Abstract
Silicon carbide (SiC) with various morphologies was employed as reinforcing fillers for poly(ethylene oxide) (PEO)-based solid polymer electrolytes (SPEs). Specifically, the SiC nanoparticles with an average size of 5–10 µm endow a significant enhancement of the interaction between carbon atoms for the fillers and the oxygen atoms from the PEO–lithium bis(trifluoromethanesulfonyl)imide (LiTFSI) matrix, which provides rapid transport channels for mobile Li+ and enhances the mechanical strength of SPEs. The SPEs reinforced with one-dimensional SiC nanoparticles exhibit superior Li+ conduction, good mechanical property, and uniform Li plating. Thus, a dendrite-free plating of lithium for 0.58 mAh at 0.1 mA·cm–2 and excellent cycle stability at various current densities for 250 h are achieved in Li/Li symmetric cells using SPEs electrolytes with 5.0 wt% SiC nanoparticles. Moreover, the LiFePO4/Li full cells assembled using SPEs electrolytes with 5.0 wt% SiC nanoparticles provide a capacity of 151.5 mAh·g–1 at 0.1 mA·cm–2 (55 °C), and maintained a Coulombic efficiency of 99% for 120 cycles.
Graphical abstract

摘要
将四种不同直径和长径比的一维SiC晶须添加到PEO/LiTFSI聚合物中制备全固态电池用复合锂离子固态电解质 (solid polymer electrolytes, 简称SPEs), 分析其组织结构、力学行为和电化学性能. 研究发现, SiC晶须掺杂可以有效提高PEO/LiTFSI聚合物的机械强度, 固态电解质SiC表面与PEO/LiTFSI基体中的氧离子具有比较强的相互作用为锂离子快速迁移提供传输通道. SiC晶须均匀分布在PEO/LiTFSI聚合物电解质中, 直径 5–10 µm、长度~50 µm 的一维SiC晶须强化SPEs展现出最优的锂离子导电性和力学性能. 采用SiC晶须含量为5 wt%电解质组装的Li/SPE/Li对称电池, 不同电流密度条件下经过250次充放电循环仍展现出良好的稳定性. 此外, 采用SiC含量为5 wt%电解质组装的LiFePO4/SPE/Li全固态电池, 在55°C、电流0.1 mA·cm–2时其初始放电电容量达到151.5 mAh·g–1, 拥有稳定的循环性和比较高的库伦效率.





Similar content being viewed by others
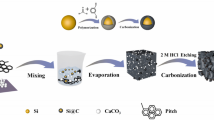
References
Armand M, Tarascon JM. Building better batteries. Nature. 2008;451:652. https://doi.org/10.1038/451652a.
Hu YS. Batteries: getting solid. Nat Energy. 2016;1:16042. https://doi.org/10.1038/nenergy.2016.42.
Ji YR, Weng ST, Li XY. Atomic-scale structural evolution of electrode materials in Li-ion batteries: a review. Rare Met. 2020; 39(3):205. https://doi.org/10.1007/s12598-020-01369-6.
Nakamura T, Amezawa K, Kulisch J, Zeier WG, Janek J. Guidelines for all-solid-state battery sesign and electrode buffer layers based on chemical potential profile calculation. ACS Appl Mater Interfaces. 2019;11:19968. https://doi.org/10.1021/acsami.9b03053.
Cheng XB, Zhang R, Zhao CZ, Zhang Q. Toward safe lithium metal anode in rechargeable batteries: a review. Chem Rev. 2017;117:10403. https://doi.org/10.1021/acs.chemrev.7b00115.
Yan CL. Realizing high performance of solid-state lithium metal batteries by flexible ceramic/polymer hybrid solid electrolyte. Rare Met. 2020; 39(5):458. https://doi.org/10.1007/s12598-020-01417-1.
Cheng XB, Zhao CZ, Yao YX, Liu H, Zhang Q. Recent advances in energy chemistry between solid-state electrolyte and safe lithium-metal anodes. Chem. 2019;5:74. https://doi.org/10.1016/j.chempr.2018.12.002.
Zhao Q, Stalin S, Zhao CZ, Archer LA. Designing solid-state electrolytes for safe, energy-dense batteries. Nat Rev Mater. 2020;5:229. https://doi.org/10.1038/s41578-019-0165-5.
Cao D, Sun X, Li Q, Natan A, Xiang P, Zhu H. Lithium dendrite in all-solid-state batteries: growth mechanisms, suppression strategies, and characterizations. Matter. 2020;3:57. https://doi.org/10.1016/j.matt.2020.03.015.
Li Y, Xu B, Xu H, Duan H, Lu X, Xin S, Zhou W, Xue L, Fu G, Manthiram A, Goodenough JB. Hybrid polymer/garnet electrolyte with a small interfacial resistance for lithium-ion batteries. Angew Chem Int Ed Engl. 2017;56:753. https://doi.org/10.1002/anie.201608924.
Chen L, Li Y, Li SP, Fan LZ, Nan CW, Goodenough JB. PEO/garnet composite electrolytes for solid-state lithium batteries: from “ceramic-in-polymer” to “polymer-in-ceramic”. Nano Energy. 2018;46:176. https://doi.org/10.1002/anie.201608924.
Xu B, Li X, Yang C, Li Y, Grundish NS, Chien PH, Dong K, Manke I, Fang R, Wu N, Xu H, Dolocan A, Goodenough JB. Interfacial chemistry enables stable cycling of all-solid-state Li metal batteries at high current densities. J Am Chem Soc. 2021;143:6542. https://doi.org/10.1021/jacs.1c00752.
Wu N, Chien PH, Qian Y, Li Y, Xu H, Grundish NS, Xu B, Jin H, Hu YY, Yu G, Goodenough JB. Enhanced surface interactions enable fast Li+ conduction in oxide/polymer composite electrolyte. Angew Chem Int Ed Engl. 2020;59:4131. https://doi.org/10.1002/anie.201914478.
Wu N, Chien PH, Li Y, Dolocan A, Xu H, Xu B, Grundish NS, Jin H, Hu YY, Goodenough JB. Fast Li+ conduction mechanism and interfacial chemistry of a NASICON/Polymer composite electrolyte. J Am Chem Soc. 2020;142:2497. https://doi.org/10.1021/jacs.9b12233.
Chen S, Wang J, Zhang Z, Wu L, Yao L, Wei Z, Deng Y, Xie D, Yao X, Xu X. In-situ preparation of poly(ethylene oxide)/Li3PS4 hybrid polymer electrolyte with good nanofiller distribution for rechargeable solid-state lithium batteries. J Power Sources. 2018;387:72. https://doi.org/10.1016/j.jpowsour.2018.03.016.
Xu H, Chien PH, Shi J, Li Y, Wu N, Liu Y, Hu YY, Goodenough JB. High-performance all-solid-state batteries enabled by salt bonding to perovskite in poly(ethylene oxide). Proc Natl Acad Sci USA. 2019;116:18815. https://doi.org/10.1073/pnas.1907507116.
Kalnaus S, Sabau AS, Tenhaeff WE, Dudney NJ, Daniel C. Design of composite polymer electrolytes for Li ion batteries based on mechanical stability criteria. J Power Sources. 2012;201:280. https://doi.org/10.1016/j.jpowsour.2011.11.020.
Liu R, He P, Wu Z, Guo F, Huang B, Wang Q, Huang Z, Wang C, Li Y. PEO/hollow mesoporous polymer spheres composites as electrolyte for all solid state lithium ion battery. J Electroanal Chem. 2018;822:105. https://doi.org/10.1016/j.jelechem.2018.05.021.
Liu R, Wu Z, He P, Fan H, Huang Z, Zhang L, Chang X, Liu H, Wang C, Li Y. A self-standing, UV-cured semi-interpenetrating polymer network reinforced composite gel electrolytes for dendrite-suppressing lithium ion batteries. J Mater. 2019;5:185. https://doi.org/10.1016/j.jmat.2018.12.006.
Zou Z, Li Y, Lu Z, Wang D, Cui Y, Guo B, Li Y, Liang X, Feng J, Li H, Nan CW, Armand M, Chen L, Xu K, Shi S. Mobile ions in composite solids. Chem Rev. 2020;120:4169. https://doi.org/10.1021/acs.chemrev.9b00760.
Wang X, Zhai H, Qie B, Cheng Q, Li A, Borovilas J, Xu B, Shi C, Jin T, Liao X, Li Y, He X, Du S, Fu Y, Dontigny M, Zaghib K, Yang Y. Rechargeable solid-state lithium metal batteries with vertically aligned ceramic nanoparticle/polymer composite electrolyte. Nano Energy. 2019;60:205. https://doi.org/10.1016/j.nanoen.2019.03.051.
Zhu P, Yan C, Dirican M, Zhu J, Zang J, Selvan RK, Chung CC, Jia H, Li Y, Kiyak Y, Wu N, Zhang X. Li0.33La0.557TiO3 ceramic nanofiber-enhanced polyethylene oxide-based composite polymer electrolytes for all-solid-state lithium batteries. J Mater Chem A. 2018;6:4279. https://doi.org/10.1039/C7TA10517G.
Chen H, Adekoya D, Hencz L, Ma J, Chen S, Yan C, Zhao H, Cui G, Zhang S. Stable seamless interfaces and rapid ionic conductivity of Ca–CeO2/LiTFSI/PEO composite electrolyte for high-rate and high-voltage all-solid-state battery. Adv Energy Mater. 2020;10:2000049. https://doi.org/10.1002/aenm.202000049.
Yu W, Deng N, Yan Z, Gao L, Cheng K, Tian X, Tang L, Cheng B, Kang W. Mg-based inorganic nanofibers constructing fast and multi-dimensionalion conductive pathways for all-solid-state lithium metal batteries. J Energy Chem. 2022;67:684. https://doi.org/10.1016/j.jechem.2021.11.015.
Gao L, Wu N, Deng N, Li Z, Li J, Che Y, Cheng B, Kang W, Liu R, Li Y. Optimized CeO2 nanowires with rich surface oxygen vacancies enable fast Li-ion conduction in composite polymer electrolytes. Energy Environ Mater. 2021. https://doi.org/10.1002/EEM2.12272.
Gong Y, Fu K, Xu S, Dai J, Hamann TR, Zhang L, Hitz GT, Fu Z, Ma Z, McOwen DW, Han X, Hu L, Wachsman ED. Lithium-ion conductive ceramic textile: a new architecture for flexible solid-state lithium metal batteries. Mater Today. 2018;21:594. https://doi.org/10.1016/j.mattod.2018.01.001.
Zheng J, Tang M, Hu YY. Lithium ion pathway within Li7La3Zr2O12-polyethylene oxide composite electrolytes. Angew Chem Int Ed Engl. 2016;55:12538. https://doi.org/10.1002/ange.201607539.
Wei WQ, Liu BQ, Gan YQ. Protecting lithium metal anode in all-solid-state batteries with a composite electrolyte. Rare Met. 2021;40(2):409. https://doi.org/10.1007/s12598-020-01501-6.
Li Z, Huang HM, Zhu JK, Wu JF, Yang H, Wei L, Guo X. Ionic conduction in composite polymer electrolytes: case of PEO:Ga-LLZO composites. ACS Appl Mater Interfaces. 2019;11:784. https://doi.org/10.1021/acsami.8b17279.
Xu L, Li J, Shuai H, Luo Z, Wang B, Fang S, Zou G, Hou H, Peng H, Ji X. Recent advances of composite electrolytes for solid-state Li batteries. J Energy Chem. 2022;67:524. https://doi.org/10.1016/j.jechem.2021.10.038.
Liu Y, Xu B, Zhang W, Li L, Lin Y, Nan C. Composition modulation and structure design of inorganic-in-polymer composite solid electrolytes for advanced lithium batteries. Small. 2020;16:1902813. https://doi.org/10.1002/smll.201902813.
Randau S, Weber DA, Kötz O, Koerver R, Braun P, Weber A, Ivers-Tiffée E, Adermann T, Kulisch J, Zeier WG, Richter FH, Janek J. Benchmarking the performance of all-solid-state lithium batteries. Nat Energy. 2020;5:259.
Han D, Mei H, Xiao S, Dassios KG, Cheng L. A review on the processing technologies of carbon nanotube/silicon carbide composites. J Eur Ceram Soc. 2018;38:3695. https://doi.org/10.1016/j.jeurceramsoc.2018.04.033.
Shen D, Zhan Z, Liu Z, Cao Y, Zhou L, Liu Y, Dai W, Nishimura K, Li C, Lin CT, Jiang N, Yu J. Enhanced thermal conductivity of epoxy composites filled with silicon carbide nanowires. Sci Rep. 2017;7:2606. https://doi.org/10.1038/s41598-017-02929-0.
Huang Y, Hu J, Yao Y, Zeng X, Sun J, Pan G, Sun R, Xu JB, Wong CP. Manipulating orientation of silicon carbide nanowire in polymer composites to achieve high thermal conductivity. Adv Mater Interfaces. 2017;4:1700446. https://doi.org/10.1002/admi.201700446.
Durandurdu M. Electronic and mechanical properties of wurtzite type SiC nanowires. Phys Status Solidi (b). 2006;243:R37. https://doi.org/10.1002/pssb.200642041.
Li R, Qing Y, Zhao J, Huang S. SiC-coated carbon nanotubes with enhanced oxidation resistance and stable dielectric properties. Materials (Basel). 2021;14:2770. https://doi.org/10.3390/ma14112770.
Yang Z, Xia Y, Mokaya R. High surface area silicon carbide whiskers and nanotubes nanocast using mesoporous silica. Chem Mater. 2002;16:3877. https://doi.org/10.1021/cm048950x.
Kuang J, Cao W, Viehland D. Silicon carbide whiskers: preparation and high dielectric permittivity. J Am Ceram Soc. 2013;96:2877. https://doi.org/10.1111/jace.12393.
Li Y, Huang X, Hu Z, Jiang P, Li S, Tanaka T. Large dielectric constant and high thermal conductivity in poly(vinylidene fluoride)/barium titanate/silicon carbide three-phase nanocomposites. ACS Appl Mater Interfaces. 2011;3:4396. https://doi.org/10.1021/am2010459.
Hao JY, Wang YY, Tong XL, Jin GQ, Guo XY. SiC nanomaterials with different morphologies for photocatalytic hydrogen production under visible light irradiation. Catal Today. 2013;212:220. https://doi.org/10.1016/j.cattod.2012.09.023.
Acknowledgements
This study was financially supported by Shandong Province Natural Science Foundation (No. ZR2020ME001).
Author information
Authors and Affiliations
Corresponding authors
Ethics declarations
Conflict of interests
The authors declare that they have no conflict of interest.
Rights and permissions
About this article
Cite this article
Wei, WQ., Liu, BQ., Wang, YQ. et al. Silicon-carbide fiber-reinforced polymer electrolyte for all-solid-state lithium-metal batteries. Rare Met. 41, 3774–3782 (2022). https://doi.org/10.1007/s12598-022-02081-3
Received:
Revised:
Accepted:
Published:
Issue Date:
DOI: https://doi.org/10.1007/s12598-022-02081-3