrLEA2, and JrLEA4 had more interactions with other LEA proteins. In contrast, only JmLEA3 interacted with other LEA proteins in J. mandshurica (Fig. S1).
We used transcriptome data from leaves, green husks, and male and female flowers to investigate the expression patterns of LEA genes in the two Juglans species (Fig. 6a, b, Tables S5 and S6). In addition, we randomly selected four JrLEAs and four JmLEAs and verified their relative expressions in leaves, green husks, and male and female flowers via qRT-PCR experiments, which were consistent with the transcriptome data (Fig. 7). Validating the results with transcriptome data combined with the qRT-PCR experiments revealed that JrLEA17 was not expressed in J. regia. Ten genes were highly expressed in J. regia leaves, eight genes were highly expressed in J. regia green husks, nine genes were highly expressed in J. regia male flowers, and nine genes were highly expressed in J. regia female flowers. However, only eight genes (JmLEA1, JmLEA2, JmLEA6, JmLEA8, JmLEA13, JmLEA16, JmLEA17, and JmLEA18) were expressed in four selected tissues of J. mandshurica. The majority of JmLEA genes (7 out of 8) were highly expressed in male flowers. Only one gene, namely JmLEA1, was highly expressed in leaves, and JmLEA17 presented a high expression level in green husks. All of those without collinear JmLEAs (unique) were not expressed in female and male flowers, leaves, or green husks. For those without collinear JrLEAs (seven in total), JrLEA13 and JrLEA16 were expressed at high levels in male and female flowers, JrLEA9 was expressed at high levels in leaves, and the other four genes (JrLEA1, JrLEA10, JrLEA5, and JrLEA14) were all expressed at low levels in the four selected organs (Fig. S2).
Expression patterns of LEAs in J. regia and J. mandshurica. a Expression patterns of identified LEAs in female flowers, male flowers, leaves and green husks of J. regia; RF, female flowers of J. regia; RM, male flowers of J. regia; RL, leaves of J. regia; RG, green husks of J. regia; b Expression patterns of identified LEAs in female flowers, male flowers, leaves and green husks of J. mandshurica; MF, female flowers of J. mandshurica; MM, male flowers of J. mandshurica; ML, leaves of J. mandshurica; MG, green husks of J. mandshurica; c Expression patterns of identified LEAs in J. regia under biotic stress. F26 indicated anthracnose-resistant varieties, F423 indicated anthracnose-susceptible varieties. The colored scale reflects gene expression levels
Relative expression of JrLEAs and JmLEAs in leaves, green husk, male flowers and female flowers. The yellow bars represent data from qRT-PCR experiments, and the blue lines represent transcriptome data. The statistical significance using student’s t-test. ns = no significant different, * = p < 0.05, ** = p < 0.01, *** = p < 0.001
No microRNAs targeted JrLEA2, JrLEA6, JrLEA9, JrLEA10, JrLEA24, JmLEA1, JmLEA2, JmLEA4, JmLEA5, JmLEA7 or JmLEA18. ath-miR414 targeted the most LEA genes (including JrLEA4, JrLEA8, JrLEA12, JrLEA13, JrLEA22, JmLEA16, and JmLEA19; Fig. S1, Table S7). In addition, ath-miR414 targeted seven LEA genes except for JmLEA19, which was not expressed in the four selected organs, and JrLEA8 was highly expressed in green husks. The remaining five genes were all highly expressed in female flowers (Fig. 6).
To further investigate the role of JrLEAs in response to biotic stress, we analyzed the gene expression patterns of different varieties of J. regia under biotic stress. The results showed that JrLEAs were expressed at higher levels in anthracnose-resistant varieties (F26) than in anthracnose-susceptible varieties (F423) after infection (Fig. 6c; Table S8). This indicates that JrLEAs might play a role in anthracnose resistance in J. regia. Most JrLEAs (72%) in anthracnose-susceptible varieties (F423, 48 hours). reached peak expression at 48 h of stress. JrLEAs presented a relatively high expression level at the late stage in anthracnose-resistant varieties (F26, 120 hours). Of the seven unique JrLEAs, JrLEA1, JrLEA5, JrLEA14, and JrLEA16 had higher expression levels at 48 hours of infection in variety F423. JrLEA1 and JrLEA5 had higher expression levels at 120 hours of infection in variety F26. The other three genes (JrLEA9, JrLEA10, and JrLEA13) showed low expression levels in both F26 and F423 varieties at different periods of infection (Fig. S3). In addition, JrLEA22 was highly expressed only in anthracnose-resistant varieties, and its expression level increased with time and was significantly different from that of anthracnose-sensitive varieties, indicating that it might be closely associated with the process of anthracnose resistance in walnuts.
Discussion
The Persian walnut (J. regia) and its wild relative, J. mandshurica, are both economically valuable tree species [38]. With the development and maturation of genome sequencing technologies in recent years, comparative genomic studies of the two Juglans species have become a current research hotspot [11, 29]. Late Embryogenesis Abundant (LEA) proteins are an important class of plant proteins that accumulate mainly at later stages of seed development in response to exogenous stresses [1]. The LEA gene family has been extensively studied in plant species, with reports of a total of 51 LEA members identified in Arabidopsis [14], 84 members identified in the banana [43], and 72 members identified in the sweet orange [44]. We identified a significant difference in the number of the identified LEAs in J. regia and J. mandshurica (Fig. 1). Phylogenetic analysis showed that these LEA genes can be divided into eight branches corresponding to their unique structural domains and mRNA homology, indicating that the LEA gene family members of these two walnuts are more closely related (Fig. 1). Most LEAs were localized in the cytoplasm and nucleus (Table 1) [45]. The LEA gene members of J. regia and J. mandshurica were distributed heterogeneously across the chromosomes, but some LEA gene clusters had high similarity and collinearity (Figs. 2 and 4). This clustering phenomenon might prevent LEA genes from losing critical functions as they evolve. The results of the collinearity analysis indicate individual LEA genes that were specific to J. regia or J. mandshurica (Tables S3 and S4). These genes may be related to the specificity of the two Juglans species. Most LEA homologous gene pairs had Ka/Ks ratios less than 1, suggesting that JrLEAs and JmLEAs were subject to purifying selection during evolution (Table S3). Duplication events such as segmental type can extend the family members in plant species and modifications as well as point mutations in the gene structure including promoter region and coding sequence site can increase the diversity and modify the expression patterns of new duplicated members [46, 47]. WGD events are a common mechanism for gene duplication [42]. In the present study, we found WGD events to account for the largest proportion of gene duplication categories, corresponding to 56% in J. regia and 50% in J. mandshurica, suggesting the important role of WGD in the duplication of the LEA gene family in the two Juglans species (Table 2). However, we observed only a few TD gene pairs (12% in J. regia and 5% in J. mandshurica), presenting similar proportions observed in other species with TD, accounting for 9.6% in the banana [43] and 6.3% in maize [17]. Although structural domains steadily arose in the identified LEAs, they presented variable genic structures in J. regia and J. mandshurica (Fig. 3).
The LEA genes of a variety of plants are involved in response processes to biotic and abiotic stresses [26]. The overexpression of the OsLEA3–2 gene revealed that, under salt stress or osmotic stress conditions, transgenic rice grew significantly stronger than the control and was able to recover after 20 days of drought stress [12]. The expression profile analysis indicated an important role of the MwLEA1 gene cloned from Agropyron mongolicum in water and salt stresses as well as in abscisic acid regulation [48]. The overexpression of ZmLEA3 in transgenic tobacco and yeast can enhance tolerance to osmotic and oxidative stress, enabling plants to withstand stress by protecting their protein structures [17]. Despite extensive research in the past, the specific regulation mechanism of LEA proteins that responds to abiotic and biotic stress remains unclear. Investigating the phylogeny, gene structures, and expression profiles of the LEA gene family can provide useful information for further studies on LEA gene evolution and gene function.
Cis-acting elements were involved in the regulation of gene expression, and a large number of cis-acting elements detected in this study provided strong evidence for the involvement of JrLEAs and JmLEAs in abiotic stress responses. We found that the upstream LEA promoter region contained several cis-acting elements in response to methyl jasmonate (MeJA) and abscisic acid (ABA) (Fig. 5), suggesting that LEA proteins might be involved in plant responses to MeJA and ABA regulation. The synthesis, expression, and physiological activity of LEA proteins are regulated by many factors (e.g., the developmental stage, hormones, ionic changes, and dehydration) and signal transduction pathways [47]. Phytohormones are usually involved in stress responses in plant and are essential for plant adaptation to adverse environmental conditions [49]. Most of the identified plants’ LEA gene families have phytohormone-responsive elements, such as tomato [50] and wheat [51]. For example, LEA gene expression is regulated by ABA-dependent pathways during seed development and desiccation [8]. Thus, these results suggest that LEA genes play an important role in the stress responses of plants.
Perennial crops were once regarded as intractable systems due to their large size and their long juvenile phase and generation length. Recently, the development of perennial crops has been garnering increasing attention, as they are essential components for sustainable agriculture that provide alternative food sources under changing environments. The published high quality Juglans genome provided an excellent opportunity to deeply explore the functional network and regulation mechanism of the candidate genes. LEA genes were once considered to be responsive to abiotic stress; however, they also play a pivotal role in biotic resistance [17]. Anthracnose causes early tree defoliation at critical times (nut filling and ripening) and leads to alternate-year bearing for nut crops [51], as it is one of the major diseases of walnuts. In our study, in addition to the analysis and discussion of abiotic stress and the analysis of cis-acting elements, we also explored LEA expression patterns in various tissues and in anthracnose-resistant varieties (F26), as well as in anthracnose-susceptible varieties (F423).
JrLEAs were expressed in leaves, green husks, male flowers, and female flowers, suggesting that these genes play an important role in the development of all four selected tissues. In contrast, most JmLEAs might play a role in other organs or in other developmental stages of the selected tissues. JrLEA8 and JrLEA19 were highly expressed in both leaves and green husks and had similar expression patterns, suggesting that they might possess the function of synergetic regulation in the development of leaves and green husks. Five JmLEAs were highly expressed in the male flowers of J. mandshurica, suggesting that these genes are more valuable candidates for studying male flower development in J. mandshurica (Fig. 6b; Table S6). The majority of LEA genes targeted by ath-miR414 were highly expressed in female flowers, suggesting that it may regulate female flower development by targeting LEA genes in the two Juglans species (Fig.S1, Table S7). Plants in Juglans experience dichogamy [52]. JrLEA4 and JrLEA22 (more highly expressed in female flowers than in male flowers) as well as JrLEA2 and JrLEA5 (more highly expressed in male flowers than in female flowers) are thought to have a possible direct or indirect effect on dichogamy in J. regia. Six genes were more highly expressed in male flowers than in female flowers, and two genes presented the opposite pattern, suggesting that LEA genes also influence dichogamy in J. mandshurica. The tissue-specific expression of these LEAs may be associated with dichogamy in two Juglans species, although the regulatory mechanisms involved remain to be further investigated.
In addition, we found higher expression levels of LEA genes for anthracnose-resistant varieties (F26) than those for anthracnose-susceptible varieties (F423), indicating that LEA genes might play a role in anthracnose resistance in J. regia (Fig. 6c; Table S8). Moreover, the expression patterns of genes belonging to the same subgroup were more similar, such as those of JrLEA15 and JrLEA21 (SMP), JrLEA3 and JrLEA23 (LEA_5), and JrLEA18 and JrLEA24 (Dehydrin). The expression levels of most JrLEAs increase with time within 48 h after infection [38]. JrLEA12 and JrLEA22 were only expressed in F26 varieties, indicating that these two genes are closely associated with anthracnose resistance in J. regia. In addition, the LEA gene family was also shown to be a gene family associated with disease resistance in J. mandshurica [29]. A similar function was found in ZmLEA3, which can respond to biotic stresses, and the overexpression of ZmLEA3 in tobacco results in its increased tolerance to the pathogen pst dc3000 (the pathogen Pseudomonas syringae pv. Tomato DC3000) [17]. These results suggest that LEAs are not only able to respond to abiotic stresses but also to biotic stresses. J. regia is more susceptible to disease than its wild relative, J. mandshurica [29]. J. mandshurica has been shown to have better resistance to disease to lesion nematode [29]. Therefore, J. mandshurica is recommended as a rootstock for J. regia to confer disease tolerance/resistance [24, 53,54,55,56]. Furthermore, speculation indicates that JmLEAs may exhibit better expression for biotic stresses compared with JrLEAs, but further studies are still needed in this regard.
Conclusion
In this study, we systematically identify LEA gene family members in J. regia and its wild relative, J. mandshurica. The phylogenetic analysis showed that the LEA genes in J. regia and J. mandshurica are divided into eight subgroups, similar to results from other plants. The phylogenetic and collinearity analyses indicated that the LEA gene family is relatively evolutionarily conserved. The analysis of cis-acting elements and gene expression indicated that JrLEAs and JmLEAs play important roles in resistance to biotic stresses in J. regia and J. mandshurica. Further exploration is necessary to determine the specific roles of JrLEAs and JmLEAs in other stresses, such as metal ion stress, extreme temperatures, UV radiation, etc.
Availability of data and materials
The raw data were downloaded from the SRA database under accession number (GSE147083).
Abbreviations
- LEA:
-
Late Embryogenesis Abundant
- SMP:
-
Seed maturation protein
- ML:
-
Maximum likelihood
- GRAVY:
-
Grand average of hydropathicity
- WGD:
-
Whole genome duplication
- TD:
-
Tandem duplication
- DSD:
-
Dispersed duplication
References
Xiang L. Molecular evolutionary research of the plant LEA gene family and preliminary analysis of an atypical LEA gene in Arabidopsis: Jiangsu University; 2016.
Hoekstra FA, Golovina EA, Buitink J. Mechanisms of plant desiccation tolerance. Trends Plant Sci. 2001;6(9):431–8.
Amara I, Zaidi I, Masmoudi K, et al. Insights into late embryogenesis abundant (LEA) proteins in plants: from structure to the functions. Am J Plant Sci. 2014;05(22):3440–5.
Stevenson SR, Kamisugi Y, Trinh CH, et al. Genetic analysis of Physcomitrella patens identifies ABSCISIC ACID NON-RESPONSIVE, a regulator of ABA responses unique to basal land plants and required for desiccation tolerance. Plant Cell. 2016;28(6):1310–27.
Xiong L, Schumaker K, Zhu J. Cell signaling during cold, drought, and salt stress. Plant Cell. 2002;14(suppl_1):S165–S83.
Mackerness S. Plant responses to ultraviolet-B (UV-B: 280–320 nm) stress: what are the key regulators? Plant Growth Regul. 2000;32(1):27–39.
Slaughter A, Daniel X, Flors V, et al. Descendants of primed Arabidopsis plants exhibit resistance to biotic stress. Plant Physiol. 2011;158(2):835–43.
Shao H, Liang Z, Shao M. LEA proteins in higher plants: structure, function, gene expression and regulation. Colloid Surface B. 2005;45(3–4):131–5.
Tunnacliffe A, Wise MJ. The continuing conundrum of the LEA proteins. Naturwissenschaften. 2007;94(10):791–812.
Hunault G, Jaspard E. LEAPdb: a database for the late embryogenesis abundant proteins. BMC Genomics. 2010;11:221.
Dure L, Crouch M, Harada J, et al. Common amino acid sequence domains among the LEA proteins of higher plants. Plant Mol Biol. 1989;12(5):475–86.
Yu J, Lai Y, Wu X, et al. Overexpression of OsEm1 encoding a group I LEA protein confers enhanced drought tolerance in rice. Biochem Biophys Res Commun. 2016;478(2):703–9.
Lim C, Lim S, Baek W, et al. The pepper late embryogenesis abundant protein CaLEA1 acts in regulating abscisic acid signaling, drought and salt stress response. Physiol Plant. 2015;154(4):526–42.
Jia F, Qi S, Li H, et al. Overexpression of late embryogenesis abundant 14 enhances Arabidopsis salt stress tolerance. Biochem Biophys Res Commun. 2014;454(4):505–11.
Wang M, Li P, Li C, et al. SiLEA14, a novel atypical LEA protein, confers abiotic stress resistance in foxtail millet. BMC Plant Biol. 2014;14:290.
Wang L, Li X, Chen S, et al. Enhanced drought tolerance in transgenic Leymus chinensis plants with constitutively expressed wheat TaLEA3. Biotechnol Lett. 2009;31(2):313–9.
Liu Y, Wang L, Xing X, et al. ZmLEA3, a multifunctional group 3 LEA protein from maize (Zea mays L.), is involved in biotic and abiotic stresses. Plant Cell Physiol. 2013;54(6):944–59.
Wu Y, Liu C, Kuang J, et al. Overexpression of SmLEA enhances salt and drought tolerance in Escherichia coli and salvia miltiorrhiza. Protoplasma. 2014;251(5):1191–9.
Woodworth R. Meiosis of microsporogenesis in the Juglandaceae. Am J Bot. 1930;17:863–9.
Schneider SJ, Hwang AY, Land SD, et al. Genetic diversity of ten black walnut (Juglans nigra L.) cultivars and construction of a mapping population. Tree Genet Genomes. 2019;15:62.
Han H, Woeste K, Hu Y, et al. Genetic diversity and population structure of common walnut (Juglans regia) in China based on EST-SSRs and the nuclear gene phenylalanine ammonia-lyase (PAL). Tree Genet Genomes. 2016;12:111.
Bai W, Liao W, Zhang D. Nuclear and chloroplast DNA phylogeography reveal two refuge areas with asymmetrical gene flow in a temperate walnut tree from East Asia. New Phytol. 2010;188(3):892–901.
Bai W, Wang W, Zhang D. Contrasts between the phylogeographic patterns of chloroplast and nuclear DNA highlight a role for pollen mediated gene flow in preventing population divergence in an east Asian temperate tree. Mol Phylogenet Evol. 2014;81:37–48.
Hu Z, Zhang T, Gao X, et al. De novo assembly and characterization of the leaf, bud, and fruit transcriptome from the vulnerable tree Juglans mandshurica for the development of 20 new microsatellite markers using Illumina sequencing. Mol Gen Genomics. 2016;291(2):849–62.
Lu AM. On the geographical dispersal of Juglandaceae. Acta Phytotaxonomica Sinica. 1982;20:257–74.
Cheng Z, Zhang X, Yao W, et al. Genome-wide search and structural and functional analyses for late embryogenesis-abundant (LEA) gene family in poplar. BMC Plant Biol. 2021;21:110.
Yasemin CA, Pinar B, Esta NY, et al. Identification and expression analysis of LEA gene family members in cucumber genome. Plant Growth Regul. 2016;80:225–41.
Liang Y, Xiong Z, Zheng J, et al. Genome-wide identification, structural analysis and new insights into late embryogenesis abundant (LEA) gene family formation pattern in Brassica napus. Sci Rep. 2016;6:24265.
Yan F, Xi R, She R, et al. Improved de novo chromosome-level genome assembly of the vulnerable walnut tree Juglans mandshurica reveals gene family evolution and possible genome basis of resistance to lesion nematode. Mol Ecol Resour. 2021;21:2063–76.
Chen C, Chen H, Zhang Y, et al. TBtools: an integrative toolkit developed for interactive analyses of big biological data. Mol Plant. 2020;13:1194–202.
Wang Y, Tang H, Debarry JD, et al. MCScanX: a toolkit for detection and evolutionary analysis of gene synteny and collinearity. Nucleic Acids Res. 2012;40:e49.
Wang D, Zhang Y, Zhang Z, et al. KaKs_Calculator 2.0: a toolkit incorporating gamma-series methods and sliding window strategies. Genom Proteom Bioinf. 2010;8(1):77–80.
Nguyen LT, Schmidt HA, Haeseler A, et al. IQ-TREE: a fast and effective stochastic algorithm for estimating maximum-likelihood phylogenies. Mol Biol Evol. 2015;32(1):268–74.
Dai X, Zhuang Z, Zhao P. psRNATarget: a plant small RNA target analysis server (2017 release). Nucleic Acids Res. 2018;46(01):W49–54.
Shannon P, Markiel A, Ozier O, et al. Cytoscape: a software environment for integrated models of biomolecular interaction networks. Genome Res. 2003;13:2498–504.
Li M, Ma J, Liu H, et al. Identification and characterization of wall-associated kinase (WAK) and WAK-like (WAKL) gene family in Juglans regia and its wild related species Juglans mandshurica. Genes. 2022;13(1):134.
Liu H, Ye H, Wang J, et al. Genome-wide identification and characterization of YABBY gene family in Juglans regia and Juglans mandshurica. Agronomy. 2022;12:1914.
Feng S, Feng H, Liu X, et al. Genome-wide identifification and characterization of long non-coding RNAs conferring resistance to Colletotrichum gloeosporioides in walnut (Juglans regia). BMC Genomics. 2021;22:15.
Chen S, Zhou Y, Chen Y, et al. Fastp: an ultra-fast all-in-one FASTQ preprocessor. Bioinformatics. 2018;34(17):i884–9.
Kim D, Langmead B, Salzberg SL. HISAT: a fast spliced aligner with low memory requirements. Nat Methods. 2015;12:357–60.
Liao Y, Smyth GK, Shi W. featureCounts: an efficient general purpose program for assigning sequence reads to genomic features. Bioinformatics. 2014;30(7):923–30.
Livak KJ, Schmittgen TD. Analysis of relative gene expression data using real-time quantitative PCR and the 2(−Delta Delta C(T)) method. Methods. 2001;25(4):402–8.
Piyatissa S, Bandupriya D. Genome-wide identification and analysis of late embryogenesis abundant (LEA) genes in Musa acuminata. Tropical Plant Biol. 2021;14:295–312.
Pedrosa A, Martins C, Gonçalves L, et al. Late embryogenesis abundant (LEA) constitutes a large and diverse family of proteins involved in development and abiotic stress responses in sweet orange (Citrus sinensis L. Osb.). PLoS One. 2015;10:e0145785.
Magwanga R, Lu P, Kirungu J, et al. Characterization of the late embryogenesis abundant (LEA) proteins family and their role in drought stress tolerance in upland cotton. BMC Genet. 2018;19(1):6.
Heidari P, Puresmaeli F, Mora-Poblete F. Genome-wide identification and molecular evolution of the magnesium transporter (MGT) gene family in Citrullus lanatus and Cucumis sativus. Agronomy. 2022;12:2253.
Faraji S, Heidari P, Amouei H, et al. Investigation and computational analysis of the sulfotransferase (SOT) gene family in potato (Solanum tuberosum): insights into sulfur adjustment for proper development and stimuli responses. Plants. 2021;10:2597.
Zhao Y, Yun J, Shi F, et al. Molecular cloning and characterization of a group 3 LEA gene from Agropyron mongolicum keng. African J Biotechnol. 2010;9:6040–8.
Liang Y, Wan N, Cheng Z, et al. Whole-genome identification and expression pattern of the vicinal oxygen chelate family in rapeseed (Brassica napus L.). Front Plant Sci. 2017;8:745.
Cao J, Li X. Identification and phylogenetic analysis of late embryogenesis abundant proteins family in tomato (Solanum lycopersicum). Planta. 2015;241:757–72.
Liu H, Xing M, Yang W, et al. Genome-wide identification of and functional insights into the late embryogenesis abundant (LEA) gene family in bread wheat (Triticum aestivum). Sci Rep. 2019;9:13375.
Zhu Y, Yin Y, Yang K, et al. Construction of a high-density genetic map using specific length amplified fragment markers and identification of a quantitative trait locus for anthracnose resistance in walnut (Juglans regia L.). BMC Genomics. 2015;16(1):614.
Chen G, Pi XM, Yu CY. A new naphthalenone isolated from the green walnut husks of Juglans mandshurica maxim. Nat Prod Res. 2015;29(2):174–9.
Ji L, Zhang Y, Yang Y, et al. Long-term effects of mixed planting on arbuscular mycorrhizal fungal communities in the roots and soils of Juglans mandshurica plantations. BMC Microbiol. 2020;20(1):304.
Trouern-Trend A, Falk T, Zaman S, et al. Comparative genomics of six Juglans species reveals disease-associated gene family contractions. Plant J. 2020;102(2):410–23.
Zhou Z, Han M, Hou M, et al. Comparative study of the leaf transcriptomes and ionoms of Juglans regia and its wild relative species Juglans cathayensis. Acta Physiol Plant. 2017;39(10):224.
Acknowledgments
Not applicable.
Funding
This work was supported by the National Natural Science Foundation of China (32070372 and 32200295) and Science Foundation for Distinguished Young Scholars of Shaanxi Province (2023-JC-JQ-22).
Author information
Authors and Affiliations
Contributions
PZ designed and conceptualized the project. JM, DZ, and YY collected samples. JM, DZ, HY, and ML performed the data analysis. JM and YY performed the experiments. JM and DZ wrote the manuscript. HY and PZ revised the manuscript accordingly. All authors reviewed the manuscript. The author(s) read and approved the final manuscript.
Corresponding author
Ethics declarations
Ethics approval and consent to participate
This study has been approved by the Chinese government and carried out with the laws of the People’s Republic of China. All participants had a license approval letter from the College of Life Sciences, Northwest University. All participants obtained the permissions to collect J. regia and J. mandshurica samples from Zhuque National Forest Park (ZNFP). All methods were carried out in accordance with relevant guidelines and regulations.
Consent for publication
Not applicable.
Competing interests
The authors declare no competing interests.
Additional information
Publisher’s Note
Springer Nature remains neutral with regard to jurisdictional claims in published maps and institutional affiliations.
Supplementary Information
Additional file 1: Figure S1.
Protein interaction network and schematic representation of the regulatory network relationships between the putative miRNAs and their targeted LEA genes. Elliptics represent proteins, rectangles represent miRNAs. The black line indicates protein interactions and the pink line indicates miRNA targeting of LEA genes. Figure S2. Expression patterns of LEA genes without collinearity in four selected organs of two Juglans species. The colored scale reflects gene expression levels. Figure S3. Expression patterns of LEA genes without collinearity under biotic stress of J. regia. F26 indicated anthracnose-resistant varieties, F423 indicated anthracnose-susceptible varieties. The colored scale reflects gene expression levels.
Additional file 2: Table S1.
Primers for the qRT-PCR experiment. Table S2. Protein sequences of all LEA genes in J. regia and J. mandshurica. Table S3. Homologous LEA gene pairs and Ka/Ks values in J. regia and J. mandshurica. Table S4. Homologous LEA gene pairs in Arabidopsis and two Juglans species. Table S5. The FPKM values of all LEA genes in different tissues of J. regia. Table S6. The FPKM values of all LEA genes in different tissues of J. mandshurica. Table S7. The putative miRNAs and their targeted LEA genes. Table S8. The FPKM values of all LEA genes in anthracnose-resistant varieties (F26) and anthracnose-susceptible varieties (F423) with the time after infection of J. regia.
Rights and permissions
Open Access This article is licensed under a Creative Commons Attribution 4.0 International License, which permits use, sharing, adaptation, distribution and reproduction in any medium or format, as long as you give appropriate credit to the original author(s) and the source, provide a link to the Creative Commons licence, and indicate if changes were made. The images or other third party material in this article are included in the article's Creative Commons licence, unless indicated otherwise in a credit line to the material. If material is not included in the article's Creative Commons licence and your intended use is not permitted by statutory regulation or exceeds the permitted use, you will need to obtain permission directly from the copyright holder. To view a copy of this licence, visit http://creativecommons.org/licenses/by/4.0/. The Creative Commons Public Domain Dedication waiver (http://creativecommons.org/publicdomain/zero/1.0/) applies to the data made available in this article, unless otherwise stated in a credit line to the data.
About this article
Cite this article
Ma, J., Zuo, D., Ye, H. et al. Genome-wide identification, characterization, and expression pattern of the late embryogenesis abundant (LEA) gene family in Juglans regia and its wild relatives J. mandshurica. BMC Plant Biol 23, 80 (2023). https://doi.org/10.1186/s12870-023-04096-z
Received:
Accepted:
Published:
DOI: https://doi.org/10.1186/s12870-023-04096-z