Abstract
Brassinosteroids (BR) are plant steroid hormones regulating various aspects of morphogenesis, such as seed development and germination, cell division and elongation, differentiation of tracheary elements, development during growth in darkness (skotomorphogenesis), photosynthesis and response to environmental stress. Brassinosteroid synthesis has been studied to a great extent in the dicot species, Arabidopsis thaliana, resulting in the identification of genes participating in this process. Much less is known about BR synthesis in crops, including the monocots. The purpose of this study was to identify and characterize barley coding sequence HvDWARF involved in brassinosteroid synthesis. This sequence, encoding brassinosteroid-6-oxidase, was identified on the basis of barley ESTs. This sequence was screened for nucleotide substitutions in semi-dwarf, chemically-induced barley mutants exhibiting changes in etiolation. The responsiveness of these genotypes to exogenous brassinosteroids was determined with the use of leaf-blade segment unrolling tests. The semi-dwarf phenotype of the BR-deficient mutants was rescued by the application of 10−5 M 24-epi-brassinolide. Two missense mutations were identified within the HvDWARF sequence in BR-deficient mutants 522DK and 527DK from variety ‘Delisa’. These substitutions cause changes of amino acid residues located within the conserved fragments of the encoded polypeptide. The transcription profile of HvDWARF and HvBAK1/SERK3, involved in BR signaling, was determined during the early stages of seedling development in BR-deficient and BR-insensitive mutants using real-time quantitative PCR. This analysis indicated that HvDWARF displays a uniformly low level of this process, whereas the transcription level of HvBAK1 proved to be spatially and temporally regulated.
Similar content being viewed by others
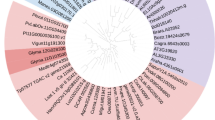
Introduction
Brassinosteroids (BR) are steroidal hormones displaying high activity in stimulation of various aspects of plant morphogenesis, such as seed development and germination, cell division and elongation, differentiation of tracheary elements, development during growth in the darkness (skotomorphogenesis), photosynthesis and response to environmental stress. They are present in pollen grains, anthers, seeds and vegetative tissues undergoing early developmental stages in a broad range of species representing various evolutionary groups. The richest sources of brassinosteroids are pollen grains and immature seeds, whereas mature leaves and stems contain a much lower concentration of these hormones (Bajguz and Tretyn 2003; Clouse and Sasse 1998; Fujioka and Yokota 2003). Brassinosteroid synthesis and signal transduction pathways have been studied to the greatest extent in the dicot species, Arabidopsis thaliana, resulting in the identification of genes participating in these two processes. The synthesis of brassinosteroids comprises several steps leading from episterol to brassinolide and is divided into two parallel pathways: early- and late C-6 oxidation (Shimada et al. 2001) (Fig. 1). Brassinolide, which is the most biologically active form of brassinosteroids (Yokota 1997), was not identified in monocotyledonous plant species including mutants that displayed defects in BR signaling in rice (Nakamura et al. 2006; Sakamoto and Matsuoka 2006; Yamamuro et al. 2000) and barley (Chono et al. 2003). In rice BR-deficient mutants with defects in synthesis of this hormone brassinolide was not identified either (Hong et al. 2002; Hong et al. 2003; Hong et al. 2005; Mori et al. 2002; Tanabe et al. 2005). In this species castasterone is a compound that is bound by a transmembrane receptor and triggers BR signaling pathway (Bishop and Koncz 2002; Sakamoto and Matsuoka 2006). In rice OsDWARF encoding BR-6-oxidase (CYP85A, Cytochrome P450 85A) is a single-copy gene and it was demonstrated that this enzyme does not catalyze the synthesis of brassinolide, and in this species BR synthesis is terminated at castasterone (Kim et al. 2008). On the other hand, in A. thaliana two genes encoding CYP85A enzymes were identified. In this species, the 6-deoxocastasterone, which is a product of late C-6 oxidation pathway, is converted to brassinolide in a two-step reaction catalyzed by oxidases encoded by two paralogous genes, BR6ox1 (BR-6-oxidase1) and BR6ox2 (BR-6-oxidase2). Gene duplication is suggested as the way for the emergence of these genes, especially that transposons were identified in close vicinity, which could have caused gene duplication via recombination. These enzymes are also responsible for the conversion of 6-deoxotyphasterol to typhasterol, 6-deoxo-3-dehydroteasterone to 3-dehydroteasterone, and 6-deoxoteasterone to teasterone, which makes the two parallel synthesis pathways converge not only on the last step, the synthesis of castasterone, but also on the previous ones (Bishop et al. 1999; Fujioka et al. 2002; Noguchi et al. 2000; Shimada et al. 2001). Both enzymes BR6ox1 (CYP85A1) and BR6ox2 (CYP85A2) catalyze the conversion of 6-deoxocastasterone to castasterone, however, the reaction of brassinolide synthesis from castasterone, known as 7-oxalactonization, is performed by the second one only (Kim et al. 2005). The reactions catalyzed by these two oxidases are known to be rate-limiting steps, determining the level of brassinosteroid synthesis (Nomura et al. 2001). In Lycopersicon esculentum, two genes, LeBR6ox1 and LeBR6ox3, encoding enzymes CYP85A1 and CYP85A3, respectively, catalyzing the same reactions as CYP85A1 and CYP85A2 in Arabidopsis were identified. However, the knockout mutation in LeBR6ox1 gene results in the dwarf phenotype and de-etiolation. This is caused by the fact that LeBR6ox3, encoding CYP85A3 oxidase catalyzing brassinolide synthesis, is expressed only in fruits, and as a consequence, there is no functional complementation between these enzymes in vegetative tissues, whereas castasterone, synthesized by CYP85A1 is the most physiologically active form of brassinosteroids (Nomura et al. 2005). In monocots the homologous DWARF gene, encoding BR-6-oxidase, has been identified and characterized so far only in Oryza sativa (Hong et al. 2002, 2005; Kim et al. 2005; Mori et al. 2002), however, maize full-length cDNA clone with homology to rice OsDWARF was identified recently (Kim et al. 2008).
In this paper the identification and functional analysis of barley coding sequence HvDWARF involved in BR synthesis is reported, along with the determination of transcription profile during early stages of seedling development.
Materials and methods
Plant material
Plant material used in this study included semi-dwarf barley mutants from the collection of the Department of Genetics, University of Silesia. These mutants were obtained by mutagenic treatment of barley grains with MNU (N-methyl-N-nitrosourea). Treatment was carried out twice for 3 h with the use of 0.7 mM MNU/treatment and with 6-h inter-incubation germination period. Barley seeds were pre-soaked in distilled water for 8 h and mutagenically treated with MNU for 3 h. After the first treatment the seeds were rinsed several times in tap water and germinated on trays with filter paper for 6 h before the second 3-h treatment with mutagen. Double treatment with inter-incubation germination period between the treatments was performed according to the protocol proposed by Maluszynska and Maluszynski (1983). The analyzed group of genotypes included semi-dwarf mutants displaying defects in BR metabolism: 667Q, 703Q, 749Q, 821Q, 822Q, 823Q, 837Q, 933Q from breeding line ‘Q448’; 522DK, 527DK, 579DK, 586DK, 587DK, 588DK, 617DK from variety ‘Delisa’; 006AR, 026AR from variety ‘Aramir’ and mutants 219DV, 224DV, 226DV and 239DV derived from variety ‘Diva’. The height of plants of semi-dwarf genotypes, which was measured in a two-year field experiment, in which 30 plants per genotype/year were analyzed, is given in Online resource 1. Genetic studies indicated allelism for semi-dwarf character of the following genotypes: 522DK and 527DK; 586DK and 588DK derived from variety ‘Delisa’ and mutants 821Q, 822Q and 823Q from breeding line ‘Q448’. In molecular analysis two allelic BR-deficient (522DK and 527DK) and one BR-insensitive (821Q) mutants were assayed and a detailed characteristic of these genotypes is given in Table 1. It was demonstrated that the analyzed phenotypes of these mutants are caused by single-gene recessive mutations. A comparison of phenotypes of mutants 522DK and 527DK carrying substitutions in HvDWARF coding sequence with the parental variety ‘Delisa’ is presented in Fig. 2. Both mutants show a similar reduction in plant height when compared to variety ‘Delisa’, 25 and 20% for 522DK and 527DK, respectively. Spikes of both mutants are of intermediate density with long awns (but shorter than in ‘Delisa’ variety).
Upper panel: Phenotypes at maturity of the semi-dwarf barley mutants 522DK (a) and 527DK (b) from variety ‘Delisa’ (c). Bar—10 cm. Lower panel: Length of seedlings of parental varieties and semi-dwarf mutants grown in light and darkness in etiolation test. Mean values are shown with bars representing SD
Physiological tests
Barley mutants were selected on the basis of etiolation test during which seedlings of each genotype were grown for 7 days in two different conditions: in light and in darkness (under black hoods covering the pots in order to eliminate the influence of light, which is known to reduce BR synthesis). After the 7-day growing period the length of seedlings was measured. Lack of enhanced elongation of seedlings during growth in darkness (when compared to those grown in light) indicated that the genotype displayed defects in etiolation.
In order to determine whether the phenotype of the analyzed mutants is caused by a malfunctioning of BR synthesis or the signaling pathways, a leaf segment unrolling test was performed according to the protocol by Chono et al. (2003). Three concentrations of 24-epi-brassinolide (Sigma) were applied: 10−8, 10−6 and 10−5 M and distilled water as a control. Leaf segments of parent varieties were analyzed as a reference. Ten leaf segments per genotype were analyzed for each solution. After the incubation period, the width of leaf blade segments was measured and compared. The test was repeated three times. In order to determine the stage of BR synthesis pathway, which is impaired in the characterized BR-deficient mutants 522DK and 527DK, the leaf-blade unrolling test with the use of teasterone and typhasterol (Chemiclones Inc., Waterloo, Ontario, Canada) as the intermediates of BR synthesis was performed. The BR-insensitive mutant 821Q was included in the test as a negative control. The test was conducted following the same procedure as described above and applying the same concentrations of intermediates within incubation solutions.
Morphological data as well as results of physiological experiments were analyzed statistically applying Student’s t test.
Identification of the HvDWARF sequence
The first step of this procedure was searching the NCBI GenBank database for sequences responsible for brassinosteroid synthesis derived from various plant species. These sequences and encoded polypeptides were utilized as queries to search barley EST databases: TIGR Plant Transcript Assemblies database (http://www.blast.jcvi.org/euk-blast/plantta_blast.cgi) and Computational Biology and Functional Genomics Laboratory database (http://compbio.dfci.harvard.edu/tgi/cgi-bin/tgi/Blast/index.cgi) for EST sequences sharing highest levels of similarity, which were used as the basis for PCR primers design. In order to clone barley coding sequences HvDWARF the following primers were used: DW1F 5′ CACACTTGTTGCCACCAGCTC 3′, DW1R 5′ CGCATGAAGGCGTCCATCT 3′, DW2F 5′ CTCCGGCAAAGCCTCCTC 3′, DW2R 5′ TCCAGCCTTTTGGAATGACC 3′, DW3F 5′ CATTCCAAAAGGCTGGAGAAT 3′, DW3R 5′ CCTACTTCTATCCCTATGTGCTTTTT 3′.
RNA extraction and reverse transcription
RNA was extracted from barley seedlings of the above mentioned genotypes (roots and leaves were combined) which had been grown for 7 days in aeroponic conditions. The extraction procedure was performed according to the protocol for TriPure Isolation Reagent (Roche). The concentration and purity of the extracted RNA was measured with the use of Spectrophotometer ND-1000 (NanoDrop). Before reverse transcriptions were performed according to RevertAid First Strand cDNA Synthesis Kit protocol (Fermentas), 3 μg of each RNA isolate (500 ng/μl) was subjected to treatment with RNase-free DNase (Promega). Each reverse transcription reaction was performed on the basis of 3 μl (300 ng/μl) of DNase-treated RNA solution. On the basis of the obtained cDNA RT-PCR, reactions were carried out with the use of the above mentioned primers. For each analyzed genotype and distinct primer’s pair four independent PCR reactions were performed in order to exclude mutations introduced by DNA polymerase during DNA synthesis. The reactions were performed in Biometra® thermocyclers.
Cloning and sequencing
PCR products were extracted from 1.5% agarose gels upon electrophoresis according to QIAEX II Agarose Gel Extraction Kit Protocol (QIAGEN). Purified extraction products were ligated with pGEM-T Easy Vectors (Promega) according to protocol. To improve ligation efficiency, every time 3 μl of extraction products were used for ligation with plasmid. Transformations of E. coli high efficiency competent cells JM109 were carried out according to pGEM-T Easy Vector System Cloning Protocol (Promega). Positively transformed (white) colonies were selected upon growth overnight at 37°C on Luria–Bertani plates with ampicillin containing 40 μl of Blue-White Select Screening Reagent (Sigma). For each PCR product three white colonies were transferred to liquid LB medium with ampicillin. Plasmid isolations were carried out according to QIAprep Spin Miniprep Kit protocol (QIAGEN). Sequencing was carried out for all three clones derived from each PCR product. Inserts were sequenced according to Sequi-Therm EXCEL™ II DNA Sequencing Kit—LC protocol (EPICENTRE) using standard M13for and M13rev fluorescently-labeled primers (concentration 10 pM/μl). PAGE electrophoresis was performed with the use of LI-COR® automatic 4300 DNA analyzers. The obtained sequencing results were analyzed with the use of e-Seq V2.0 and AlignIR software (LI-COR), the latter one allowed the alignment of sequences derived from different genotypes in order to identify mutations.
Sequence analysis
The sequences carrying mutation(s) were subject to in silico translation in order to determine the influence of the identified substitution on the sequence of the encoded polypeptide. To determine the conservation level of the substituted amino acid residue, the sequences of homologous polypeptides were aligned with the use of BoxShade (http://www.ch.embnet.org/software/BOX_form.html). The amino acid residues were further characterized using PSIPRED Protein Structure Prediction program (http://www.bioinf.cs.ucl.ac.uk/psipred/).
Real-time quantitative PCR (RT-qPCR)
The starting point of this study was to design PCR primers, which enabled amplification of short fragments of identified sequences HvDWARF [GenBank: DQ832258], HvBAK1/SERK3 [GenBank: EF216861] and HvCPD [GenBank: EU514492] as well as a fragment of HvG3PD gene [GenBank: X60343] encoding glyceraldehyde-3-phosphate dehydrogenase, which is constitutively and uniformly expressed and was used as a reference gene. The following primer pairs were used: Q-DWF 5′ CATCTCCTTGCCCATCAATC 3′, Q-DWR 5′ ATCGAAGTCGTGGACATGGT 3′, Q-BAKF 5′ TGCTGCCTTGCTATTTGCTA 3′, Q-BAKR 5′ CTTGCCAAATCCACCTCTTC 3′, Q-CPDF 5′ TCCATTCCCTCACCCTCA 3′, Q-CPDR 5′ GTATTCACAGCGGAGGCTCT 3′ and Q-G3PDF 5′ TCAAGCAAGGACTGGAGAGG 3′, Q-G3PDR 5′ ACACATCCACAGTGGGAACC 3′. The next step was RNA extraction from immature barley grains of variety ‘Delisa’ and BR-deficient mutants 522DK, 527DK and BR-insensitive 821Q. RNA was also extracted separately from leaves and roots of these genotypes during early stages of development on second and ninth day after germination. Its purpose was to determine the spatial and temporal transcription profile of the analyzed sequences. RNA was extracted in three biological repetitions as described above. Before reverse transcriptions, 1 μg of each RNA isolate (500 ng/μl) was subjected to treatment with RNase-free DNase (Promega). Each reverse transcription reaction was performed on the basis of 6 μl of DNase-treated RNA solution (double volume). Determination of transcription profiles using RT-qPCR strategy was carried out using LightCycler 2.0 instrument (Roche). Before analysis PCR conditions were optimized and specific amplification products were obtained for each primer pair. The following qPCR amplification profile was used: preliminary denaturation—95°C, 10 min; denaturation—95°C, 10 s; annealing temperature specific for each primer pair—8 s; elongation—72°C, 12 s; melting curve analysis—65°C, 15 s, do 95°C (0,1°C/s). Steps 2–4 were repeated 45 times. The following annealing temperatures were applied: Q-G3PDF, R—56°C; Q-DWF, R—58°C; Q-BAKF, R and QCPDF, R—57°C.
RT-qPCR reactions were performed with the use of LightCycler® FastStart DNA Master SYBR Green I Kit (Roche) and LightCycler® Capillaries 20 μl (Roche). Each reaction was technically repeated. The mixture for each reaction included: H2O (PCR-grade) 6.6 μl, MgCl2 (25 mM) 0.4 μl, Primer F (10 pM/μl) 0.5 μl, Primer R (10 pM/μl) 0.5 μl, Master Mix (including LightCycler®Fast-Start Enzyme) 1 μl, cDNA 1 μl (1,200 ng/μl). For each sequence the reference curve was analyzed in order to determine the reaction’s efficiency. Analysis of relative transcription levels of the identified sequences was performed using LightCycler Software V4.0 (Roche).
Results
Physiological classification of mutants
All the analyzed semi-dwarf mutants were classified as forms displaying defects in BR metabolism as they showed de-etiolation during growth in darkness, their length was similar to those seedlings which were grown in light, whereas seedlings of parental varieties showed an enhanced growth rate in darkness (Fig. 2). In order to characterize the type of abnormalities in BR metabolism, a leaf segment unrolling test was carried out. A positive reaction to 24-epi-brassinolide (24-eBL) was exhibited as gradual unrolling of leaf blade segments in relation to the increase in concentration of 24-eBL (10−8, 10−6, and 10−5 M) in incubation solution. Mutants 522DK, 527DK, 579DK, 586DK, 587DK, 588DK and 617DK from variety ‘Delisa’, 006AR, 026AR from variety ‘Aramir’, 224DV, 226DV and 239DV from variety ‘Diva’ as well as mutants 667Q, 703Q and 749Q from breeding line ‘Q448’ showed this reaction (Fig. 3a–c). This result indicated that these genotypes are BR-sensitive and their phenotypes are caused by defects in the process of brassinosteroid biosynthesis. On the other hand, mutant 219DV from variety ‘Diva’ and mutants 821Q, 822Q, 823Q, 837Q and 933Q from breeding line ‘Q448’ in the leaf blade segment unrolling test did not display any reaction to the applied concentrations of 24-eBL (Fig. 3b, c). This result implied that these genotypes are BR-insensitive and their phenotypes are caused by the malfunctioning of BR signal transduction pathway. Results of the leaf segment unrolling test are illustrated in Online resources 2 and 3.
Width of leaf blades of semi-dwarf mutants derived from varieties ‘Delisa’ (a) ‘Aramir’ and ‘Diva’ (b) and breeding line ‘Q448’ (c) incubated in increasing concentrations of 24-eBL. Length of seedlings treated with 24-eBL (10−5 M) during a two-week growth period of BR-feeding experiment (d). Mean values are shown with bars representing SD
To confirm that BR-sensitive genotypes respond to brassinosteroids and their phenotypes may be rescued by exogenous application of BR, seedlings of these genotypes were treated during a two-week growth period with the same 24-eBL concentrations which were used during the leaf segment unrolling test. After a two-week growth period, plants of all the BR-sensitive genotypes treated with the highest concentration of 24-eBL (10−5 M) were of similar height as the parental varieties (Fig. 3d). Similarly to the BR-sensitive genotypes, the BR-insensitive mutants were also treated with exogenous 24-eBL during a two-week growth period. However, in this case even high concentrations of brassinolide did not cause changes in the growth rate of these genotypes (Fig. 3d).
It was also analyzed whether the mutants display defects in gibberellic acid (GA) biosynthesis by the application of GA (at concentrations 10−9, 10−6, and 10−3 M) during 2 weeks of seedlings growth. All the genotypes responded to the applied concentrations of GA similarly to the wild type (Online resource 4), therefore, it was concluded that they have no abnormalities in GA biosynthesis or signaling. The above mutants’ classification constituted the basis for further analysis of HvDWARF coding sequence responsible for BR synthesis, which was identified during the study, in the group of BR-deficient mutants.
Identification of barley HvDWARF coding sequence and its functional analysis
The identification of HvDWARF coding sequence was based on barley ESTs derived from TIGR Plant Transcript Assemblies database and Computational Biology and Functional Genomics Laboratory database (websites’ addresses are given in Materials and methods). The first step of the identification and analysis of HvDWARF coding sequence was a retrieval from GenBank database of the rice OsDWARF mRNA sequence [GenBank: AB084385] together with the sequence of encoded BR-6-oxidase [GenBank: BAC45000]. These sequences were used as queries to search against the above mentioned databases with the use of BLASTN and TBLASTN programs, respectively, to retrieve barley EST sequences sharing the highest level of similarity. Default settings were used for BLAST screens. The barley EST sequences CB883392 (591 bp) and BQ461420 (635 bp) shared the highest identity with rice OsDWARF mRNA reaching 78 and 83% with the Expect values e−56 and e−30, respectively. These EST sequences covered the 5′ and 3′ ends of OsDWARF mRNA, respectively, and were used as a basis for designing the PCR primers which allowed the amplification and identification of full-length barley coding sequence HvDWARF together with 5′ and 3′ UTR sequences. The total length of the identified sequence was 1,724 bp, while the coding sequence (CDS) spanned 1,419 bp. The identified sequence together with the amino acid sequence of the encoded polypeptide, which was determined on the basis of in silico translation, were deposited in database under accession numbers [GenBank: DQ832258 and ABH01181], respectively. Sequences OsDWARF and HvDWARF share an 83% similarity with the Expect value E = 0.0 which was determined with the use of bl2seq.
The molecular weight of HvDWARF polypeptide, 472 amino acids in length, was determined as 53,389 Da with the use of Protein Molecular Weight program (http://www.bioinformatics.org/sms/prot_mw). The amino acid sequence of the polypeptide shares an 84% identity with the OsDWARF protein, with similarity reaching 90% and Expect value E = 0.0. The fragment of HvDWARF protein comprising residues from 40 to 459 is occupied by P450 cytochrome domain (pfam00067), which binds haem prosthetic group, being responsible for performing the redox reactions.
Taking into account the expected function of HvDWARF protein as BR-6-oxidase participating in the last step of brassinosteroid biosynthesis, the identified sequence encoding this polypeptide was analyzed in the group of mutants displaying defects in BR synthesis. In two allelic, BR-deficient, mutants 522DK and 527DK from variety ‘Delisa’ missense substitutions were identified in different fragments of HvDWARF sequence. In the case of 522DK mutant, G>A substitution was identified at position 1130, which causes the change of valine-341 residue to isoleucine. With the use of program BoxShade v.3.2 it was shown that the substituted valine-341 is a highly conserved residue present is analogous position in homologous DWARF polypeptides from barley, rice, Arabidopsis and tomato (Online resource 5).
In mutant 527DK, two substitutions located within the distal part of HvDWARF sequence were identified: first, A>G substitution at position 1434 causes change of glutamine-442 residue to arginine, while the second mutation C>T at position 1507 proved to be a silent-type substitution. Substitution A>G at position 1434 is located in the fragment of the sequence encoding P450 cytochrome domain, while the second one is located outside this region. With the use of BoxShade program, it was demonstrated that glutamine-442 is located within a highly conserved fragment of HvDWARF polypeptide within the P450 cytochrome domain in a close vicinity of amino acid residues responsible for the direct binding of haem prosthetic group (Online resource 5).
An analysis was carried out in order to characterize the identified HvDWARF protein sequence and to corroborate the significant role of amino acid residues, substituted in both allelic mutants, with the use of PSIPRED program v.3.0, which enables the localization of amino acid residues according to topology of secondary, spatial domains through the superimposition of secondary structure of the protein on its sequence (Bryson et al. 2005). Default settings of PSIPRED were used for the analysis. It was shown that both amino acid residues, which were substituted in mutants 522DK and 527DK, are located in the regions of HvDWARF polypeptide participating in the formation of secondary, spatial domains. Valine-341 is located within the alpha helix domain which encompasses amino acid residues at positions 333 to 344. Glutamine-442 is the last residue in the alpha helical domain, which is formed by residues located at positions 425 to 442 at the junction with beta-sheet domain spanning the residues at positions 443 to 447. It was shown with the use of PSIPRED program that the substitutions identified in mutants 522DK and 527DK lead to alterations in the arrangement of secondary domains. When compared to the arrangement of structural domains in wild type HvDWARF polypeptide, the protein forms in both mutants show distinct pattern of domains’ location. The entire amino acid sequences of HvDWARF polypeptides were analyzed, however, protein fragments carrying amino acid substitutions, identified in both allelic mutants, are shown in (Online resource 6).
Analysis of transcription profile of HvDWARF, HvBAK1/SERK3 and HvCPD sequences
A similar approach which had led to the identification of HvDWARF has been applied to identify barley sequence HvBAK1/SERK3 being highly similar to rice and Arabidopsis BAK1 genes encoding a component of BR receptor and barley HvCPD sequence encoding brassinosteroid C-23 hydroxylase. Transcription levels of these sequences were determined in barley seeds and during early stages of seedling development in BR-deficient and BR-insensitive mutants. The genotypes used in this study included variety ‘Delisa’ as a reference, BR-deficient mutants 522DK and 527DK from variety ‘Delisa’ and BR-insensitive mutant 821Q from breeding line ‘Q448’ (second reference genotype). The analysis indicated that transcription level of HvDWARF sequence, related to BR biosynthesis, is uniformly low in both leaves and roots, irrespective of the analyzed genotype or the stage of seedling development. The transcription level of this sequence proved to be only slightly elevated in analyzed mutants when compared to variety ‘Delisa’ and breeding line ‘Q448’. In all mutants the HvDWARF transcription level proved to be very similar (Fig. 4a).
The transcription level of HvDWARF sequence in variety ‘Delisa’, BR-deficient mutants 522DK, 527DK, breeding line ‘Q448’ and BR-insensitive mutant 821Q (a). The transcription level of HvBAK1/SERK3 sequence in variety ‘Delisa’, BR-deficient mutants 522DK, 527DK, breeding line ‘Q448’ and BR-insensitive mutant 821Q (b). Changes of transcription level of HvDWARF gene in response to exogenous 24-eBL in variety ‘Delisa’ (top), and BR-deficient mutant 522DK (middle) and 527DK (bottom) (c). Changes of transcription level of HvBAK1 gene in response to exogenous 24-epi-brassinolide in variety ‘Delisa’ (top), and BR-deficient mutant 522DK (middle) and 527DK (bottom) (d). Transcription profile of HvCPD gene in BR-deficient mutants 522DK and 527DK from variety ‘Delisa’ and BR-insensitive mutant 821Q from breeding line ‘Q448’ (e). Transcription level of reference gene HvG3PD is equal to 1.0. Mean values of three replicates are shown with bars representing SD
The transcription profile of sequence HvBAK1/SERK3 showed a common pattern in all the analyzed genotypes: it displayed fluctuations during seedling development, however, at each stage it proved to be higher in roots than in leaves. In both organs, as the seedling development proceeded, the transcription level of this sequence was significantly reduced until the ninth day upon germination when the analysis was completed. Transcription level of this sequence in seeds was of intermediate value, comparable with the transcription level in leaves on the second day after germination, however, significantly lower than transcription level in roots at the same time after germination (Fig. 4b).
In the case of mutants 522DK and 527DK, whose phenotype is caused by a defect in BR biosynthesis, the overall profile of changes in HvBAK1/SERK3 transcription during seedling development was maintained. However, its level at each stage was significantly and proportionally reduced when compared to the reference genotype, variety ‘Delisa’ (Fig. 4b). In the BR-insensitive mutant 821Q profile of HvBAK1/SERK3 transcription at each of the analyzed time-points was comparable to the analogous values that were noted in variety ‘Delisa’ and breeding line ‘Q448’ (Fig. 4b).
In order to confirm that the identified HvDWARF and HvBAK1 genes are involved in BR synthesis and signaling, respectively, influence of exogenously applied 24-eBL on their transcription profiles was determined. In the experiment two different 24-eBL concentrations were applied: 10−7 and 10−6 M. Transcription level of HvDWARF gene in variety ‘Delisa’ and both BR-deficient mutants was gradually reduced correspondingly to the increase in BR concentration. This tendency was particularly prominent in roots of these genotypes, which proved to be significantly more sensitive to higher concentration of the hormone (10−6 M) (Fig. 4c). The BR-insensitive mutant 821Q, as expected, did not show any response to the applied concentrations of the hormone with respect to the transcription profile of HvDWARF gene (data not shown).
As far as the influence of exogenously applied 24-eBL on HvBAK1 gene transcription profile is concerned, the previously described pattern of developmental down-regulation of the transcription was maintained in all assayed genotypes. In both BR-deficient mutants transcription level of HvBAK gene was significantly and proportionally reduced in all analyzed organs and time-points with respect to the corresponding values in parental variety ‘Delisa’. Similarly as in the case of HvDWARF gene, the applied concentrations of BR negatively regulated the transcription of HvBAK1 in a dose-dependent manner. Transcription level of HvBAK gene in variety ‘Delisa’ and both BR-deficient mutants was gradually reduced correspondingly to the increase in 24-eBL concentration. Similarly to HvDWARF transcription profile this tendency was particularly prominent in roots of these genotypes, which proved to be significantly more sensitive to higher concentration of the hormone (Fig. 4d). Also in this case the BR-insensitive mutant 821Q did not show any response to the applied concentrations of the hormone with respect to the transcription profile of HvBAK1 gene (data not shown).
Transcription of the identified HvCPD gene was also analyzed in order to check whether the identified missense mutations in the two BR-deficient mutants 522DK and 527DK have any influence on its level. The homologous CPD genes in other species are known to belong to the pivotal factors regulating BR homeostasis. In variety ‘Delisa’ and breeding line ‘Q448’ HvCPD transcription was at comparable level to HvDWARF, and similarly to the latter proved to be slightly higher in the leaves than in the roots. However, transcription level of HvCPD gene was significantly, about two times higher in BR-deficient mutants (522DK and 527DK) as well as BR-insensitive mutant 821Q in all examined organs when compared to corresponding parental lines (Fig. 4e).
Influence of BR-synthesis intermediates on unrolling of leaf-blades of BR-deficient mutants
In order to confirm with the use of physiological approach that the phenotype of two allelic BR-deficient mutants 522DK and 527DK is caused by defect in the function of BR-6-oxidase, catalyzing the last step in BR synthesis pathway, the unrolling test were performed applying two BR synthesis intermediates: teasterone and typhasterol. The tests were carried out on the two BR-deficient mutants, their parental variety ‘Delisa’, on BR-insensitive mutant 821Q and its parental breeding line ‘Q448’. The BR-insensitive mutant was assayed in the test as the negative control. Teasterone caused only slight reaction of both BR-deficient mutants even at very high concentration (10−5 M). This compound led to gradual unrolling of leaf blades of both parental varieties (‘Delisa’ and ‘Q448’), however, in both cases the unrolling was not complete. As expected, BR-insensitive mutant 821Q did not show any response to the applied concentrations of teasterone (Fig. 5a).
Width of leaf blades of BR-deficient mutants 522DK and 527DK from variety ‘Delisa’ and BR-insensitive mutant 821Q from breeding line ‘Q448’ incubated in increasing concentrations of teasterone (a). Width of leaf blades of BR-deficient mutants 522DK and 527DK from variety ‘Delisa’ and BR-insensitive mutant 821Q from breeding line ‘Q448’ incubated in increasing concentrations of typhasterol (b). Mean values are shown with bars representing SD
Typhasterol (direct precursor of castasterone) evoked more pronounced effect in unrolling of leaf blades of BR-deficient plants, especially at the highest concentration. However, it should be stressed that even at this concentration the unrolling was not complete as it was when 24-eBL was applied. Additionally, even at the highest concentration (10−5 M) the leaf blades of both BR-deficient mutants were significantly less unrolled when compared with the leaf blades of parental varieties. This compound led to gradual unrolling of leaf-blades of both parental varieties. However, as previously the unrolling was not complete. BR-insensitive mutant 821Q did not show any response to the applied concentrations of typhasterol (Fig. 5b).
Discussion
Effects of substitutions identified within barley sequence HvDWARF in allelic mutants 522DK and 527DK and in homologous genes
Allelic mutants 522DK and 527DK from variety ‘Delisa’ in the applied physiological tests displayed positive reactions to 24-eBL, which indicated that these mutants carry defects in BR biosynthesis. A semi-dwarf phenotype and the lack of drastic developmental abnormalities, which are typical for mutants displaying defects at early stages of BR synthesis, implied that the mutated gene encodes the enzyme catalyzing one of the late steps in this process (Choe et al. 1999, 2000; Lindsey et al. 2003; Schaller 2003). Up to now, there has been no barley sequence responsible for BR synthesis described in the scientific literature (data from NCBI database). Bioinformatic analyses and experimental studies allowed cloning and identification of complete barley coding sequence HvDWARF. Identity shared between the identified sequence and rice OsDWARF mRNA was 83% with the Expect value E = 0.0. This Expect value indicates that the homologous sequence was identified (Mori et al. 2002). The sequence of polypeptide encoded by identified HvDWARF sequence shared an 84% identity and a 90% similarity with rice OsDWARF protein. As previously, the Expect value was E = 0.0. This result suggests that the identified protein HvDWARF and rice enzyme OsDWARF have the same function in BR synthesis, as in A. thaliana the identity of two functionally active BR-6-oxidases AtBR6ox1 (CYP85A1) and AtBR6ox2 (CYP85A2) encoded by genes duplicated in the genome through recombination of vicinal transposons, reached 83% with 92% of sequence similarity (Castle et al. 2005; Kim et al. 2005). As it was demonstrated in this study, homologous proteins HvDWARF and OsDWARF are of similar length, 472 and 469, respectively, and similar molecular mass, 53,389 Da and 52,822 Da, respectively, (http://expasy.org/sprot). Additional confirmation that these proteins have the same function is that their analogous fragments are occupied by P450 cytochrome domain (pfam00067), binding haem prosthetic group, and participating in redox reactions that occur during castasterone synthesis (Hong et al. 2002; Mori et al. 2002).
The function of the identified coding sequence HvDWARF and its encoded polypeptide was validated by the identification of missense substitutions that caused changes of conserved amino acid residues in two allelic, semi-dwarf mutants, 522DK and 527DK. Both residues are located within highly conserved fragments of HvDWARF polypeptide. Valine-341 is situated within the Glu-x-x-Arg domain, overlapping with one of the substrate-recognition sites (SRSs) and within helix K, present in the analogous positions in various polypeptides belonging to the family of P450 cytochromes in plants and animals (including mammals), which indicates that this residue plays a crucial role in the functioning of the enzyme (Szekeres et al. 1996; Werck-Reichhart and Feyereisen 2000; Williams et al. 2004; Kim et al. 2005; Marchler-Bauer et al. 2007). In order to additionally confirm the role of this residue, its location with regard to the secondary spatial domains was determined with the use of PSIPRED program. This amino acid residue is situated within the alpha-helical domain which encompasses the residues at positions 333–344. This result is congruent with literature data on arrangement of spatial domains within analogous fragments of other P450 cytochrome proteins, including highly conserved helix K. This helical domain is responsible for the stabilization of the central part of enzyme molecule (Werck-Reichhart and Feyereisen 2000; Mori et al. 2002). The influence of the substitution identified in the mutant 522DK on brassinosteroid metabolism was reflected by a significant reduction of the transcription level of HvBAK1/SERK3 sequence, demonstrated in this study with the use of RT-qPCR technique.
In the allelic mutant 527DK, two substitutions located within the distal part of HvDWARF sequence were identified. Missense mutation at position 1434 is also situated within the fragment encoding P450 cytochrome domain. An analysis with the use of BoxShade program indicated that the polypeptide region encoded by this fragment shares a high level of conservation with the homologous polypeptides from mono- and dicotyledonous species. This result indicates that the fragment of polypeptide, surrounding the substituted amino acid residue, plays a significant role in the function of HvDWARF polypeptide. Moreover, glutamine-442 is a residue located in a close vicinity downstream of FxxGxRxCxG domain (Phe-xx-Gly-x-Arg-x-Cys-x-Gly), situated in the distal part of the polypeptide, and is responsible for binding the haem prosthetic group (Mori et al. 2002; Shimada et al. 2003; Werck-Reichhart and Feyereisen 2000). This residue has hydrophilic properties and, by a substitution identified in mutant 527DK, is replaced by a basic, positively charged, arginine. Both amino acids have distinct values of isoelectric point, 5.65 for glutamine and 10.76 for arginine. This substitution within a fragment of polypeptide that has high level of conservation may cause a significant alteration in the function of HvDWARF polypeptide. The significant role of glutamine-442 in reference to the arrangement of secondary, spatial domains was determined with the use of PSIPRED program. This residue is the last one forming the alpha-helical domain, encompassing residues 425–442, which is followed by the beta-sheet domain spanning residues 443–447. The substitution of an amino acid residue is located at the junction of two spatial domains and may invoke significant changes in protein conformation. In this study it was shown with the use of bioinformatic tools that identified substitutions of amino acid residues in mutants 522DK and 527DK cause changes in the arrangement of secondary domains (Online resource 6) which is known to have an impact on the enzymatic efficiency of the protein. The presence of alpha-helical and beta-sheet spatial domains is reflected by a high level of conservation shared by the distal part of homologous protein sequences, encompassing fragments responsible for binding of the haem prosthetic group (Hong et al. 2002; Mori et al. 2002). Analysis of the arrangement of analogous, secondary, spatial domains in homologous DWARF proteins from A. thaliana, L. esculentum, O. sativa and H. vulgare performed in this study with the use of PSIPRED program, showed a high level of similarity, particularly in the distal parts of these polypeptides. Similarly to the presented results, it was reported that the alpha-helical domain in the close vicinity of the haem prosthetic group binding region is followed by several, tandem beta-sheets located in the distal part of the vast group of P450 cytochrome proteins (Werck-Reichhart and Feyereisen 2000). The high conservation of amino acid sequence as well as the arrangement of spatial domains in the distal part of P450 cytochrome proteins may be explained by the fact, that in this fragment not only the haem prosthetic group binding region but also the last of substrate-recognition sites are present (Szekeres et al. 1996; Werck-Reichhart and Feyereisen 2000; Williams et al. 2004; Kim et al. 2005). A similar arrangement of spatial, secondary domains was also determined in two other members of P450 cytochrome proteins, identified in our laboratory, HvCYP90D and HvCPD (data not shown). It indicates that these fragments of P450 proteins are of crucial importance for appropriate conformation of polypeptides and their enzymatic function (Werck-Reichhart and Feyereisen 2000). Therefore, substitution of glutamine-442 may result in a functional alteration of this enzyme, especially that this residue was substituted by arginine, characterized by different physical and chemical properties. As it was in the case of allelic mutant 522DK, the effect of the substitution identified in the mutant 527DK was reflected by a significant reduction of transcription level of HvBAK1/SERK3 sequence, demonstrated in this study with the use of RT-qPCR technique.
Mutations in homologous sequences DWARF (BR6ox) were identified also in other mono- and dicotyledonous species (Bishop et al. 1999; Hong et al. 2002; Hong et al. 2005; Jager et al. 2007; Kim et al. 2005; Mori et al. 2002). In rice, four different mutations have so far been identified within the OsDWARF sequence. Two mutants brd (brassinosteroid-dependent) and brd1-1 (brassinosteroid-deficient dwarf1) carried deletions within coding sequences of the gene that led to the formation of a truncated polypeptide OsDWARF and consequently, drastic alterations of plants’ phenotypes, dwarfism and morphological deformations associated with fertility reduction (Hong et al. 2002; Mori et al. 2002; Hong et al. 2005; Kim et al. 2005). The critical role of amino acid residue’s location within the conserved region of the polypeptide was illustrated by the results of a study on two other rice mutants that carried substitutions within OsDWARF sequence. It was shown that the same type of substitution of glycine residues located within the same domain (SRS1) may lead to various phenotypical results depending on the level of conservation of the residue and its position within the functional domain. The first of these substitutions (brd1-2) results in a change of glycine-111 to valine, while the second (brd1-3) leads to a replacement of glycine-101 by valine. Both residues are located within the substrate-recognition site. Glycine-101 is the first amino acid within this functional domain, whereas glycine-111 is situated in its central part (Kim et al. 2005). Glycine-111 is a highly conserved residue present at the analogous positions in various members of the cytochrome P450 protein family, whereas glycine-101 is conserved only among DWARF homologs. The difference in conservation level and position within the functional domain between these residues leads to alternative phenotypes of mutants: brd1-2 resembles mutants carrying deletions within OsDWARF coding sequence in terms of height reduction and morphological deformations, indicating that this residue plays a crucial role in the functioning of the encoded polypeptide. The second mutant (brd1-3) displays a semi-dwarf phenotype with a height reduction ca. 30% and without any abnormalities in leaf morphology (Hong et al. 2002, 2005).
Insertional mutation within A. thaliana AtCYP85A1/BR6ox1 gene had no effect on the phenotype, therefore the function of the encoded polypeptide was inferred on the basis of sequence similarity to DWARF C-6 oxidase from L. esculentum. Knock-out mutation in paralogous A. thaliana gene AtCYP85A2/BR6ox2 did not lead to any apparent alteration in the phenotype, either. However, simultaneous loss-of-function mutations in both of these genes in double mutant cyp85a1/cyp85a2 led to drastic dwarfism and sterility (Nomura et al. 2001; Shimada et al. 2001, 2003; Kim et al. 2005; Kwon et al. 2005). This result indicated that these paralogous genes have redundant functions (Bishop et al. 2006). These genes share a high level of similarity also in regulatory regions and the encoded polypeptides share an 83% identity. Both encoded enzymes catalyze C-6 oxidation, leading to the synthesis of castasterone. However, only CYP85A2 performs the Baeyer–Villiger reaction, known as 7-oxalactonization, leading to the synthesis of brassinolide (Castle et al. 2005; Kim et al. 2005). Results of this study corroborate the difference between the genomes of mono- and dicotyledonous species in terms of duplication of DWARF (BR6ox) genes. A functional analysis of Arabidopsis, tomato and pea genomes indicated that in each of them a duplication of genes encoding homologous CYP85 enzymes occurred. However, in rice genome analysis only one copy of functional gene encoding BR C-6 oxidase, catalyzing the synthesis of castasterone, was identified (Bishop et al. 2006). The fact that brassinolide was not extracted from monocotyledonous plant tissues, including BR-insensitive forms carrying mutations in homologous BRI1 genes from rice (Nakamura et al. 2006; Sakamoto and Matsuoka 2006; Yamamuro et al. 2000) and barley (Chono et al. 2003) seems to corroborate this conclusion. There was no brassinolide accumulation in rice mutants displaying defects in brassinosteroid synthesis, either (Hong et al. 2002; Mori et al. 2002; Hong et al. 2003; Hong et al. 2005; Tanabe et al. 2005). It was also demonstrated that in this species castasterone is the compound that interacts directly with the BRI1 receptor, initiating the BR signaling (Bishop and Koncz 2002; Sakamoto and Matsuoka 2006). On the contrary, brassinolide was accumulated in Arabidopsis mutants showing defects in BR signaling (Friedrichsen and Chory 2001; Shimada et al. 2001). Lack of a paralogous sequence performing redundant function in BR synthesis explains the impact of mutations identified in rice OsDWARF gene (Hong et al. 2002; Mori et al. 2002) and barley HvDWARF coding sequence (this study) on the plants’ phenotype.
The coding sequences HvDWARF and HvBAK1/SERK3 display different transcription profiles at early stages of barley seedling development
In the presented study the HvDWARF and HvBAK1/SERK3 sequences involved in BR synthesis and transduction pathway, respectively, showed distinct transcription profiles during barley seedling development. In the case of HvDWARF in all analyzed genotypes, including BR-deficient and BR-insensitive mutants, the transcription level was uniformly low, irrespective of organ or developmental stage. This indicates that a lowered level of BR synthesis conferred by substitutions identified within HvDWARF sequence is not compensated by the increase in transcription level of this gene. Low transcription level of homologous sequences from other species was also demonstrated (Bancos et al. 2006; Shimada et al. 2001, 2003). Additionally, in rice a low OsDWARF transcription level was noted in both leaves and roots (Nakamura et al. 2006). The transcripts of this gene could not be detected with the use of standard Northern blotting procedure (Bishop et al. 1999; Hong et al. 2003; Shimada et al. 2003). The transcription level of the homologous Arabidopsis gene analyzed with GUS construct was not detected either. As corroboration, fluorescence probe from CYP85A1 gene, utilized in microarray experiment, did not exceed the threshold level, remaining at the level of average background fluorescence (Castle et al. 2005). It was reported that reactions catalyzed by the enzymes encoded by these homologous genes constitute the rate-limiting step during BR biosynthesis (Nomura et al. 2001; Jager et al. 2007) and generally, genes encoding such enzymes show a low transcription level (Shimada et al. 2001; Shimada et al. 2003; Kim et al. 2006). Lack of significant increase in the transcription level of HvDWARF sequence in BR-insensitive mutant 821Q is corroborated by uniformly low transcription level of Arabidopsis AtCYP85A1/BR6ox1 homologous gene in mutants displaying defects in BR signaling: bri1-9 and bak1 (Nomura et al. 2001), indicating that abnormalities in BR perception are compensated at another stage of BR biosynthesis by a feedback mechanism.
The other sequence characterized in this study, HvBAK1/SERK3, displays a distinct spatial and temporal profile of transcription during barley seedling development: in leaves and roots the transcription of this sequence is down-regulated, however, at each of the time-points it remains significantly higher in roots than in leaves. Similarly, transcription of the homologous A. thaliana gene, AtBAK1/SERK3, proved to be higher in roots than in leaves. Taking into account that the encoded polypeptide forms a functional complex with BRI1 kinase, a temporal regulation of BAK1/SERK3 transcription is inferred on the basis of developmental changes in BRI1 transcription. It was observed that the transcription level of AtBRI1 gene is developmentally down-regulated both in leaves and roots which explains the reduced sensitivity of mature organs to exogenous BR (Friedrichsen et al. 2000; Russinova et al. 2004). Similarly to the results presented in this study, in P. sativum the transcription level of gene PsBRI1 was significantly higher in roots than in other organs, including immature seeds (Nomura et al. 2003). The transcription level of homologous rice gene OsBRI1 proved to be also very low in immature grains (Yamamuro et al. 2000). In the presented results, the transcription level of BR signaling-related HvBAK1/SERK3 sequence was relatively low in immature barley grains. In Arabidopsis, the synthesis of biologically active forms of BR in roots is very low. Significant differences in transcription level of genes involved in BR synthesis and metabolism are found between roots and leaves (Bancos et al. 2002; Müssig et al. 2003; Nakamura et al. 2006). Roots display an increased sensitivity to exogenous brassinosteroids. Concentrations appropriate for stimulation of shoot growth have an inhibitory effect on root elongation (Li et al. 2005). This effect is, at least partially, the result of an increased transcription level of genes encoding components of transmembrane BR receptor complex (Nomura et al. 2003). It was also noted that the concentration of terminal, most biologically active forms of BR, is reduced in roots compared to shoots and it is associated with the increased expression of a gene involved in BR catabolism through C-26 hydroxylation in roots (Neff et al. 1999; Turk et al. 2003; Symons and Reid 2004; Nakamura et al. 2005; Tanaka et al. 2005; Bancos et al. 2006). This situation results in an increased transcription of genes encoding the components of BR receptor in roots (Shimada et al. 2003). Another Arabidopsis gene responsible for BR inactivation, mediated through 23-O glycosylation, is UGT73C5 (UDP-Glycosyltransferase 73C5). Its expression is gradually down-regulated during plant development both in shoots and in roots (Poppenberger et al. 2005), which is associated with the developmental down-regulation of genes involved in BR perception (observed also in this study). This regulation is based on a feedback mechanism.
Barley mutants 522DK and 527DK, characterized in this study, displayed a different transcription profile of HvBAK1/SERK3 sequence when compared to variety ‘Delisa’: at the analyzed stages of seedling development and in organs assayed the level of transcription was significantly, but proportionally, reduced. Since the homeostasis of BR metabolism is maintained through a negative feedback mechanism this result indicates that in these mutants the compensation for diminished level of BR synthesis, evoked by the identified mutations, takes place at one of the earlier stages of BR synthesis, causing a decrease in the transcription of HvBAK1/SERK3 sequence. Similar results were observed in A. thaliana (Tanaka et al. 2005). The assumption that the compensation for defects in BR synthesis does not occur at the BR signaling level is further corroborated by the fact that no alteration in the transcription profile of HvBAK1/SERK3 sequence was observed in BR-insensitive mutant 821Q. The results of the presented study indicate that this compensation does not occur at the synthesis stage for which HvDWARF sequence is responsible either, as no alteration in its transcription profile was observed in analyzed mutants when compared to variety ‘Delisa’.
To confirm that both analyzed coding sequences, HvDWARF and HvBAK1, are related with BR synthesis and signaling, respectively, and are regulated by feedback mechanism experiments were carried out in which the influence of BR treatment on transcription of these genes was measured. Transcription level of both, HvDWARF gene (Fig. 4c) and HvBAK1 gene (Fig. 4d), was gradually decreased in BR dose-dependent manner. This effect in both genes was more pronounced in the roots when compared to leaves, what is congruent with the fact that root tissues are more sensitive to BRs than leaves. These results clearly indicate that the barley HvDWARF and HvBAK1 genes are regulated by feedback mechanism.
It is known that the compensation in BR homeostasis takes places at the stages catalyzed by enzymes encoded by homologs of DWARF4 and/or CPD (Constitutive Photomorphogenesis and Dwarfism) genes. In Arabidopsis both of them are responsible for maintaining the brassinosteroid homeostasis on the basis of a feedback mechanism (Mathur et al. 1998; Tanaka et al. 2005; Bancos et al. 2006; Kim et al. 2006). It was noted that an accumulation of DWARF4 transcripts occurs both in BR-deficient and BR-insensitive mutants (Choe et al. 2001; Noguchi et al. 2000). It was also observed that the expression of this gene is inhibited by the application of exogenous BR, indicating that it is a key player in the quantitative regulation of endogenous level of biologically active form of this hormone. The transcription level of DWARF4 gene is particularly low even when compared to other sequence regulating BR synthesis in the feedback manner as for example, CPD (Kim et al. 2006). Also, in rice it was observed that the transcription of homologous genes OsCPD1 and OsCPD2 in BR-insensitive and BR-deficient mutants was elevated. This indicates that also in monocots these genes regulate BR synthesis in response to alterations in the homeostasis of this hormone (Sakamoto and Matsuoka 2006). Therefore, in the presented paper the transcription profile of HvCPD gene was also analyzed in order to check whether the identified missense mutations in the two BR-deficient mutants 522DK and 527DK and BR-insensitivity of the mutant 821Q have any influence on its level. In all analyzed mutants (BR-deficient and -insensitive) transcription level of HvCPD gene was significantly elevated when compared to the parental varieties. This indicated that the HvCPD gene plays role in compensation of BR-related defects in these mutants. Therefore, this result is of great value in elucidating the BR homeostasis in barley.
The experiments involving unrolling of the leaf blades of BR-deficient mutants in response to the intermediates of BR synthesis (teasterone and typhasterol) showed, that these compounds are unable to restore the normal unrolling rate of leaf segments (as it was in the case of 24-eBL). It indicates that both compounds are located downstream the stage of BR synthesis pathway, which is impaired in BR-deficient mutants. Since typhasterol is the direct precursor of castasterone, which is a substrate of BR-6-oxidase encoded by HvDWARF gene, it confirms that the identified mutations in BR-deficient mutants are responsible for their phenotype.
References
Bajguz A, Tretyn A (2003) The chemical characteristic and distribution of brassinosteroids in plants. Phytochemistry 62:1027–1046
Bancos S, Nomura T, Sato T, Molnar G, Bishop GJ, Koncz C, Yokota T, Nagy F, Szekeres M (2002) Regulation of transcript levels of the Arabidopsis cytochrome P450 genes involved in brassinosteroid biosynthesis. Plant Physiol 130:504–513
Bancos S, Szatmari AM, Castle J, Kozma-Bognar L, Shibata K, Yokota T, Bishop GJ, Nagy F, Szekeres M (2006) Diurnal regulation of the brassinosteroid-biosynthetic CPD gene in Arabidopsis. Plant Physiol 141:299–309
Bishop GJ, Koncz C (2002) Brassinosteroids and plant steroid hormone signaling. Plant Cell 14(Suppl 1):97–110
Bishop GJ, Nomura T, Yokota T, Harrison K, Noguchi T, Fujioka S, Takatsuto S, Jones JDG, Kamiya Y (1999) The tomato DWARF enzyme catalyzes C-6 oxidation in brassinosteroid biosynthesis. Proc Natl Acad Sci USA 96:1761–1766
Bishop G, Nomura T, Yokota T, Montoya T, Castle J, Harrison K, Kushiro T, Kamiya Y, Yamaguchi S, Bancos S, Szatmàri AM, Szekeres M (2006) Dwarfism and cytochrome P450-mediated C-6 oxidation of plant steroid hormones. Biochem Soc Trans 34:1199–1201
Bryson K, McGuffin LJ, Marsden RL, Ward JJ, Sodhi JS, Jones DT (2005) Protein structure prediction servers at University College London. Nucl Acids Res 33(Web Server issue):W36–38
Castle J, Szekeres M, Jenkins G, Bishop GJ (2005) Unique and overlapping expression patterns of Arabidopsis CYP85 genes involved in brassinosteroid C-6 oxidation. Plant Mol Biol 57:129–140
Choe S, Noguchi T, Fujioka S, Takatsuto S, Tissier CP, Gregory BD, Ross AS, Tanaka A, Yoshida S, Tax FE, Feldmann KA (1999) The Arabidopsis dwf7/ste1 mutant is defective in the Δ7 sterol C-5 desaturation step leading to brassinosteroid biosynthesis. Plant Cell 11:207–221
Choe S, Tanaka A, Noguchi T, Fujioka S, Takatsuto S, Ross AS, Tax FE, Yoshida S, Feldmann KA (2000) Lesions in the sterol Δ7 reductase gene of Arabidopsis cause dwarfism due to a block in brassinosteroid biosynthesis. Plant J 21:431–443
Choe S, Fujioka S, Noguchi T, Takatsuto S, Yoshida S, Feldmann KA (2001) Overexpression of DWARF4 in the brassinosteroid biosynthethic pathway results in increased vegetative growth and seed yield in Arabidopsis. Plant J 26:573–582
Chono M, Honda I, Zeniya H, Yoneyama K, Saisho D, Takeda K, Takatsuto S, Hoshino T, Watanabe Y (2003) A semidwarf phenotype of barley uzu results from a nucleotide substitution in the gene encoding a putative brassinosteroid receptor. Plant Physiol 133:1209–1219
Clouse SD, Sasse JM (1998) Brassinosteroids: essential regulators of plant growth and development. Annu Rev Plant Physiol Plant Mol Biol 49:427–451
Friedrichsen DM, Chory J (2001) Steroid signaling in plants: from the cell surface to the nucleus. BioEssays 23:1028–1036
Friedrichsen DM, Joazeiro CAP, Li J, Hunter T, Chory J (2000) Brassinosteroid-insensitive1 is a ubiquitously expressed leucine-rich repeat receptor serine/threonine kinase. Plant Physiol 123:1247–1255
Fujioka S, Yokota T (2003) Biosynthesis and metabolism of brassinosteroids. Annu Rev Plant Biol 54:137–164
Fujioka S, Takatsuto S, Yoshida S (2002) An early C-22 oxidation branch in the brassinosteroid biosynthetic pathway. Plant Physiol 130:930–939
Hong Z, Ueguchi-Tanaka M, Shimizu-Sato S, Inukai Y, Fujioka S, Shimada Y, Takatsuto S, Agetsuma M, Yoshida S, Watanabe Y, Uozu S, Kitano H, Ashikari M, Matsuoka M (2002) Loss-of-function of a rice brassinosteroid biosynthethic enzyme, C-6 oxidase, prevents the organized arrangement and polar elongation of cells in the leaves and stem. Plant J 32:495–508
Hong Z, Ueguchi-Tanaka M, Umemura K, Uozu S, Fujioka S, Takatsuto S, Yoshida S, Ashikari M, Kitano H, Matsuoka M (2003) A rice brassinosteroid-deficient mutant, ebisu dwarf (d2) is caused by a loss of function of a new member of cytochrome P450. Plant Cell 15:2900–2910
Hong Z, Ueguchi-Tanaka M, Fujioka S, Takatsuto S, Yoshida S, Hasegawa Y, Ashikari M, Kitano H, Matsuoka M (2005) The rice brassinosteroid-deficient dwarf2 mutant defective in the rice homolog of Arabidopsis DIMINUTO/DWARF1 is rescued by the endogenously accumulated alternative bioactive brassinosteroid, dolichosterone. Plant Cell 17:2243–2254
Jager CE, Symons GM, Nomura T, Yamada Y, Smith JJ, Yamaguchi S, Kamiya Y, Weller JL, Yokota T, Reid JB (2007) Characterization of two brassinosteroid C-6 oxidase genes in pea. Plant Physiol 143:1894–1904
Kim TW, Hwang JY, Kim YS, Joo SH, Chang SC, Lee JS, Takatsuto S, Kim SK (2005) Arabidopsis CYP85A2, a cytochrome P450, mediates the Baeyer-Villiger oxidation of castasterone to brassinolide in brassinosteroid biosynthesis. Plant Cell 17:2397–2412
Kim HB, Kwon M, Ryu H, Fujioka S, Takatsuto S, Yoshida S, An CS, Lee I, Hwang I, Choe S (2006) The regulation of DWARF4 expression is likely a critical mechanism in maintaining the homeostasis of bioactive brassinosteroids in Arabidopsis. Plant Physiol 140:548–557
Kim BK, Fujioka S, Takatsuto S, Tsujimoto S, Choe S (2008) Castasterone is a likely end product of brassinosteroid biosynthetic pathway in rice. Biochem Biophys Res Commun 374:614–619
Kwon M, Fujioka S, Jeon JH, Kim HB, Takatsuto S, Oshida S, An CS, Choe S (2005) A double mutant for the CYP85A1 and CYP85A2 genes of Arabidopsis exhibits a brassinosteroid dwarf phenotype. J Plant Biol 48:237–244
Li L, Xu J, Xu ZH, Xue HW (2005) Brassinosteroids stimulate plant tropisms through modulation of polar auxin transport in Brassica and Arabidopsis. Plant Cell 17:2738–2753
Lindsey K, Pullen ML, Topping JF (2003) Importance of plant sterols in pattern formation and hormone signaling. Trends Plant Sci 8:521–525
Maluszynska J, Maluszynski M (1983) MNUA and MH mutagenic effect after double treatment of barley seeds in different germination periods. Acta Biol 11:238–248
Marchler-Bauer A, Anderson JB, Derbyshire MK, DeWeese-Scott C, Gonzales NR, Gwadz M, Hao L, He S, Hurwitz DI, Jackson JD, Ke Z, Krylov D, Lanczycki CJ, Liebert CA, Liu C, Lu F, Lu S, Marchler GH, Mullokandov M, Song JS, Thanki N, Yamashita RA, Yin JJ, Zhang D, Bryant SH (2007) CDD: a conserved domain database for interactive domain family analysis. Nucleic Acids Res 35:237–240
Mathur J, Molnar G, Fujioka S, Takatsuto S, Sakurai A, Yokota T, Adam G, Voigt B, Nagy F, Maas C, Schell J, Koncz C, Szekeres M (1998) Transcription of the Arabidopsis CPD gene, encoding steroidogenic cytochrome P450, is negatively controlled by brassinosteroids. Plant J 14:593–602
Mori M, Nomura T, Ooka H, Ishizaka M, Yokota T, Sugimoto K, Okabe K, Kajiwara H, Satoh K, Yamamoto K, Hirochika H, Kikuchi S (2002) Isolation and characterization of a rice dwarf mutant with a defect in brassinosteroid biosynthesis. Plant Physiol 130:1152–1161
Müssig C, Shin GH, Altmann T (2003) Brassinosteroids promote root growth in Arabidopsis. Plant Physiol 133:1261–1271
Nakamura M, Satoh T, Tanaka SI, Mochizuki N, Yokota T, Nagatani A (2005) Activation of the cytochrome P450 gene, CYP72C1, reduces the levels of active brassinosteroids in vivo. J Exp Bot 56:833–840
Nakamura A, Fujioka S, Sunohara H, Kamiya N, Hong Z, Inukai Y, Miura K, Takatsuto S, Yoshida S, Ueguchi-Tanaka M, Hasegawa Y, Kitano H, Matsuoka M (2006) The role of OsBRI1 and its homologous genes OsBRL1 and OsBRL3 in rice. Plant Physiol 140:580–590
Neff MM, Nguyen SM, Malancharuvil EJ, Fujioka S, Noguchi T, Seto H, Tsubuki M, Honda T, Takatsuto S, Yoshida S, Chory J (1999) BAS1: a gene regulating brassinosteroid levels and light responsiveness in Arabidopsis. Proc Natl Acad Sci USA 96:15316–15323
Noguchi T, Fujioka S, Choe S, Takatsuto S, Tax FE, Yoshida S, Feldmann KA (2000) Biosynthethic pathways of brassinolide in Arabidopsis. Plant Physiol 124:201–209
Nomura T, Sato T, Bishop GJ, Kamiya Y, Takatsuto S, Yokota T (2001) Accumulation of 6-deoxocathasterone and 6-deoxocastasterone in Arabidopsis, pea and tomato is suggestive of common rate-limiting steps in brassinosteroid biosynthesis. Phytochemistry 57:171–178
Nomura T, Bishop GJ, Kaneta T, Reid JB, Chory J, Yokota T (2003) The LKA gene is a BRASSINOSTEROID INSENSITIVE1 homolog of pea. Plant J 36:291–300
Nomura T, Kushiro T, Yokota T, Kamiya Y, Bishop GJ, Yamaguchi S (2005) The last reaction producing brassinolide is catalyzed by cytochrome P450 s, CYP85A3 in tomato and CYP85A2 in Arabidopsis. J Biol Chem 280:17873–17879
Poppenberger B, Fujioka S, Soeno K, George GL, Vaistij FE, Hiranuma S, Seto H, Takatsuto S, Adam G, Yoshida S, Bowles D (2005) The UGT73C5 of Arabidopsis thaliana glucosylates brassinosteroids. Proc Natl Acad Sci USA 102:15253–15258
Russinova E, Borst JW, Kwaaitaal M, Caño-Delgado A, Yin Y, Chory J, de Vries SC (2004) Heterodimerization and endocytosis of Arabidopsis brassinosteroid receptors BRI1 and SERK3 (BAK1). Plant Cell 16:3216–3229
Sakamoto T, Matsuoka M (2006) Characterization of CONSTITUTIVE PHOTOMORPHOGENESIS AND DWARFISM homologs in rice (Oryza sativa L.). J Plant Growth Regul 25:245–251
Schaller H (2003) The role of sterols in plant growth and development. Prog Lipid Res 42:163–175
Shimada Y, Fujioka S, Miyauchi N, Kushiro M, Takatsuto S, Nomura T, Yokota T, Kamiya Y, Bishop GJ, Yoshida S (2001) Brassinosteroid-6-oxidases from Arabidopsis and tomato catalyze multiple C-6 oxidation in brassinosteroid biosynthesis. Plant Physiol 126:770–779
Shimada Y, Goda H, Nakamura A, Takatsuto S, Fujioka S, Yoshida S (2003) Organ-specific expression of brassinosteroid-biosynthethic genes and distribution of endogenous brassinosteroids in Arabidopsis. Plant Physiol 131:287–297
Symons GM, Reid JB (2004) Brassinosteroids do not undergo long-distance transport in pea. Implications for the regulation of endogenous brassinosteroid levels. Plant Physiol 135:2196–2206
Szekeres M, Nemeth K, Koncz-Kalman Z, Mathur J, Kauschmann A, Altmann T, Redei GP, Nagy F, Schell J, Koncz C (1996) Brassinosteroids rescue the deficiency of CYP90, a cytochrome P450, controlling cell elongation and de-etiolation in Arabidopsis. Cell 85:171–182
Tanabe S, Ashikari M, Fujioka S, Takatsuto S, Yoshida S, Yano M, Yoshimura A, Kitano H, Matsuoka M, Fujisawa Y, Kato H, Iwasaki Y (2005) A novel cytochrome P450 is implicated in brassinosteroid biosynthesis via the characterization of a rice dwarf mutant, dwarf11, with reduced seed length. Plant Cell 17:776–790
Tanaka K, Asami T, Yoshida S, Nakamura Y, Matsuo T, Okamoto S (2005) Brassinosteroid homeostasis in Arabidopsis is ensured by feedback expressions of multiple genes involved in its metabolism. Plant Physiol 138:1117–1125
Turk EM, Fujioka S, Seto H, Shimada Y, Takatsuto S, Yoshida S, Denzel MA, Torres QI, Neff MM (2003) CYP72B1 inactivates brassinosteroid hormones: and intersection between photomorphogenesis and plant steroid signal transduction. Plant Physiol 133:1643–1653
Werck-Reichhart D, Feyereisen R (2000) Cytochrome P450: a success story. Genome Biol 1:3000.1–3000.9
Williams PA, Cosme J, Vinkovic DM, Ward A, Angove HC, Day PJ, Vonrhein C, Tickle IJ, Jhoti H (2004) Crystalstructures of human cytochrome P450 3A4 bound to metyrapone and progesterone. Science 305:683–686
Yamamuro C, Ihara Y, Wu X, Noguchi T, Fujioka S, Takatsuto S, Ashikari M, Kitano H, Matsuoka M (2000) Loss of function of a rice brassinosteroid insensitive1 homolog prevents internode elongation and bending of the lamina joint. Plant Cell 12:1591–1605
Yokota T (1997) The structure, biosynthesis and function of brassinosteroids. Trends Plant Sci 2:137–143
Open Access
This article is distributed under the terms of the Creative Commons Attribution Noncommercial License which permits any noncommercial use, distribution, and reproduction in any medium, provided the original author(s) and source are credited.
Author information
Authors and Affiliations
Corresponding author
Electronic supplementary material
Below is the link to the electronic supplementary material.
10725_2011_9607_MOESM1_ESM.tif
Online resource 1 Height of plants of all analyzed genotypes at maturity. Mean values are presented along with SD (TIFF 557 kb)
10725_2011_9607_MOESM2_ESM.tif
Online resource 2 Positive (a) and negative (b) reaction in leaf segment unrolling test. White bars represent width of 2 mm (TIFF 1782 kb)
10725_2011_9607_MOESM4_ESM.tif
Online resource 4 Influence of various concentrations of gibberellic acid on growth rate of seedlings of all analyzed genotypes (TIFF 2174 kb)
10725_2011_9607_MOESM5_ESM.tif
Online resource 5 Sequence alignment of homologous DWARF polypeptides from Oryza sativa, Hordeum vulgare, Arabidopsis thaliana and Lycopersicon esculentum performed with the use of BoxShade v.3.2 tool (TIFF 2715 kb)
10725_2011_9607_MOESM6_ESM.tif
Online resource 6 Location of secondary spatial domains within HvDWARF polypeptide in parental variety ‘Delisa’ (a), mutant 522DK (b) and mutant 527DK (c) (TIFF 2199 kb)
Rights and permissions
Open Access This is an open access article distributed under the terms of the Creative Commons Attribution Noncommercial License (https://creativecommons.org/licenses/by-nc/2.0), which permits any noncommercial use, distribution, and reproduction in any medium, provided the original author(s) and source are credited.
About this article
Cite this article
Gruszka, D., Szarejko, I. & Maluszynski, M. Identification of barley DWARF gene involved in brassinosteroid synthesis. Plant Growth Regul 65, 343–358 (2011). https://doi.org/10.1007/s10725-011-9607-9
Received:
Accepted:
Published:
Issue Date:
DOI: https://doi.org/10.1007/s10725-011-9607-9