Abstract
Shape memory polymers (SMP) have the potential to be utilized as a lightweight, solid state actuator in modern reconfigurable structures including as a deployment system for satellite solar panels or morphing aircraft wings. This paper is primarily concerned with the use of Veriflex-S® shape memory polymer and bi-directional carbon-fiber-reinforced-polymer (CFRP) in a flexural unimorph actuator configuration. One of the major deficiencies of SMP unimorphs is the permanent set (unrecovered shape) after a single or multiple temperature cycle(s). The novel concept of incorporating transverse curvature in the CFRP substrate, similar to that of an extendable tape measurer, is proposed to improve the shape recovery by increasing the bending stiffness of the unimorph actuator to compensate for the lack of recovery of the SMP. A set of experiments was designed to investigate the influence of transverse curvature, the relative widths of SMP and CFRP substrates, and shape memory polymer thickness on actuator recoverability after multiple thermo-mechanical cycles. The performance of SMP unimorph actuators with varying degrees of transverse curvature were evaluated versus that of traditional SMP unimorphs incorporating a flat substrate. Digital image correlation was implemented to quantify the out-of-plane deflection of the unimorph composite actuators (UCAs) during the actuation cycle. Experimental results indicate that an actuator with transverse curvature significantly reduces the residual deformation by at least two orders of magnitude which could be further tailored to enhance the performance of shape memory polymers in reconfigurable arrangements.



















Similar content being viewed by others
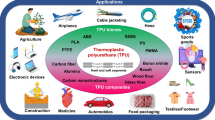
Abbreviations
- ρ:
-
Substrate Radius of Curvature
- c:
-
Polymer Width
- CF:
-
Carbon Fiber
- CFRP:
-
Carbon-Fiber-Reinforced Polymer
- CTE:
-
Coefficient of Thermal Expansion
- CV:
-
Coefficient of Variation
- DIC:
-
Digital Image Correlation
- L:
-
Actuator Length
- MAV:
-
Micro Air Vehicle
- s:
-
Substrate Width
- SMP:
-
Shape Memory Polymer
- t:
-
Polymer Thickness
- Tg :
-
Glass Transition Temperature
- UCA:
-
Unimorph Composite Actuator
- u,v,w:
-
Lengthwise, Widthwise, and Vertical Displacements
- x,y,z:
-
Lengthwise, Widthwise, and Vertical Coordinates
References
Nettles D (2009) Thermomechanical characterization of a shape memory polymer based syntactic foam. 1–83
Li G, Nettles D (2010) Thermomechanical characterization of a shape memory polymer based self-repairing syntactic foam. Polymer (Guildf) 51:755–762. doi:10.1016/j.polymer.2009.12.002
Volk BL (2009) Thermomechanical characterization amd modeling of shape memory polymers. 124
Liang F, Sivilli R, Gou J et al (2013) Electrical actuation and shape recovery control of shape-memory polymer nanocomposites. Int J Smart Nano Mater 4:167–178. doi:10.1080/19475411.2013.837846
Lu H, Liang F, Gou J (2011) Nanopaper enabled shape-memory nanocomposite with vertically aligned nickel nanostrand: controlled synthesis and electrical actuation. Soft Matter 7:7416–7423. doi:10.1039/c1sm05765k
Lu H, Gou J, Leng J, Du S (2011) Magnetically aligned carbon nanotube in nanopaper enabled shape-memory nanocomposite for high speed electrical actuation. Appl Phys Lett 98:174105. doi:10.1063/1.3585669
Yu X, Zhou S, Zheng X et al (2009) A biodegradable shape-memory nanocomposite with excellent magnetism sensitivity. Nanotechnology 20:235702. doi:10.1088/0957-4484/20/23/235702
Schmidt AM (2006) Electromagnetic activation of shape memory polymer networks containing magnetic nanoparticles. Macromol Rapid Commun 27:1168–1172. doi:10.1002/marc.200600225
Razzaq MY, Anhalt M, Frormann L, Weidenfeller B (2007) Mechanical spectroscopy of magnetite filled polyurethane shape memory polymers. Mater Sci Eng A 471:57–62. doi:10.1016/j.msea.2007.03.059
Beblo R V. (2010) Characterization and modeling of light activated shape memory polymer. 218
Lendlein A, Jiang H, Junger O, Langer R (2005) Light-induced shape-memory polymers. Nature 434:695–697. doi:10.1038/nature03438.1
Leng J, Zhang D, Liu Y et al (2010) Study on the activation of styrene-based shape memory polymer by medium-infrared laser light. Appl Phys Lett 96:111905. doi:10.1063/1.3353970
Vaia R (2005) Remote-controlled actuators. Nature 4:429–430
Maitland DJ, Metzger MF, Schumann D et al (2002) Photothermal properties of shape memory polymer micro-actuators for treating stroke. Lasers Surg Med 30:1–11. doi:10.1002/lsm.10007
Otsuka K, Wayman CM (1998) Shape memory materials, 1st ed. 284
LaCroix BW, Ifju PG (2012) Utilization and performance enhancements of multiple piezoelectric actuators on micro air vehicles. AIAA Aerosp. Sci. Meet. American Institute of Aeronautics and Astronautics, Nashville, pp 1–14
Lacroix BW, Ifju PG (2013) Macro fiber composites and substrate materials for MAV Wing Morphing. Soc. Exp. Mech. Society of Experimental Mechanics, Lombard, pp 1–13
Yakacki CM, Shandas R, Lanning C et al (2007) Unconstrained recovery characterization of shape-memory polymer networks for cardiovascular applications. Biomaterials 28:2255–2263. doi:10.1016/j.biomaterials.2007.01.030
Thill C, Etches J, Bond I, et al (2008) Morphing skins. Aeronaut J 1–23
Gross KE (2008) Mechanical characterization of shape memory polymers to assess candidacy as morphing aircraft skin. University of Pittsburgh
Yin W, Liu J, Leng J (2009) Deformation analysis of shape memory polymer for morphing wing skin under airflow. Front Mech Eng Chin 4:447–449. doi:10.1007/s11465-009-0062-5
Joo J, Smyers B, Beblo R et al (2011) Load-bearing multi-functional structure with direct thermal harvesting for thermally activated reconfigurable wing design. Int. Conf. Compos. Mater. Society of Composite Materals, Jeju, pp 1–6
Ko S-H, Bae J-S, Rho J-H (2014) Development of a morphing flap using shape memory alloy actuators: the aerodynamic characteristics of a morphing flap. Smart Mater Struct 23:074015. doi:10.1088/0964-1726/23/7/074015
Rauscher SG (2008) Testing and analysis of shape-memory polymers for morphing aircraft skin application by by. 160
Li G, Asce M, Xu T (2011) Thermomechanical characterization of shape memory polymer – based self-healing syntactic foam sealant for expansion joints. J Transp Eng 805–814. doi: 10.1061/(ASCE)TE.1943-5436.0000279
Nji J, Li G (2010) A biomimic shape memory polymer based self-healing particulate composite. Polymer (Guildf) 51:6021–6029. doi:10.1016/j.polymer.2010.10.021
Li G, John M (2008) A self-healing smart syntactic foam under multiple impacts. Compos Sci Technol 68:3337–3343. doi:10.1016/j.compscitech.2008.09.009
Fulcher JT, Karaca HE, Tandon GP, Lu YC (2012) Thermomechanical and shape memory properties of thermosetting shape memory polymer under compressive loadings. J Appl Polym Sci. doi:10.1002/app.38791
Fulcher JT (2011) Mechanical characterizations of environmentally conditioned shape memory polymers for reconfigurable aerospace structures. 65
Fulcher JT, Karaca HE, Tandon GP et al (2011) Multiscale characterization of water-,oil-,and uv-conditioned shape-memory polymer under compression. In: Proulx T (ed) Mechanics of time-dependent materials and processes in conventional and multifunctional materials. Society of Experimental Mechanics, Uncasville, pp 97–103
Fulcher JT, Lu YC, Tandon GP, Foster DC (2010) Thermomechanical characterization of shape memory polymers using high temperature nanoindentation. Polym Test 29:544–552. doi:10.1016/j.polymertesting.2010.02.001
Nahid MNH, Wahab MAA, Lian K (2011) Degradation of shape memory polymer due to water and diesel fuels. In: Proulx T (ed) Mechanics of time-dependent materials and processes in conventional and multifunctional materials. Society of Experimental Mechanics, Uncasville, pp 37–48
McClung AJW, Tandon GP, Goecke KE, Baur JW (2011) Non-contact technique for characterizing full-field surface deformation of shape memory polymers at elevated and room temperatures. Polym Test 30:140–149. doi:10.1016/j.polymertesting.2010.11.010
McClung AJW, Ruggles-Wrenn MB (2009) Strain rate dependence and short-term relaxation behavior of a thermoset polymer at elevated temperature: experiment and modeling. J Press Vessel Technol 131:031405. doi:10.1115/1.3110025
McClung AJW, Tandon GP, Baur JW (2011) Fatigue cycling of shape memory polymer resin. In: Proulx T (ed) Mechanics of time-dependent materials and processes in conventional and multifunctional materials. Springer New York, New York, pp 119–127
McClung AJW, Tandon GP, Baur JW (2011) Strain rate- and temperature-dependent tensile properties of an epoxy-based, thermosetting, shape memory polymer (Veriflex-E). Mech Time-Dependent Mater 16:205–221. doi:10.1007/s11043-011-9148-7
McClung AJW, Tandon GP, Baur JW (2011) Deformation rate-, hold time-, and cycle-dependent shape-memory performance of Veriflex-E resin. Mech Time-Dependent Mater 17:39–52. doi:10.1007/s11043-011-9157-6
Yu K, McClung AJW, Tandon GP et al (2014) A thermomechanical constitutive model for an epoxy based shape memory polymer and its parameter identifications. Mech Time-Dependent Mater 18:453–474. doi:10.1007/s11043-014-9237-5
Lu H (2013) State diagram of phase transition temperatures and solvent-induced recovery behavior of shape-memory polymer. J Appl Polym Sci 127:2896–2904. doi:10.1002/app.37683
Lu H, Liu Y, Gou J et al (2011) Surface coating of multi-walled carbon nanotube nanopaper on shape-memory polymer for multifunctionalization. Compos Sci Technol 71:1427–1434. doi:10.1016/j.compscitech.2011.05.016
Lu H, Yu K, Liu Y, Leng J (2010) Sensing and actuating capabilities of a shape memory polymer composite integrated with hybrid filler. Smart Mater Struct 19:065014. doi:10.1088/0964-1726/19/6/065014
Lu H, Liu Y, Leng J, Du S (2010) Qualitative separation of the physical swelling effect on the recovery behavior of shape memory polymer. Eur Polym J 46:1908–1914. doi:10.1016/j.eurpolymj.2010.06.013
Chowdhury AMS, Schmidt C, Neuking K, Eggeler G (2013) Comparative studies on the accumulation of strain and recovery ratio of Veriflex(R), a shape-memory polymer for a high strain (isin m = 210 %): Atomic force microscopic experiments. High Perform Polym 26:20–26. doi:10.1177/0954008313494907
Chowdhury AMS, Schmidt C, Neuking K, Eggeler G (2013) Comparative studies on the accumulation of strain and recovery ratio of Veriflex(R), a shape-memory polymer: infrared and laser experiments. High Perform Polym 25:886–893. doi:10.1177/0954008313487929
Liu Y, Gall K, Dunn ML et al (2006) Thermomechanics of shape memory polymers: Uniaxial experiments and constitutive modeling. Int J Plast 22:279–313. doi:10.1016/j.ijplas.2005.03.004
Cortes P, Terzak J, Kubas G et al (2014) The morphing properties of a vascular shape memory composite. Smart Mater Struct 23:015018. doi:10.1088/0964-1726/23/1/015018
Beblo R, Gross K, Mauck Weiland L (2010) Mechanical and curing properties of a styrene-based shape memory polymer. J Intell Mater Syst Struct 21:677–683. doi:10.1177/1045389X10364860
Beblo R, Weiland LM (2008) Strain induced anisotropic properties of shape memory polymer. Smart Mater Struct 17:055021. doi:10.1088/0964-1726/17/5/055021
Atli B, Gandhi F, Karst G (2008) Thermomechanical characterization of shape memory polymers. J Intell Mater Syst Struct 20:87–95. doi:10.1177/1045389X07086689
Carrell J, Tate D, Wang S, Zhang H-C (2011) Shape memory polymer snap-fits for active disassembly. J Clean Prod 19:2066–2074. doi:10.1016/j.jclepro.2011.06.027
Lan X, Liu Y, Lv H et al (2009) Fiber reinforced shape-memory polymer composite and its application in a deployable hinge. Smart Mater Struct 18:024002. doi:10.1088/0964-1726/18/2/024002
Leng J, Lu H, Liu Y, et al (2009) Shape-memory polymers — a class of novel smart materials. 34
Voit W, Ware T, Dasari RR et al (2010) High-strain shape-memory polymers. Adv Funct Mater 20:162–171. doi:10.1002/adfm.200901409
Monkman G (2000) Advances in shape memory polymer actuation. Mechatronics 10:489–498. doi:10.1016/S0957-4158(99)00068-9
Schmidt C, Neuking K, Eggeler G (2008) Functional fatigue of shape memory polymers. Adv Eng Mater 10:922–927. doi:10.1002/adem.200800213
Gall K, Mikulas M, Munshi NA et al (2000) Carbon fiber reinforced shape memory polymer composites. J Intell Mater Syst Struct 11:877–886. doi:10.1106/EJGR-EWNM-6CLX-3X2M
Beloshenko VA, Varyukhin VN, Voznyak YV (2005) The shape memory effect in polymers. Russ Chem Rev 74:265–283. doi:10.1070/RC2005v074n03ABEH000876
Liu C, Qin H, Mather PT (2007) Review of progress in shape-memory polymers. J Mater Chem 17:1543. doi:10.1039/b615954k
Vaia R, Baur J (2008) Materials science: adaptive composites. Science 319:420–421. doi:10.1126/science.1152931
Cantrell JT, LaCroix BW, Ifju PG (2013) Passive roll compensation on micro air vehicles with perimeter reinforced membrane wings. 51st AIAA Aerosp. Sci. Meet. American Institute of Aeronautics and Astronautics, Grapevine, Texas, pp 1–12
Ifju PG, Jenkins DA, Waszak MR, et al (2002) Flexible-wing-based micro air vehicles. J Am Inst Aeronaut Astronaut 1–13
Shyy W, Ifju P, Viieru D (2005) Membrane wing-based micro air vehicles. Appl Mech Rev 58:283. doi:10.1115/1.1946067
Stanford B, Ifju P (2008) Aeroelastic topology optimization of membrane structures for micro air vehicles. Struct Multidiscip Optim 38:301–316. doi:10.1007/s00158-008-0292-x
Albertani RJ, Stanford B, DeLoach R et al (2008) Wind-tunnel testing and modeling of a micro air vehicle with flexible wings. J Aircr 45:1025–1032. doi:10.2514/1.33338
Ifju P, Lee K, Albertani R et al (2008) Bendable wing for micro air vehicle. 2:11
Jagdale V, Stanford B, Claxton D et al (2006) Experimental characterization of load stiffening wing for small UAV. Soc Exp Mech 1–7
Johnson B, Claxton D, Stanford B, et al (2006) Development of a composite bendable-wing micro air vehicle. J Am Inst Aeronaut Astronaut 1–16
Sutton MA, Turner JL, Bruck HA, Chae TA (1991) Full-field representation of discretely sampled surface deformation for displacement and strain analysis. Exp Mech 31:168–177
Sutton MA (2008) Springer handbook of experimental solid mechanics. 565–600
Sutton MA, Orteu JJ, Schreier H (2009) Image correlation for shape, motion and deformation measurements: basic concepts, theory and applications. 342
Abudaram YJ, Ifju PG, Hubner JP, Ukeiley L (2013) Controlling pretension of silicone membranes on micro air vehicle wings. J Strain Anal Eng Des 49:161–170. doi:10.1177/0309324713490926
Yamamoto N, Gdoutos E, Toda R et al (2014) Thin films with ultra-low thermal expansion. Adv Mater 26:3076–3080. doi:10.1002/adma.201304997
Gdoutos E, Shapiro AA, Daraio C (2013) Thin and thermally stable periodic metastructures. Exp Mech 53:1735–1742. doi:10.1007/s11340-013-9748-z
Author information
Authors and Affiliations
Corresponding author
Rights and permissions
About this article
Cite this article
Cantrell, J.T., Ifju, P.G. Experimental Characterization of Unimorph Shape Memory Polymer Actuators Incorporating Transverse Curvature in the Substrate. Exp Mech 55, 1395–1409 (2015). https://doi.org/10.1007/s11340-015-0035-z
Received:
Accepted:
Published:
Issue Date:
DOI: https://doi.org/10.1007/s11340-015-0035-z