Abstract
Introduction
Malignant peripheral nerve sheath tumors (MPNSTs) are malignancies that demonstrate nerve sheath differentiation in the peripheral nervous system. They can occur sporadically or be associated with neurofibromatosis type 1 (NF1), an autosomal dominant neurocutaneous disorder, with up to 13% of patients developing MPNSTs in their lifetimes. Previous studies have suggested conflicting findings regarding the prognosis of NF1 for patients with MPNSTs. The elucidation of NF1 as an independent prognostic factor on mortality has implications for clinical management. We aim to investigate the role of NF1 status as an independent prognostic factor of overall survival (OS) and disease-specific survival (DSS) in MPNSTs.
Methods
An electronic literature search of PubMed and MEDLINE was performed on studies reporting OS or DSS outcomes of MPNSTs with and without NF1. A grey literature search by reviewing bibliographies of included studies and review articles was performed to find pertinent studies. Data was extracted and assessed in accordance with the PRISMA guidelines. A meta-analysis was performed to calculate hazard ratios (HRs) using a random-effects model. The primary and secondary outcomes were all-cause and disease-specific mortality, respectively, with NF1 as an independent prognostic factor of interest.
Results
A total of 59 retrospective studies involving 3602 patients fulfilled the inclusion criteria for OS analysis, and 23 studies involving 704 MPNST patients were included to evaluate DSS outcomes. There was a significant increase in the hazard of all-cause mortality (HR 1.63, 95% CI 1.45 to 1.84) and disease-specific mortality (HR 1.52, 95% CI 1.24 to 1.88) among NF1 as compared to sporadic cases. Subgroup analyses and meta-regression showed that this result was consistent regardless of the quality of the study and year of publication.
Conclusion
NF1 is associated with a substantially higher risk of all-cause and disease-specific mortality. This finding suggests that closer surveillance is required for NF1 patients at risk of developing MPNSTs.
Similar content being viewed by others
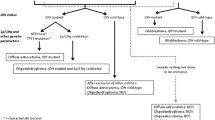
Background
Malignant peripheral nerve sheath tumors (MPNSTs) are malignancies that show nerve sheath differentiation in the peripheral nervous system and have been previously described as neurogenic sarcoma, neurofibrosarcoma, malignant schwannoma, and malignant neurilemmoma [1]. They are rare with an incidence of 1.46 per million person-years in the general population [2]. In neurofibromatosis type 1 (NF1), an autosomal dominant neurocutaneous disorder, with an estimated birth incidence of 1 in 2500, up to 13% of patients develop MPNSTs in their lifetimes [3, 4]. These tumors tend to arise from pre-existing plexiform neurofibromas, which are uncommon in non-NF1 patients [1, 5].
It is unknown if sporadic MPNST (sMPNST) or NF1-related MPNST (nfMPNST) exhibit variable behavior, and there have been conflicting reports on factors predicting survival for patients with sMPNST and nfMPNST. The prognosis for MPNST is poor and has remained abysmal in the last few decades with 5-year survival rates ranging between 16 and 62% [6, 7]. Some studies have reported poorer survival rates in the NF1 group [4, 8,9,10,11], while others have suggested that there is little difference [12, 13]. Biologically, somatic changes in NF1, CDKN2A/B, and polycomb repressor complex 2 (PRC2) are identified in most MPNSTs regardless of NF1 status, and the set of genetic events leading to the development of NF1 associated and sporadic MPNSTs follow a similar pathogenic process [14, 15]. Therefore, the basis of NF1 as an independent (poor) prognostic factor in MPNST has been a constant source of contention. The clarification of NF1’s role in the prognosis of MPNST would enable clinicians to stratify the risk of the disease more effectively. This would have significant implications for staging, follow-up, and subsequent management. It is imperative, as MPNSTs are largely resistant to chemotherapy and radiotherapy, and a timely wide excision with clear margins remains the only possible curative option [16, 17]. In this meta-analysis, our aim is to investigate the impact of NF1 on the mortality of sMPNSTs compared to nfMPNSTs.
Methods
Literature search strategy
This meta-analysis was done in accordance with the Preferred Reporting Items for Systematic Reviews and Meta-Analyses (PRISMA) guidelines [18]. The review protocol was registered in the PROSPERO International Prospective Register of Systematic Reviews, registration number CRD42021275352, https://www.crd.york.ac.uk/prospero/display_record.php?ID=CRD42021275352. A search was conducted on PubMed and MEDLINE (via Ovid) up until September 30, 2021, with no language limitations. The search term employed was “peripheral nervous system neoplasms” [MeSH Terms] OR “nerve sheath neoplasms” [MeSH Terms] AND “malignant” AND “human” AND (prognosis OR mortality OR survival OR clinicopathologic). In addition to PubMed search results, we searched the grey literature by reviewing the bibliographies of the included studies as well as review articles. The reference lists of selected publications were reviewed to find pertinent studies, ensuring that the literature review was systematic and rigorous. The study protocol underwent revision due to the diverse formats of survival data presented in the studies. This required conducting different statistical analyses to extract as much survival data as possible from the existing literature. Figure 1 shows the flowchart of the literature review and study selection for this meta-analysis.
Study selection
Studies reporting overall survival (OS) or disease-specific survival (DSS) outcomes of malignant peripheral nerve sheath tumors with and without NF1 in humans were included. The inclusion criteria were (i) clear diagnosis of NF1 status, (ii) two-arm study involving sMPNSTs and nfMPNSTs, (iii) more than ten patients in each study, (iv) sufficient statistical information to allow quantification of effect based on hazard ratio (HR), and its 95% confidence interval (CI). We set a minimum of ten patients per study as a cutoff to balance the prospect of achieving a reliable survival estimate for hazard ratio analyses and reduce the risk of excluding too many studies given that most study samples are small. Review articles, case reports, animal studies, cell line studies, molecular/genetic/cellular markers studies, and duplicated studies were excluded. As in the previous literature [19], six articles [14, 20,21,22,23,24] were combined according to the same first author to form three distinct studies in our meta-analysis, after verifying that there was no duplication. For three further studies, [9, 25, 26] only the latest published result was included since the prior two studies were incorporated in the last publication.
Data extraction
Data extracted included the name of the first author, publication year, country, study size per arm and overall, age, gender, anatomical location, and median duration of follow-up. For papers that did not report the mean age and its variance, the mean and SD were calculated using the methods described by Hozo et al. [27]. The assessment of the quality of studies was evaluated using the Newcastle–Ottawa Scale (NOS) by two different reviewers (ZL, MB), and disagreements were resolved by consensus or appeal to a third author (MP) [28]. The total quality score ranged from 0 to 9, contributed by three components including selection of the exposed and unexposed cohort, comparability of the groups, and outcome assessment. Studies that scored 7–9, 4–6, and 3 or fewer points were classified as having a low, moderate, or high risk of bias, respectively.
Statistical analysis
Publication bias was evaluated by visual examination of funnel plot of the standard error of log(HR) against log(HR), Egger’s asymmetry test, and the trim-and-fill method [29].
For studies which provided individual patient data (IPD), the HR and SE were estimated via the Cox proportional hazard model. For studies in which the number of events, log-rank test p-value, randomized number in each group, and confidence interval of HR were provided, the HR and SD were calculated according to the methodology described by Parmar et al., using the formula \({\text{exp}}[\left({{\text{O}}}_{{\text{ri}}}-{E}_{ri}\right)/{V}_{ri}]\) [30]. For studies which reported the 3-year or 5-year survival rate digitally or graphically, the pooled HR was estimated using the formulae of Tierney et al., assuming constant censoring within each interval [31]. If the median survival time for each arm was available only, the HR was estimated based on the ratio of median survival time [32]. For studies which provided the Kaplan–Meier (KM) curves only, the individual patient data were reconstructed from the KM curves using the methodology described by Guyot et al., and the HR was estimated via the Cox proportional hazard model [33]. When zero event was recorded in either arm, a continuity correction factor of 0.5 was applied to the number of events and non-events in each arm [34].
The DerSimonian-Laird random-effects model was implemented to derive the pooled estimate of HR and its corresponding 95% confidence interval [35]. This model assumed that the individual studies were drawn at random from a larger population, with each having its own underlying effect size. The interstudy heterogeneity is represented by I [2, 36]. We have further performed a sensitivity analysis excluding studies with confidence intervals (CI) larger than 100 to avoid overestimating the pooled estimate.
Further, meta-regression analyses were conducted based on publication year, NOS scores, and prespecified anatomical location (extremity versus non-extremity) to fully utilize the information by considering the entire range of the data, rather than dichotomizing them as binary variables based on pre-specified cutoffs. Besides, subgroup analysis was performed based on pre-specified anatomical location. As many studies have provided information for both extremity and non-extremity locations, the robust variance estimate was utilized to account for the non-independent effect sizes in the meta-regression based on anatomical location.
All statistical analyses were conducted based on a two-sided test assuming a 5% significance level, using STATA version 17.
Results
Characteristics of studies
These 59 studies selected were all retrospective studies published from 1963 to 2020. More than half were published after 2000 (61%), while 21 (36%) were published after 2010 (Table 1). Most studies (75%) were conducted in the USA or Europe, while studies from Asia were published mostly after 2010. The size of the study varied from 11 to 294 with 16 studies (27%) of size less than 20. The median follow-up duration ranged from 5 to 180 months. The mean age of patients in these studies ranged from 9.5 to 59 years. All studies reviewed were in the English language.
Study quality
The median NOS score was 7 (range 4 to 8), with the majority of the studies having a score of at least 7 (72%) suggesting a low risk of bias. For 16 studies with moderate risk of bias, they were largely due to ambiguity in follow-up duration or not accounting for participants who were lost to follow-up.
Assessment of publication bias
The funnel plot (Additional file 1: Fig. S1) tended to be symmetrical about the pooled log(HR) of 0.48. In addition, Egger’s test revealed no evidence of small-study effects (p = 0.799). However, there was some evidence of heterogeneity between studies \({(I}^{2}=29\%;p=0.02).\) The funnel plot after applying the trim-and-fill method (Additional file 2: Fig. S2) is consistent with the previous observation, and it tended to be symmetrical about the log(HR). The mean effect size based on 59 studies is 0.49 (95% CI 0.37 to 0.61), and the updated estimate based on 62 studies is 0.48 (95% CI 0.36 to 0.60).
Meta-analysis of 59 articles (OS) and 23 articles (DSS)
The 59 studies included in the meta-analysis for OS involved a total of 3602 patients and 2141 events [4, 8,9,10,11,12,13,14, 20, 21, 23, 24, 37,38,39,40,41,42,43,44,45,46,47,48,49,50,51,52,53,54,55,56,57,58,59,60,61,62,63,64,65,66,67,68,69,70,71,72,73,74,75,76,77,78,79,80,81,82,83,84,85]. There were 1361 patients in the nfMPNST arm and 2241 patients in the sMPNST arm. The HR of individual studies ranged from 0.18 to 17.04, and the pooled random effect estimate was 1.63 (95% CI 1.45 to 1.84) (Fig. 2). The exclusion of 2 studies with CI > 100, (Arponchayon et al. 1984 and An et al. 2017) did not affect the effect estimate, HR = 1.62 (92% CI 1.44–1.82).
The 23 studies included in the meta-analysis for DSS involved a total of 704 patients and 393 events [12, 20, 37, 40, 43, 45, 47, 51, 55, 59, 60, 62, 64, 66, 68, 69, 71,72,73,74, 76, 79, 82]. There were 330 patients in the nfMPNST arm and 374 patients in the sMPNST arm. The HR of individual studies ranged from 0.20 to 3.96, and the pooled random effect estimate was 1.52 (95% CI 1.24 to 1.88) (Fig. 3). The exclusion of a study with CI > 100 (An et al. 2017) did not affect the effect estimate, HR = 1.52 (95% CI 1.23– 1.88).
Meta-regression by publication year
Twenty-three (39%) studies involving a total of 974 patients were published before 2000, with sample sizes ranging from 11 [60] to 165 [75]. Thirty-six studies including a total of 2628 patients were published post-2000, with study size ranging from 11 [55] to 294 [77]. The meta-regression suggested that the effect of NF1 on OS did not change according to the year of publication (β = − 0.001, 95% CI − 0.010 to 0.008, p = 0.881) (Additional file 3: Fig. S3). Similarly, the meta-regression suggested that the effect of NF1 on DSS did not change according to the year of publication (β = 0.001, 95% CI − 0.017 to 0.020, p = 0.895) (Additional file 4: Fig. S4).
Meta-regression by NOS score
There were 43 studies with scores ≥ 7 involving 2823 patients and 16 studies involving 779 patients with scores less than 7. The regression coefficient for the NOS score was 0.02 (95% CI − 0.09 to 0.13, p = 0.705), suggesting that the effect of NF1 on OS was relatively unaffected by the NOS score (Additional file 5: Fig. S5). The regression coefficient for the NOS score on DSS was − 0.09 (95% CI − 0.29 to 0.11, p = 0.386), suggesting that the effect of NF1 on DSS was also relatively unaffected by the NOS score (Additional file 6: Fig. S6).
Subgroup analysis and meta-regression by anatomical location
A total of 24 studies provided information for subgroup analysis based on anatomical location. Of these, 11 studies included both extremity and non-extremity locations, 5 studies had information for only extremity location, and 8 studies provided information for only non-extremity locations. A total of 388 patients with extremity and 400 patients with non-extremity location were thus included. The HR for the analysis of OS ranged from 0.36 to 31.11 for the 16 studies that reported extremity location and 0.18 to 8.49 for the 19 studies that reported non-extremity locations. There was no difference in HR of all-cause mortality between studies that reported extremity (HR = 1.44, 95% CI 0.95 to 2.17) and those that reported non-extremity (HR = 1.36, 95% CI 0.90 to 2.05) (p = 0.847) (Fig. 4). The effect estimate of location based on meta-regression was 1.04 (95% CI 0.53 to 2.03, p = 0.906). The HR for the analysis of DSS ranged from 0.63 to 31.11 for the 10 studies that reported extremity location and 0.20 to 8.49 for the 13 studies that reported non-extremity locations. There was also no difference in HR of disease-specific mortality between studies that reported extremity (HR = 1.16, 95% CI 0.70 to 1.91) and those that reported non-extremity (HR = 1.47, 95% CI 0.89 to 2.42) (p = 0.51) (Fig. 5). The effect estimate of location based on meta-regression was 0.80 (95% CI 0.36 to 1.82, p = 0.568).
Discussion
MPNSTs are associated with the highest risk of mortality among soft tissue sarcomas [86]. Due to its rarity, there are few large studies on factors predicting survival. Among these factors, there is a lack of consensus on whether NF1 is an independent indicator of poor prognosis. In this present meta-analysis of 3602 individuals with MPNSTs, we found that NF1 was associated with a 63% increase in the hazard of all-cause mortality and a 52% increase in the hazard of disease-specific mortality compared to sporadic cases. This is similar to the findings by other authors which have reported poorer prognoses for NF1 patients [9, 87], with some demonstrating worse survival outcomes in the NF1 group despite comparable histological grades and tumor sizes [8, 16, 75].
However, there had been previous studies that reported no difference in survival outcomes of MPNST between NF1 and non-NF1 patients [12, 13, 88]. In a large MPNST meta-analysis, Kolberg et al. proposed the notion that the survival difference between NF1 and sporadic MPNSTs was diminishing with a trend towards improved survival in NF1 patients with additional data from more recent publications [19]. To better evaluate the association of survival data with the period of publication, we conducted a meta-regression by year of publication. Our results did not support this notion as we did not detect any difference in the effect of NF1 on all-cause mortality across the years of publication. This can be explained by the lack of novel therapeutic developments for MPNSTs in the past several decades as they remain largely resistant to chemotherapy and radiotherapy [86]. To date, no molecular targeted therapy has demonstrated efficacy in the reduction of tumor size or improvement in survival outcomes [89]. Complete surgical extirpation with clear margins remains the only potential curative therapy for MPNST [17]. The quantification of effect estimates of our study based on HR compared to odds ratio (OR) in Kolberg’s study could also contribute to our varied conclusions. We opted to use HR analysis as this fully utilizes the available information on when a MPNST mortality occurs, instead of simply considering whether an event occurs in an OR analysis.
Given that NF1 has been established as a significant predictor of mortality, we propose considering NF1 status when staging MPNSTs and advocate closer monitoring of these patients. It is imperative that health systems take extra measures to ensure that NF1 patients have prompt and convenient access to specialized care, particularly if they have suspicions of malignancies. Furthermore, we recommend that clinicians lower their threshold in obtaining advanced imaging to facilitate early detection of tumors in this (NF1) population.
While we conclusively show that NF1 is a significant poor prognostic factor in MPNST, the reason for this remains unanswered. From a biological standpoint, suggestions that NF1 tumors are inherently more aggressive have been disputed as the genomic aberrations in NF1, CDKN2A, Polycomb repressive complex 2 (PRC2), PDGFRA, EGFR, MET, and TP53 found in both nfMPNST and sMPNST are largely similar [90] (p2), [91]. On the other hand, there is data to suggest that tumorigenesis begins earlier for NF1 patients, and these tumors are more resistant to treatment, leading to poorer outcomes [42]. We postulate that while the genomic aberrations are similar even for MPNSTs of divergent differentiation, the precise sequence of pathogenic events may be crucial in our understanding of the disease [91, 92]. The findings of our study suggest that NF1 patients require closer clinical follow-up to diagnose and treat these aggressive tumors early.
In terms of anatomical sites of presentation, they are largely similar for both sporadic and NF1 patients [9]. Some studies have reported better survival outcomes for MPNSTs in distal extremities [75, 88], as well as lower rates of recurrence [88]. As part of our study, we did a subgroup analysis to distill the effect of anatomical location on clinical outcomes. We found no significant difference in OS and DSS for extremity and non-extremity MPNSTs. We postulate that the prognostic value of the anatomical site of the disease may not be as important as attaining a negative margin, which has been proven to be an important factor in predicting local recurrence and survival among patients with soft tissue sarcomas [93,94,95,96,97]. Another plausible explanation is the relative ease of achieving a negative resection margin in the extremity and the additional benefit of clinical surveillance for tumors of the extremities, where local recurrences may be more apparent without the need for image-guided surveillance.
Our study has several limitations and caveats. First, it is important to recognize limitations with MPNST being a rare condition, and there is a lack of standardization in terms of follow-up and data collection for the studies. The rarity of MPNSTs explains the heterogeneity seen in our forest plots, like in previous analyses. Second, we acknowledged that NF1 patients are vulnerable to other causes of mortality apart from MPNSTs and hence included DSS analysis to reduce potential biases. A previous large meta-analysis of > 1800 patients has found no discernible difference between overall survival and disease-specific survival when comparing NF1 and sporadic cases of MPNST [19]. This is in contrast to our findings where NF1 was associated with an increased hazard of both all-cause and disease-specific mortality. Third, it is crucial to recognize that the treatment regime for each study was different with some offering adjuvant chemoradiotherapy as an option. However, this information was often not provided in these studies to allow for adjustment. Fourth, all our studies are retrospective and observational in nature. The intrinsic biases of observational studies such as loss to follow-up and confounders hence cannot be eliminated. Subgroup analyses that we have performed may also have limited power to detect the differences due to small numbers. However, it is unlikely that a randomized controlled trial with sufficient power can be established given the low incidence of MPNSTs.
Conclusion
The results of this study show that NF1 is associated with a substantially higher risk of all-cause and disease-specific mortality for MPNSTs. We found no significant change in the association of NF1 as a poor prognostic indicator of mortality with time of publication or with the anatomical location of the tumor. As such, clinicians should consider NF1 status in staging the disease and closer monitoring of NF1 patients at risk of developing MPNSTs to enhance their survival rates through timely intervention.
Availability of data and materials
The datasets used and/or analyzed during the current study are available from the corresponding author upon reasonable request.
Abbreviations
- MPNST:
-
Malignant peripheral nerve sheath tumors
- NF1:
-
Neurofibromatosis type I
- sMPNST:
-
Sporadic MPNST
- nfMPNST:
-
NF1-related MPNST
- KM:
-
Kaplan-Meier
- OS:
-
Overall survival
- DSS:
-
Disease-specific survival
- NOS:
-
Newcastle-Ottawa Score
- HR:
-
Hazard ratio
- OR:
-
Odds ratio
- PRISMA:
-
Preferred Reporting Items for Systematic Reviews and Meta-Analyses
- IPD:
-
Individual patient data
References
Ferner RE, Gutmann DH. International consensus statement on malignant peripheral nerve sheath tumors in neurofibromatosis. Cancer Res. 2002;62(5):1573–7.
Bates JE, Peterson CR, Dhakal S, Giampoli EJ, Constine LS. Malignant peripheral nerve sheath tumors (MPNST): a SEER analysis of incidence across the age spectrum and therapeutic interventions in the pediatric population. Pediatr Blood Cancer. 2014;61(11):1955–60. https://doi.org/10.1002/pbc.25149.
Huson SM, Compston DA, Clark P, Harper PS. A genetic study of von Recklinghausen neurofibromatosis in south east Wales. I. Prevalence, fitness, mutation rate, and effect of parental transmission on severity. J Med Genet. 1989;26(11):704–11. https://doi.org/10.1136/jmg.26.11.704.
Evans DGR, Baser ME, McGaughran J, Sharif S, Howard E, Moran A. Malignant peripheral nerve sheath tumours in neurofibromatosis 1. J Med Genet. 2002;39(5):311–4. https://doi.org/10.1136/jmg.39.5.311.
Friedman JM, Birch PH. Type 1 neurofibromatosis: a descriptive analysis of the disorder in 1,728 patients. Am J Med Genet. 1997;70(2):138–43. https://doi.org/10.1002/(sici)1096-8628(19970516)70:2%3c138::aid-ajmg7%3e3.0.co;2-u.
Cai Z, Tang X, Liang H, Yang R, Yan T, Guo W. Prognosis and risk factors for malignant peripheral nerve sheath tumor: a systematic review and meta-analysis. World J Surg Oncol. 2020;18(1):257. https://doi.org/10.1186/s12957-020-02036-x.
Sobczuk P, Teterycz P, Czarnecka AM, et al. Malignant peripheral nerve sheath tumors – outcomes and prognostic factors based on the reference center experience. Surg Oncol. 2020;35:276–84. https://doi.org/10.1016/j.suronc.2020.09.011.
Porter DE, Prasad V, Foster L, Dall GF, Birch R, Grimer RJ. Survival in malignant peripheral nerve sheath tumours: a comparison between sporadic and neurofibromatosis type 1-associated tumours. Sarcoma. 2009;2009:1–5. https://doi.org/10.1155/2009/756395.
Ducatman BS, Scheithauer BW, Piepgras DG, Reiman HM, Ilstrup DM. Malignant peripheral nerve sheath tumors. A clinicopathologic study of 120 cases. Cancer. 1986;57(10):2006–21. https://doi.org/10.1002/1097-0142(19860515)57:10%3c2006::aid-cncr2820571022%3e3.0.co;2-6.
Wong WW, Hirose T, Scheithauer BW, Schild SE, Gunderson LL. Malignant peripheral nerve sheath tumor: analysis of treatment outcome. Int J Radiat Oncol Biol Phys. 1998;42(2):351–60. https://doi.org/10.1016/s0360-3016(98)00223-5.
Tabone-Eglinger S, Bahleda R, Côté JF, et al. Frequent EGFR positivity and overexpression in high-grade areas of human MPNSTs. Sarcoma. 2008;2008:849156. https://doi.org/10.1155/2008/849156.
Hruban RH, Shiu MH, Senie RT, Woodruff JM. Malignant peripheral nerve sheath tumors of the buttock and lower extremity. A study of 43 cases. Cancer. 1990;66(6):1253–65. https://doi.org/10.1002/1097-0142(19900915)66:6%3c1253::aid-cncr2820660627%3e3.0.co;2-r.
Cashen DV, Parisien RC, Raskin K, Hornicek FJ, Gebhardt MC, Mankin HJ. Survival data for patients with malignant schwannoma. Clin Orthop. 2004;426:69–73. https://doi.org/10.1097/01.blo.0000131256.82455.c5.
Brekke HR, Ribeiro FR, Kolberg M, et al. Genomic changes in chromosomes 10, 16, and X in malignant peripheral nerve sheath tumors identify a high-risk patient group. J Clin Oncol Off J Am Soc Clin Oncol. 2010;28(9):1573–82. https://doi.org/10.1200/JCO.2009.24.8989.
Pemov A, Li H, Presley W, Wallace MR, Miller DT. Genetics of human malignant peripheral nerve sheath tumors. Neuro-Oncol Adv. 2020;2(Supplement_1):i50–61. https://doi.org/10.1093/noajnl/vdz049.
Nambisan RN, Rao U, Moore R, Karakousis CP. Malignant soft tissue tumors of nerve sheath origin. J Surg Oncol. 1984;25(4):268–72. https://doi.org/10.1002/jso.2930250410.
Farid M, Demicco EG, Garcia R, et al. Malignant peripheral nerve sheath tumors. Oncologist. 2014;19(2):193–201. https://doi.org/10.1634/theoncologist.2013-0328.
Page MJ, McKenzie JE, Bossuyt PM, et al. The PRISMA 2020 statement: an updated guideline for reporting systematic reviews. BMJ. 2021:71. https://doi.org/10.1136/bmj.n71. Published online March 29.
Kolberg M, Høland M, Agesen TH, et al. Survival meta-analyses for >1800 malignant peripheral nerve sheath tumor patients with and without neurofibromatosis type 1. Neuro-Oncol. 2013;15(2):135–47. https://doi.org/10.1093/neuonc/nos287.
Brekke HR, Kolberg M, Skotheim RI, et al. Identification of p53 as a strong predictor of survival for patients with malignant peripheral nerve sheath tumors. Neuro-Oncol. 2009;11(5):514–28. https://doi.org/10.1215/15228517-2008-127.
D’agostino AN, Soule EH, Miller RH. Sarcomas of the peripheral nerves and somatic soft tissues associated with multiple neurofibromatosis (von Recklinghausen’s disease). Cancer. 1963;16:1015–27. https://doi.org/10.1002/1097-0142(196308)16:8%3c1015::aid-cncr2820160808%3e3.0.co;2-i.
D’agostino AN, Soule EH, Miller RH. Primary malignant neoplasms of nerves (malignant neurilemomas) in patients without manifestations of multiple neurofibromatosis (von Recklinghausen’s disease). Cancer. 1963;16:1003–14. https://doi.org/10.1002/1097-0142(196308)16:8%3c1003::aid-cncr2820160807%3e3.0.co;2-s.
Schmidt H, Würl P, Taubert H, et al. Genomic imbalances of 7p and 17q in malignant peripheral nerve sheath tumors are clinically relevant. Genes Chromosomes Cancer. 1999;25(3):205–11.
Schmidt H, Taubert H, Meye A, et al. Gains in chromosomes 7, 8q, 15q and 17q are characteristic changes in malignant but not in benign peripheral nerve sheath tumors from patients with Recklinghausen’s disease. Cancer Lett. 2000;155(2):181–90. https://doi.org/10.1016/S0304-3835(00)00426-2.
Ducatman BS, Scheithauer BW. Postirradiation neurofibrosarcoma. Cancer. 1983;51(6):1028–33. https://doi.org/10.1002/1097-0142(19830315)51:6%3c1028::aid-cncr2820510610%3e3.0.co;2-3.
Ducatman BS, Scheithauer BW. Malignant peripheral nerve sheath tumors with divergent differentiation. Cancer. 1984;54(6):1049–57. https://doi.org/10.1002/1097-0142(19840915)54:6%3c1049::aid-cncr2820540620%3e3.0.co;2-1.
Hozo SP, Djulbegovic B, Hozo I. Estimating the mean and variance from the median, range, and the size of a sample. BMC Med Res Methodol. 2005;5(1):13. https://doi.org/10.1186/1471-2288-5-13.
Stang A. Critical evaluation of the Newcastle-Ottawa scale for the assessment of the quality of nonrandomized studies in meta-analyses. Eur J Epidemiol. 2010;25(9):603–5. https://doi.org/10.1007/s10654-010-9491-z.
Harbord RM, Harris RJ, Sterne JA. Updated tests for small–study effects in meta–analyses. Stata J. 2009;9(2):197–210.
Parmar MK, Torri V, Stewart L. Extracting summary statistics to perform meta-analyses of the published literature for survival endpoints. Stat Med. 1998;17(24):2815–34. https://doi.org/10.1002/(sici)1097-0258(19981230)17:24%3c2815::aid-sim110%3e3.0.co;2-8.
Tierney JF, Stewart LA, Ghersi D, Burdett S, Sydes MR. Practical methods for incorporating summary time-to-event data into meta-analysis. Trials. 2007;8:16. https://doi.org/10.1186/1745-6215-8-16.
Cortés J, González JA, Campbell MJ, Cobo E. A hazard ratio was estimated by a ratio of median survival times, but with considerable uncertainty. J Clin Epidemiol. 2014;67(10):1172–7. https://doi.org/10.1016/j.jclinepi.2014.05.017.
Guyot P, Ades AE, Ouwens MJNM, Welton NJ. Enhanced secondary analysis of survival data: reconstructing the data from published Kaplan-Meier survival curves. BMC Med Res Methodol. 2012;12:9. https://doi.org/10.1186/1471-2288-12-9.
Sweeting MJ, Sutton AJ, Lambert PC. What to add to nothing? Use and avoidance of continuity corrections in meta-analysis of sparse data. Stat Med. 2004;23(9):1351–75. https://doi.org/10.1002/sim.1761.
DerSimonian R, Laird N. Meta-analysis in clinical trials. Control Clin Trials. 1986;7(3):177–88. https://doi.org/10.1016/0197-2456(86)90046-2.
Higgins JPT, Thompson SG, Deeks JJ, Altman DG. Measuring inconsistency in meta-analyses. BMJ. 2003;327(7414):557–60. https://doi.org/10.1136/bmj.327.7414.557.
Angelov L, Davis A, O’Sullivan B, Bell R, Guha A. Neurogenic sarcomas: experience at the University of Toronto. Neurosurgery. 1998;43(1):56–64. https://doi.org/10.1097/00006123-199807000-00035.
Ariel IM. Tumors of the peripheral nervous system. CA Cancer J Clin. 1983;33(5):282–99. https://doi.org/10.3322/canjclin.33.5.282.
Arpornchayanon O, Hirota T, Itabashi M, et al. Malignant peripheral nerve tumors: a clinicopathological and electron microscopic study. Jpn J Clin Oncol. 1984;14(1):57–74.
Bergamaschi L, Bisogno G, Manzitti C, et al. Salvage rates and prognostic factors after relapse in children and adolescents with malignant peripheral nerve sheath tumors. Pediatr Blood Cancer. 2018;65(2):e26816. https://doi.org/10.1002/pbc.26816.
Bojsen-Møller M, Myhre-Jensen O. A consecutive series of 30 malignant schwannomas. Survival in relation to clinico-pathological parameters and treatment. Acta Pathol Microbiol Immunol Scand [A]. 1984;92(3):147–55.
Carli M, Ferrari A, Mattke A, et al. Pediatric malignant peripheral nerve sheath tumor: the Italian and German Soft Tissue Sarcoma Cooperative Group. J Clin Oncol. 2005;23(33):8422–30. https://doi.org/10.1200/JCO.2005.01.4886.
Casanova M, Ferrari A, Spreafico F, et al. Malignant peripheral nerve sheath tumors in children: a single-institution twenty-year experience. J Pediatr Hematol Oncol. 1999;21(6):509–13.
Daimaru Y, Hashimoto H, Enjoji M. Malignant peripheral nerve-sheath tumors (malignant schwannomas): an immunohistochemical study of 29 cases. Am J Surg Pathol. 1985;9(6):434–44. https://doi.org/10.1097/00000478-198506000-00005.
deCou JM, Rao BN, Parham DM, et al. Malignant peripheral nerve sheath tumors: the St. Jude Children’s Research Hospital experience. Ann Surg Oncol. 1995;2(6):524–9. https://doi.org/10.1007/BF02307086.
de Vasconcelos RAT, Coscarelli PG, Alvarenga RP, Acioly MA. Malignant peripheral nerve sheath tumor with and without neurofibromatosis type 1. Arq Neuropsiquiatr. 2017;75(6):366–71. https://doi.org/10.1590/0004-282x20170052.
Demir HA, Varan A, Yalçn B, Akyüz C, Kutluk T, Büyükpamukçu M. Malignant peripheral nerve sheath tumors in childhood: 13 cases from a single center. J Pediatr Hematol Oncol. 2012;34(3):204–7. https://doi.org/10.1097/MPH.0b013e31822d4cef.
Doorn PF, Molenaar WM, Buter J, Hoekstra HJ. Malignant peripheral nerve sheath tumors in patients with and without neurofibromatosis. Eur J Surg Oncol EJSO. 1995;21(1):78–82. https://doi.org/10.1016/S0748-7983(05)80073-3.
Fan Q, Yang J, Wang G. Clinical and molecular prognostic predictors of malignant peripheral nerve sheath tumor. Clin Transl Oncol. 2014;16(2):191–9. https://doi.org/10.1007/s12094-013-1061-x.
Ganju A, Roosen N, Kline DG, Tiel RL. Outcomes in a consecutive series of 111 surgically treated plexal tumors: a review of the experience at the Louisiana State University Health Sciences Center. J Neurosurg. 2001;95(1):51–60. https://doi.org/10.3171/jns.2001.95.1.0051.
Le Guellec S, Decouvelaere AV, Filleron T, et al. Malignant peripheral nerve sheath tumor is a challenging diagnosis: a systematic pathology review, immunohistochemistry, and molecular analysis in 160 patients from the French Sarcoma Group Database. Am J Surg Pathol. 2016;40(7):896–908. https://doi.org/10.1097/PAS.0000000000000655.
Hagel C, Zils U, Peiper M, et al. Histopathology and clinical outcome of NF1-associated vs. sporadic malignant peripheral nerve sheath tumors. J Neurooncol. 2007;82(2):187–92. https://doi.org/10.1007/s11060-006-9266-2.
Halling KC, Scheithauer BW, Halling AC, et al. p53 expression in neurofibroma and malignant peripheral nerve sheath tumor: an immunohistochemical study of sporadic and NF1-associated tumors. Am J Clin Pathol. 1996;106(3):282–8. https://doi.org/10.1093/ajcp/106.3.282.
Holtkamp N, Atallah I, Okuducu AF, et al. MMP-13, p53 in the progression of malignant peripheral nerve sheath tumors. Neoplasia. 2007;9(8):671–7. https://doi.org/10.1593/neo.07304.
An HY, Hong KT, Kang HJ, et al. Malignant peripheral nerve sheath tumor in children: a single-institute retrospective analysis. Pediatr Hematol Oncol. 2017;34(8):468–77. https://doi.org/10.1080/08880018.2017.1408730.
Hwang IK, Hahn SM, Kim HS, et al. Outcomes of treatment for malignant peripheral nerve sheath tumors: different clinical features associated with neurofibromatosis type 1. Cancer Res Treat. 2017;49(3):717–26. https://doi.org/10.4143/crt.2016.271.
Kamran SC, Howard SA, Shinagare AB, et al. Malignant peripheral nerve sheath tumors: prognostic impact of rhabdomyoblastic differentiation (malignant triton tumors), neurofibromatosis 1 status and location. Eur J Surg Oncol EJSO. 2013;39(1):46–52. https://doi.org/10.1016/j.ejso.2012.09.001.
Kar M, Deo SVS, Shukla NK, et al. Malignant peripheral nerve sheath tumors (MPNST) – clinicopathological study and treatment outcome of twenty-four cases. World J Surg Oncol. 2006;4(1):55. https://doi.org/10.1186/1477-7819-4-55.
Kourea HP, Bilsky MH, Leung DH, Lewis JJ, Woodruff JM. Subdiaphragmatic and intrathoracic paraspinal malignant peripheral nerve sheath tumors: a clinicopathologic study of 25 patients and 26 tumors. Cancer. 1998;82(11):2191–203. https://doi.org/10.1002/(sici)1097-0142(19980601)82:11%3c2191::aid-cncr14%3e3.0.co;2-p.
Kunisada T, Kawai A, Ozaki T, Sugihara S, Taguchi K, Inoue H. A clinical analysis of malignant schwannoma. https://doi.org/10.18926/AMO/30784. Published online April 1997. Accessed September 6, 2021
Lamm W, Schur S, Köstler WJ, et al. Clinical signs of neurofibromatosis impact on the outcome of malignant peripheral nerve sheath tumors. Oncology. 2014;86(2):122–6. https://doi.org/10.1159/000357137.
Lodding P, Kindblom LG, Angervall L. Epithelioid malignant schwannoma: a study of 14 cases. Virchows Arch A Pathol Anat Histopathol. 1986;409(4):433–51. https://doi.org/10.1007/BF00705415.
Longhi A, Errani C, Magagnoli G, et al. High grade malignant peripheral nerve sheath tumors: outcome of 62 patients with localized disease and review of the literature. J Chemother Florence Italy. 2010;22(6):413–8. https://doi.org/10.1179/joc.2010.22.6.413.
Loree TR, North JH, Werness BA, Nangia R, Mullins AP, Hicks WL. Malignant peripheral nerve sheath tumors of the head and neck: analysis of prognostic factors. Otolaryngol Neck Surg. 2000;122(5):667–72. https://doi.org/10.1067/mhn.2000.106191.
Martin E, Coert JH, Flucke UE, et al. Neurofibromatosis-associated malignant peripheral nerve sheath tumors in children have a worse prognosis: a nationwide cohort study. Pediatr Blood Cancer. 2020;67(4):e28138. https://doi.org/10.1002/pbc.28138.
McCarron KF, Goldblum JR. Plexiform neurofibroma with and without associated malignant peripheral nerve sheath tumor: a clinicopathologic and immunohistochemical analysis of 54 cases. Mod Pathol Off J U S Can Acad Pathol Inc. 1998;11(7):612–7.
McCaughan JA, Holloway SM, Davidson R, Lam WWK. Further evidence of the increased risk for malignant peripheral nerve sheath tumour from a Scottish cohort of patients with neurofibromatosis type 1. J Med Genet. 2007;44(7):463–6. https://doi.org/10.1136/jmg.2006.048140.
Meis JM, Enzinger FM, Martz KL, Neal JA. Malignant peripheral nerve sheath tumors (malignant schwannomas) in children. Am J Surg Pathol. 1992;16(7):694–707. https://doi.org/10.1097/00000478-199207000-00008.
Mertens F, Dal Cin P, De Wever I, et al. Cytogenetic characterization of peripheral nerve sheath tumours: a report of the CHAMP study group. J Pathol. 2000;190(1):31–8. https://doi.org/10.1002/(SICI)1096-9896(200001)190:1%3c31::AID-PATH505%3e3.0.CO;2-#.
Miao R, Wang H, Jacobson A, et al. Radiation-induced and neurofibromatosis-associated malignant peripheral nerve sheath tumors (MPNST) have worse outcomes than sporadic MPNST. Radiother Oncol. 2019;137:61–70. https://doi.org/10.1016/j.radonc.2019.03.015.
Okada K, Hasegawa T, Tajino T, et al. Clinical relevance of pathological grades of malignant peripheral nerve sheath tumor: a multi-institution TMTS study of 56 cases in northern Japan. Ann Surg Oncol. 2007;14(2):597–604. https://doi.org/10.1245/s10434-006-9053-5.
Owosho AA, Estilo CL, Huryn JM, Chi P, Antonescu CR. A clinicopathologic study of head and neck malignant peripheral nerve sheath tumors. Head Neck Pathol. 2018;12(2):151–9. https://doi.org/10.1007/s12105-017-0841-y.
Raney B, Schnaufer L, Ziegler M, Chatten J, Littman P, Jarrett P. Treatment of children with neurogenic sarcoma Experience at the Children’s Hospital of Philadelphia, 1958–1984. Cancer. 1987;59(1):1–5. https://doi.org/10.1002/1097-0142(19870101)59:1%3c1::aid-cncr2820590105%3e3.0.co;2-a.
Scheithauer BW, Erdogan S, Rodriguez FJ, et al. Malignant peripheral nerve sheath tumors of cranial nerves and intracranial contents: a clinicopathologic study of 17 cases. Am J Surg Pathol. 2009;33(3):325–38. https://doi.org/10.1097/PAS.0b013e31818d6470.
Sordillo PP, Helson L, Hajdu SI, et al. Malignant schwannoma–clinical characteristics, survival, and response to therapy. Cancer. 1981;47(10):2503–9. https://doi.org/10.1002/1097-0142(19810515)47:10%3c2503::aid-cncr2820471033%3e3.0.co;2-3.
Trojanowski JQ, Kleinman GM, Proppe KH. Malignant tumors of nerve sheath origin. Cancer. 1980;46(5):1202–12. https://doi.org/10.1002/1097-0142(19800901)46:5%3c1202::aid-cncr2820460521%3e3.0.co;2-5.
Valentin T, Le Cesne A, Ray-Coquard I, et al. Management and prognosis of malignant peripheral nerve sheath tumors: the experience of the French Sarcoma Group (GSF-GETO). Eur J Cancer Oxf Engl. 1990;2016(56):77–84. https://doi.org/10.1016/j.ejca.2015.12.015.
Noesel MM, Orbach D, Brennan B, et al. Outcome and prognostic factors in pediatric malignant peripheral nerve sheath tumors: an analysis of the European Pediatric Soft Tissue Sarcoma Group (EpSSG) NRSTS‐2005 prospective study. Pediatr Blood Cancer. 2019;66(10). doi:https://doi.org/10.1002/pbc.27833
Wang J, Ou S wu, Guo Z ze, Wang Y jie, Xing D guang. Microsurgical management of giant malignant peripheral nerve sheath tumor of the scalp: two case reports and a literature review. World J Surg Oncol. 2013;11(1):269. https://doi.org/10.1186/1477-7819-11-269
Wang T, Yin H, Han S, et al. Malignant peripheral nerve sheath tumor (MPNST) in the spine: a retrospective analysis of clinical and molecular prognostic factors. J Neurooncol. 2015;122(2):349–55. https://doi.org/10.1007/s11060-015-1721-5.
Watson MA, Perry A, Tihan T, et al. Gene expression profiling reveals unique molecular subtypes of neurofibromatosis type i-associated and sporadic malignant peripheral nerve sheath tumors. Brain Pathol. 2006;14(3):297–303. https://doi.org/10.1111/j.1750-3639.2004.tb00067.x.
White HR. Survival in malignant schwannoma. An 18-year study. Cancer. 1971;27(3):720–9. https://doi.org/10.1002/1097-0142(197103)27:3%3c720::aid-cncr2820270331%3e3.0.co;2-d.
Yu J, Deshmukh H, Payton JE, et al. Array-based comparative genomic hybridization identifies CDK4 and FOXM1 alterations as independent predictors of survival in malignant peripheral nerve sheath tumor. Clin Cancer Res. 2011;17(7):1924–34. https://doi.org/10.1158/1078-0432.CCR-10-1551.
Yuan Z, Xu L, Zhao Z, et al. Clinicopathological features and prognosis of malignant peripheral nerve sheath tumor: a retrospective study of 159 cases from 1999 to 2016. Oncotarget. 2017;8(62):104785–95. https://doi.org/10.18632/oncotarget.18975.
Zhou W, Du X, Song F, et al. Prognostic roles for fibroblast growth factor receptor family members in malignant peripheral nerve sheath tumor. Oncotarget. 2016;7(16):22234–44. https://doi.org/10.18632/oncotarget.8067.
Kattan MW, Leung DHY, Brennan MF. Postoperative nomogram for 12-year sarcoma-specific death. J Clin Oncol Off J Am Soc Clin Oncol. 2002;20(3):791–6. https://doi.org/10.1200/JCO.2002.20.3.791.
Ghosh BC, Ghosh L, Huvos AG, Fortner JG. Malignant schwannoma. A clinicopathologic study. Cancer. 1973;31(1):184–90. https://doi.org/10.1002/1097-0142(197301)31:1%3c184::aid-cncr2820310126%3e3.0.co;2-8.
Anghileri M, Miceli R, Fiore M, et al. Malignant peripheral nerve sheath tumors: prognostic factors and survival in a series of patients treated at a single institution. Cancer. 2006;107(5):1065–74. https://doi.org/10.1002/cncr.22098.
Widemann BC, Italiano A. Biology and management of undifferentiated pleomorphic sarcoma, myxofibrosarcoma, and malignant peripheral nerve sheath tumors: state of the art and perspectives. J Clin Oncol. 2018;36(2):160–7. https://doi.org/10.1200/JCO.2017.75.3467.
Lee W, Teckie S, Wiesner T, et al. PRC2 is recurrently inactivated through EED or SUZ12 loss in malignant peripheral nerve sheath tumors. Nat Genet. 2014;46(11):1227–32. https://doi.org/10.1038/ng.3095.
Carroll SL. The challenge of cancer genomics in rare nervous system neoplasms: malignant peripheral nerve sheath tumors as a paradigm for cross-species comparative oncogenomics. Am J Pathol. 2016;186(3):464–77. https://doi.org/10.1016/j.ajpath.2015.10.023.
Wu LMN, Deng Y, Wang J, et al. Programming of Schwann cells by Lats1/2-TAZ/YAP signaling drives malignant peripheral nerve sheath tumorigenesis. Cancer Cell. 2018;33(2):292-308.e7. https://doi.org/10.1016/j.ccell.2018.01.005.
Enneking WF, Spanier SS, Goodman MA. A system for the surgical staging of musculoskeletal sarcoma. Clin Orthop. 1980;153:106–20.
Gronchi A, Casali PG, Mariani L, et al. Status of surgical margins and prognosis in adult soft tissue sarcomas of the extremities: a series of patients treated at a single institution. J Clin Oncol Off J Am Soc Clin Oncol. 2005;23(1):96–104. https://doi.org/10.1200/JCO.2005.04.160.
Stojadinovic A, Leung DHY, Hoos A, Jaques DP, Lewis JJ, Brennan MF. Analysis of the prognostic significance of microscopic margins in 2,084 localized primary adult soft tissue sarcomas. Ann Surg. 2002;235(3):424–34. https://doi.org/10.1097/00000658-200203000-00015.
Trovik CS, Bauer HC, Alvegård TA, et al. Surgical margins, local recurrence and metastasis in soft tissue sarcomas: 559 surgically-treated patients from the Scandinavian Sarcoma Group Register. Eur J Cancer Oxf Engl 1990. 2000;36(6):710–6. https://doi.org/10.1016/s0959-8049(99)00287-7.
Zagars GK, Ballo MT, Pisters PWT, et al. Prognostic factors for patients with localized soft-tissue sarcoma treated with conservation surgery and radiation therapy: an analysis of 1225 patients. Cancer. 2003;97(10):2530–43. https://doi.org/10.1002/cncr.11365.
Acknowledgements
We thank Mohammad Boshahri for his assistance in scoring the studies based on the NOS.
Funding
Nil.
Author information
Authors and Affiliations
Contributions
Concept and design of study: MEP, BCT, ZXL. Search strategy, selection of eligible studies, collating patient/study data: ZXL, TYG. Statistical analyses: TYG, BCT. Interpretation of data: ZXL, TYG, BCT, MEP. Manuscript drafting: ZXL, TYG, BCT, MEP. Supervision of study: BCT, MEP.
Authors’ information
N.A.
Corresponding authors
Ethics declarations
Ethics approval and consent to participate
N.A.
Consent for publication
N.A.
Competing interests
The authors declare no competing interests.
Additional information
Publisher’s Note
Springer Nature remains neutral with regard to jurisdictional claims in published maps and institutional affiliations.
Supplementary Information
Additional file 1: Fig. S1.
Funnel plot for publication bias.
Additional file 2: Fig. S2.
Funnel plot for publication bias after trim-and-fill method.
Additional file 3: Fig. S3.
Meta-regression: logHR of OS on year of publication.
Additional file 4: Fig. S4.
Meta-regression: logHR of DSS on year of publication.
Additional file 5: Fig. S5.
Meta-regression: logHR of OS on NOS scores.
Additional file 6: Fig. S6.
Meta-regression: logHR of DSS on NOS scores.
Rights and permissions
Open Access This article is licensed under a Creative Commons Attribution 4.0 International License, which permits use, sharing, adaptation, distribution and reproduction in any medium or format, as long as you give appropriate credit to the original author(s) and the source, provide a link to the Creative Commons licence, and indicate if changes were made. The images or other third party material in this article are included in the article's Creative Commons licence, unless indicated otherwise in a credit line to the material. If material is not included in the article's Creative Commons licence and your intended use is not permitted by statutory regulation or exceeds the permitted use, you will need to obtain permission directly from the copyright holder. To view a copy of this licence, visit http://creativecommons.org/licenses/by/4.0/. The Creative Commons Public Domain Dedication waiver (http://creativecommons.org/publicdomain/zero/1.0/) applies to the data made available in this article, unless otherwise stated in a credit line to the data.
About this article
Cite this article
Lim, Z., Gu, T.Y., Tai, B.C. et al. Survival outcomes of malignant peripheral nerve sheath tumors (MPNSTs) with and without neurofibromatosis type I (NF1): a meta-analysis. World J Surg Onc 22, 14 (2024). https://doi.org/10.1186/s12957-023-03296-z
Received:
Accepted:
Published:
DOI: https://doi.org/10.1186/s12957-023-03296-z