Abstract
Background
GABA, a key inhibitory neurotransmitter, has synaptic and extrasynaptic receptors on the postsynaptic neuron. Background GABA, which spills over from the synaptic cleft, acts on extrasynaptic delta subunit containing GABAA receptors. The role of extrasynaptic GABAergic input in migraine is unknown. We investigated the susceptibility to valid migraine-provoking substances with clinically relevant behavioral readouts in Genetic Absence Epilepsy of Rats Strasbourg (GAERS), in which the GABAergic tonus was altered. Subsequently, we screened relevant GABAergic mechanisms in Wistar rats by pharmacological means to identify the mechanisms.
Methods
Wistar and GAERS rats were administered nitroglycerin (10 mg/kg) or levcromakalim (1 mg/kg). Mechanical allodynia and photophobia were assessed using von Frey monofilaments and a dark-light box. Effects of GAT-1 blocker tiagabine (5 mg/kg), GABAB receptor agonist baclofen (2 mg/kg), synaptic GABAA receptor agonist diazepam (1 mg/kg), extrasynaptic GABAA receptor agonists gaboxadol (4 mg/kg), and muscimol (0.75 mg/kg), T-type calcium channel blocker ethosuximide (100 mg/kg) or synaptic GABAA receptor antagonist flumazenil (15 mg/kg) on levcromakalim-induced migraine phenotype were screened.
Results
Unlike Wistar rats, GAERS exhibited no reduction in mechanical pain thresholds or light aversion following nitroglycerin or levcromakalim injection. Ethosuximide did not reverse the resistant phenotype in GAERS, excluding the role of T-type calcium channel dysfunction in this phenomenon. Tiagabine prevented levcromakalim-induced mechanical allodynia in Wistar rats, suggesting a key role in enhanced GABA spillover. Baclofen did not alleviate mechanical allodynia. Diazepam failed to mitigate levcromakalim-induced migraine phenotype. Additionally, the resistant phenotype in GAERS was not affected by flumazenil. Extrasynaptic GABAA receptor agonists gaboxadol and muscimol inhibited periorbital allodynia in Wistar rats.
Conclusion
Our study introduced a rat strain resistant to migraine-provoking agents and signified a critical involvement of extrasynaptic δGABAergic receptors. Extrasynaptic δ GABAA receptors, by mediating constant background inhibition on the excitability of neurons, stand as a novel drug target with a therapeutic potential in migraine.
Graphical abstract

Similar content being viewed by others
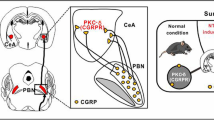
Introduction
Migraine headache is a leading cause of morbidity among the adult population worldwide. Attacks are characterized by unilateral, pulsating, and moderate to severe headaches lasting 4-72 hours. Migraine attacks can be provoked in clinical studies by numerous agents such as nitroglycerin (NTG), levcromakalim (LVC), and calcitonin gene-related peptide (CGRP) [1, 2]. These agents were also translated into animal models to better study the pathophysiology of the disease. NTG injection was shown to reduce periorbital mechanical thresholds and provoke anxiety-like behavior and light aversion [3,4,5]. Similarly, LVC induced periorbital allodynia, anxiety-like behavior, and increased sensitivity to light [5]. The LVC-induced migraine-like phenotype was evident as early as 30 minutes following the injection and completely resolved after 120 minutes in contrast to NTG, which caused a longer-lasting phenotype. LVC can strongly cause vasodilation by mechanistically opening ATP-gated potassium channels and recruiting CGRP signaling [6, 7]. Previous research suggests that LVC primarily acts on meningeal vessels and adjoined peripheral afferents [8].
Gamma-aminobutyric acid (GABA) is the primary inhibitory neurotransmitter in the central nervous system. GABA’s inhibitory effects are mediated by two types of receptors, GABAA and GABAB receptors [9]. GABAA receptors are ligand-gated ion channels composed of 5 subunits. So far, 19 different subunit isoforms have been described: six α (alpha1-6), three β (beta1-3), three γ (gamma1-3), three ρ (rho1-3), and one each of the δ (delta), ε (epsilon), π (pi), and θ (theta) [10]. GABA decreases the likelihood of action potential generation and neuronal excitability through phasic and tonic inhibition modes [11]. Phasic inhibition is constituted mainly through synaptic GABAA receptors composed of αβγ subunits and are briefly activated upon presynaptic GABA release. The presence of γ subunit anchors the receptor to the postsynaptic membrane where GABA is directly released [12]. Tonic inhibition is mediated by “spillover” of GABA, a specific fraction of GABA that escapes the synaptic cleft, and acts on extrasynaptic GABAA and GABAB receptors [11, 13].
Extrasynaptic GABAA receptors have a particular configuration, harboring δ instead of γ subunit. The presence of δ subunit (and lack of γ subunit) in the GABAA receptor (δGABAA receptors) results in high affinity towards even low concentrations of ambient GABA and slow dissociation kinetics, allowing significant neuromodulation and provides a balance between excitation and inhibition [14,15,16,17]. Tonic inhibition decreases the size and duration of an excitatory postsynaptic potential, which narrows the temporal and spatial window for signal integration and reduces the likelihood of action potential generation [11]. δGABAA receptors are found ubiquitously in nearly all parts of the central nervous and trigeminal systems [11, 15, 18,19,20,21,22]. Previous studies have demonstrated the expression of δGABAA receptors in all neuroglial cells [23, 24]. The role of the GABAergic system has been implicated in migraine headaches [25,26,27]. Tonic inhibitory input may reduce the excitability of central pain pathways responsible for migraine pain propagation [27]. Any of the previous studies did not evaluate the involvement of extrasynaptic δGABAA receptors in migraine. It is not established if extrasynaptic δGABAA receptor activity is implicated in migraine phenotype.
Genetic Absence, Epilepsy of Rats Strasbourg (GAERS), is a particular strain of rats associated with increased GABAergic tonus and T-type calcium channel dysfunction [28, 29]. GABAergic excess is not due to increased vesicular GABAergic release or over-expression of extrasynaptic GABAergic receptors but from decreased reuptake of GABA in the synaptic cleft [30, 31]. GABAB receptors also play a significant role in the GAERS phenotype [32]. Nonetheless, the role of GABAA receptors is controversial. Recent evidence implies that alterations in phasic and tonic GABAA receptor-related activity contribute to the absence of epilepsy phenotype in a cell- and region-specific manner in the central nervous system [31]. In the study of Çarçak et al., the paired-pulse depression paradigm was more pronounced in GAERS rats than in normal Wistar rats [33]. It is uncertain whether this GABAergic excess can affect the susceptibility to migraine-inducing substances. A further question is which components of this GABA overactivity can contribute to a possible alteration of migraine-like phenotype susceptibility.
We hypothesize that GAERS have altered susceptibility to migraine-provoking agents due to two fundamental mechanisms, namely T-type calcium channels and GABAergic dysfunction. In this study, we showed that acute NTG or LVC injection do not provoke a migraine-like phenotype in GAERS rats, and we aim to establish 1) the contribution of T-type calcium channels to GAERS resistance, 2) the imitation of GAERS-like response by manipulating the GABA reuptake and GABA receptors in Wistar rats.
Methods
Animals
All animal experiments were approved by the Hacettepe University Animal Experimentation Ethics Board (approval no: 2022/05–11) in compliance with ARRIVE guidelines. Male Wistar and GAERS rats (250-350 g) were used throughout the experiments. Wistar rats were purchased from Kobay, Türkiye, and GAERS rats were obtained from the breeding colony of Acıbadem University, Türkiye. Rats were housed in triplets on a 12/12 h light/dark schedule (lights on at 07:00 h) in a climate-controlled room with ad libitum chow and water. Rats were acclimatized for at least one week before being subject to any experimental procedure.
Experimental drugs and animal models of acute migraine
Acute migraine models are established by administering nitroglycerin (NTG – 10 mg/kg in 10% ethanol in saline) or levcromakalim (LVC – 1 mg/kg in 5% ethanol in saline) for a single time. Both drugs at the designated doses have been shown to induce an acute migraine-like phenotype in rats at the 120-minute and 60-minute time points, respectively [3, 5]. The vehicle for NTG and LVC in our experiments was ethanol in saline. All the information regarding experimental drugs and their administration is supplied in Table 1.
Experimental design
For von Frey testing and pain behavior experiments, Wistar and GAERS rats were randomly divided into three treatment groups each, totaling up to 6 groups: control (CTRL), nitroglycerin (NTG), levcromakalim (LVC) for Wistar rat groups; a control group for GAERS (GAERS), GAERS nitroglycerin (GAERS NTG), GAERS levcromakalim (GAERS LVC) for GAERS rat groups. Control groups were injected with vehicle (ethanol in saline).
Periorbital and hind paw mechanical thresholds were measured following the injection of NTG, LVC, or vehicle. Baseline measurements were annotated as “BL” in the figures. In the relevant timeframes specified above (120 minutes for NTG, 60 minutes for LVC), light aversion was assessed in vehicle-, NTG-, and LVC-injected Wistar and GAERS rats. Subsequently, the possible therapeutic effects of other pharmacological interventions were evaluated with von Frey filaments. Only one experimenter conducted von Frey experiments and was blinded to animal condition.
Determination of sample size
Sample sizes were determined by using G*power and resource equation [34, 35]. G-power analysis has retrieved nine animals per group for mechanical threshold testing and migraine-related light sensitivity experiment (α error: 0.05, β error: 0.8, effect size: 0.5). On the other hand, seven animals per group was calculated to be sufficient for both mechanical allodynia and light aversion tests from resource equation. Therefore, we have decided to use eight animals in each behavioral experiment. A total of 152 animals were used for all experiments.
Behavioral experiments
Mechanical allodynia
Von Frey monofilaments were used to measure periorbital and hind paw mechanical thresholds by the up-down method [36]. For hind paw threshold testing, rats were placed onto a flat wire mesh platform (Fig. 1A), and the experimental procedure was started after their exploratory behavior had ceased. Von Frey monofilaments were applied to the middle of the hind paw’s sole. Positive responses were characterized by paw withdrawal and paw licking [5, 37, 38] For periorbital testing, a large horizontal wire mesh tube apparatus (28 cm x 10 cm x 7 cm) was employed, as we used in our recent publications [5, 39] (Fig. 1B). The apparatus volume was five times larger than a standard restrainer device (15 cm x 4 cm x 6 cm) which allowed free movements without stress and animals never resisted to enter the apparatus in any of the trials. Animals were acclimated to handling and testing apparatus for five days (10 minutes per day) before the testing day [5, 39,40,41]. We began with a force of 2.0 grams and applied the filament perpendicularly to the midline of the forehead. Positive responses were identified as aversive head withdrawals, headshakes, vocalizations, ipsilateral head scratches, and whole-body retractions [42, 43]. To calculate the 50% withdrawal threshold, we utilized an online calculator accessible at https://bioapps.shinyapps.io/von_frey_app/ [44].
Illustration of the hind paw and periorbital sensory testing. A A rat is shown in the hind paw testing platform. Von Frey filament is applied to the mid-plantar area of the hind paw without touching the foot pads. B A rat is shown during periorbital testing in our apparatus, which allows rats to move and turn around. Von Frey filament is perpendicularly applied to the midline of the frontal area. A positive response was noted when the animal quickly withdrew its head from the filament
Light aversion
Light aversion was assessed using a dark-light box setup comprising two connected boxes: one brightly illuminated at 3000 lux and the other nearly completely dark [45]. Rats were allowed to explore both chambers freely during the test, and we recorded the duration they spent in the well-lit compartment and the count of transitions made from the dark area to the bright one over 15 minutes [46].
Statistical analysis
The results were not processed and were presented as raw data. The results are given as the mean ± standard error of the mean (SEM). Statistical analyses were conducted using GraphPad Prism software (GraphPad Software Inc., CA, USA). For data with a parametric distribution, we applied either Student’s t-test when comparing two groups or one-way or two-way analysis of variance (ANOVA) followed by the appropriate post hoc test when comparing three or more groups. Post hoc tests were not conducted in cases where the ANOVA F-values were not statistically significant. P < 0.05 was considered statistically significant.
Results
GAERS rats are resistant to acute mechanical allodynia induced by NTG or LVC injections
Ethanol in saline solutions was used as a vehicle in two different concentrations (5% and 10%) in our experiments. In each vehicle group, results from the von Frey testing and light aversion experiment were not statistically different; thereby, the results of the vehicle groups (5% and 10 % ethanol) were pooled. Wistar rats and GAERS rats had separate vehicle groups (n=8 per group).
Firstly, we compared the allodynic effects of acute NTG or LVC injections to Wistar or GAERS rats with their corresponding control groups. Acute LVC significantly reduced periorbital thresholds in Wistar rats at the 30-,60- and 90-min timepoints (F (1, 15) = 8.16, P < 0.05, Fig. 2A). NTG-administered Wistar rats also exhibited statistically significant periorbital allodynia at the 60- and 120-min time points (F (1, 17) = 4.6, P < 0.05, Fig. 2A). However, periorbital mechanical thresholds of LVC- or NTG-injected GAERS rats were similar to GAERS at all timepoints (F (1, 15) = 3.11, P > 0,05 and F (1, 14) = 0.54, P > 0.05, respectively, Fig. 2D).
Acute LVC and NTG do not provoke migraine-like phenotype in GAERS. A LVC and NTG induced statistically significant periorbital allodynia (Sidak’s multiple comparisons two-way ANOVA, P < 0.05). B LVC and NTG injections caused mechanical threshold reduction in the hind paw plantar area (Sidak’s multiple comparisons two-way ANOVA, P < 0.01 and P < 0.001, respectively). C LVC and NTG-injected rats exhibited light aversion behavior as evident from less time spent in the light compartment of the dark light box test (Student’s t-test, P < 0.05). GAERS rats did not display periorbital (D) or hind paw (E) allodynia following LVC or NTG injection (Sidak’s multiple comparisons two-way ANOVA, P > 0.05). F GAERS rats did not show aversion to light after LVC or NTG administration (Student’s t-test, P > 0.05) (n=8 per group) (*P < 0.05 LVC vs CTRL; ** P < 0.01 LVC vs. CTRL; *** P < 0.001 LVC vs. CTRL; # P < 0.05 NTG vs. CTRL; ## P < 0.01 NTG vs. CTRL; ### P < 0.001 NTG vs. CTRL)
Similarly, hind paw mechanical thresholds of LVC group were detected significantly lower at the 30- and 60-min timepoints and NTG injection yielded an extracephalic allodynic response at 60-, 120-, 180-min timepoints in Wistar rats (F (1, 19) = 8.24, P < 0.01 and F (1, 23) = 21.99, P < 0.001, respectively, Fig. 2B). In contrast, GAERS LVC rats displayed a partial hind paw mechanical threshold reduction only at the 30-min timepoint, but this did not reach statistical significance (F (1, 15) = 4.02, P > 0.05, Fig. 2E). GAERS NTG group did not display any statistically significant threshold difference in the hind paw region (F (1, 14) = 0.0006, P > 0.05, Fig. 2E). We have also tested the effects of vehicle injection on the mechanical thresholds of GAERS rats and found no statistically significant difference at any timepoint.
GAERS rats do not exhibit light aversion after NTG or LVC injections
We further conducted a dark-light box test to examine the resistance of GAERS phenotype to migraine-provoking substances. The behavioral testing was initiated when the lowest periorbital mechanical thresholds were detected (120 minutes for NTG and 30 minutes for LVC). GAERS rats underwent behavioral testing at these time points as well.
NTG- and LVC-injections provoked light aversion, as evident from less amount of time spent in the lightbox (P < 0.05, Fig. 2C). In contrast, GAERS NTG and GAERS LVC groups were not significantly different from GAERS (P > 0.05, Fig. 2F).
Resistance of GAERS is independent of T-type calcium channel dysfunction
After verifying the GAERS resistance in both NTG- and LVC-induced acute migraine models, we continued our experiments with LVC. LVC was shown to provoke migraine attacks with a higher likelihood than NTG in clinical studies [47]. Although NTG is a more commonly used migraine-provoking substance, it has a broad and non-specific pharmacological target profile, some of which may be irrelevant to migraine pathogenesis, such as direct nitrergic action on the neuroglial system. On the other hand, LVC is an ATP-gated potassium channel opener, a more relevant and pathophysiologically better-characterized target concerning migraine phenotype [48]. Therefore, we decided that LVC was a better option for our study.
At first, we wanted to demonstrate if this resistance was caused by increased T-type calcium channel conductance. We blocked these channels by administering ethosuximide (100 mg/kg). GAERS rats, when injected LVC together with 100 mg/kg of ethosuximide, still had comparable mechanical thresholds with the GAERS group and did not display cephalic or extracephalic pain sensitivity (F (1, 9) = 3.26, P > 0.05, Fig. 3A and F (1, 9) = 4.79, P > 0.05, Fig. 3B respectively). As we did not detect any difference at 30-min and 60-min, we did not test further for 180- and 240-min time points.
100 mg/kg ethosuximide does not reverse LVC-induced migraine-like phenotype resistance in GAERS. 100 mg/kg ethosuximide was administered to GAERS along with LVC to explore if T-type Ca channel overactivity would reverse the pain phenotype resistance in periorbital (A) and hind paw (B) areas. No change was detected at 30-min and 60-min time points; 90-min, 180-min, and 240-min tests were not performed (Sidak’s multiple comparisons two-way ANOVA, P > 0.05, n = 8 per group)
Thus, it is inferred that the resistance of GAERS was not due to the T-type calcium channel dysfunction.
After observing that GAERS resistance is not a direct/indirect result of T-type calcium channel dysfunction, we decided to investigate whether the GABAergic system perturbations cause the pain-resistance phenotype. As stated above, dysfunctions in the GABAergic system are speculated to be trifold: 1) GAT-1 underactivity, 2) GABAB receptor overactivity, and 3) GABAA receptor dysfunction. In the following experiments, we imitated these dysfunctions in the normal Wistar rats to better grasp the mechanism of migraine-like pain resistance.
GAT-1 antagonist tiagabine blocks mechanical allodynia in Wistar rats
At first, we wanted to evaluate the role of increased GABA concentrations in the synaptic cleft. To increase the synaptic GABA concentrations and to imitate the GAERS phenotype, we used tiagabine to block GAT-1 transporters. Tiagabine injection fully ameliorated the LVC-provoked mechanical allodynia both in periorbital and hind paw regions (F (1, 14) = 5.51, P < 0.05, Fig. 4A and F (1, 17) = 6.54, P < 0.05, Fig. 4B, respectively).
GAT-1 inhibitor tiagabine ameliorated LVC-induced periorbital and hind paw allodynia in Wistar rats. Wistar rats were injected with 5 mg/kg tiagabine and 1 mg/kg LVC. We have explored if mimicking GAT-1 overactivity in Wistar rats could prevent the mechanical threshold reduction. Tiagabine injection prevented periorbital (A) and hind paw (B) allodynia in LVC-administered Wistar rats (Sidak’s multiple comparisons two-way ANOVA, P < 0.05, n = 8 per group) (*P < 0.05 LVC vs CTRL; ** P < 0.01 LVC vs. CTRL; *** P < 0.001 LVC vs. CTRL; ✝ P < 0.05 LVC vs. treatment; ✝✝ P < 0.05 LVC vs. treatment)
Regarding how increased GABA concentrations block the pain sensitivity response, we have hypothesized that increased GABA in the synaptic cleft should act on the postsynaptic receptors, namely GABAA and GABAB receptors. In the following experiments, we used various pharmacological interventions to delineate the receptor type further.
GABAB receptor agonist baclofen does not mitigate acute mechanical allodynia in Wistar rats
To assess the role of GABAB receptor overactivity, we administered baclofen and LVC to Wistar rats. Thereby, we wanted to evaluate if GABAB receptor agonism can alleviate LVC-induced pain sensitivity. Baclofen-injected rats still exhibited as much periorbital and hind paw allodynia when compared to the LVC group (F (1, 11) = 0.80, P > 0.05, Fig. 5A and F (1, 14) = 0.39, P > 0.05, Fig. 5B, respectively).
Synaptic GABAAR agonist diazepam does not ameliorate mechanical allodynia in Wistar rats
The role of synaptic GABAA receptors was evaluated with diazepam. Diazepam administration did not prevent LVC-induced periorbital and hind paw tactile sensitivity (F (1, 10) = 1.37, P > 0.05, Fig. 5C and F (1, 13) = 0.41, P > 0.05, Fig. 5D, respectively).
Synaptic GABAA receptor antagonist flumazenil does not negate the GAERS resistance
To further validate that synaptic GABAA receptors do not participate in GAERS resistance, we administered flumazenil with LVC to GAERS rats. Flumazenil- and LVC-injected GAERS rats did not exhibit periorbital and hind paw pain sensitivity when compared to GAERS rats (F (1, 10) = 0.06, P > 0.05, Fig. 5E and F (1, 10) = 0.98, P > 0.05, Fig. 5F, respectively).
GABAB and synaptic GABAA receptors do not significantly mediate GAERS resistance to LVC and NTG. GABAB receptor agonist baclofen (2 mg/kg) (A, B) or synaptic GABAA receptor agonist diazepam (1 mg/kg) (C, D) were given to Wistar rats right after LVC administration. None blocked periorbital or hind paw allodynia in LVC-injected Wistar rats (Sidak’s multiple comparisons two-way ANOVA, P > 0.05, n = 8 per group). In line with the diazepam data, synaptic GABAA receptor antagonist flumazenil (10 mg/kg) (E, F) did not reverse the resistance when given together with LVC to GAERS (Sidak’s multiple comparisons two-way ANOVA, P > 0.05, n = 8 per group). Statistical significances between CTRL and LVC groups were not marked on the graphs where no significant treatment effect between LVC and treatment groups was detected
Extrasynaptic GABAA receptor agonists gaboxadol and muscimol block mechanical allodynia
Finally, we wanted to explore the contribution of extrasynaptic GABAA receptors to migraine-like phenotype resistance. Gaboxadol and muscimol are well-known agonists of extrasynaptic GABAA receptors expressing delta subunit (δGABAA receptors), so we proceeded with these agents. As a result, gaboxadol fully reversed the mechanical allodynia in the periorbital area (F (1, 13) = 9.59, P < 0.01, Fig. 6A). It has also ameliorated the hind paw allodynia in the 60-min timepoint. Still, without any effect at the 30-min timepoint (F (1, 16) = 5.03, P < 0.05, Fig. 6B). On the other hand, muscimol entirely blocked the periorbital allodynia (F (1, 13) = 16.55, P < 0.01, Fig. 6C) with no effect on hind paw allodynia at any of the timepoints tested (F (1, 16) = 0.002, P > 0.05, Fig. 6D).
Extrasynaptic GABAA receptor agonists relieved mechanical allodynia in acute LVC-induced migraine model in Wistar rats. Gaboxadol (4 mg/kg) and muscimol (0.75 mg/kg) entirely prevented LVC-induced periorbital allodynia (A, C) (Sidak’s multiple comparisons two-way ANOVA, P < 0.01, n = 8 per group). On the other hand, gaboxadol provided only partial benefit for LVC-induced extracephalic hypersensitivity (B), whereas muscimol had no effect (D) (Sidak’s multiple comparisons two-way ANOVA, P < 0.05 and Sidak’s multiple comparisons two-way ANOVA, P > 0.05, respectively, n = 8 per group) (*P < 0.05 LVC vs CTRL; ** P < 0.01 LVC vs. CTRL; *** P < 0.001 LVC vs. CTRL; ✝ P < 0.05 LVC vs. treatment; ✝✝ P < 0.01 LVC vs. treatment; ✝✝✝ P < 0.001 LVC vs. treatment)
Discussion
Our study has revealed several novel findings: GAERS rats show resistance to NTG- and LVC-provoked acute migraine models. With this, we described for the first time an animal model that is not affected by the acute injection of two well-established migraine-provoking substances, NTG and LVC. In addition, resistance was not linked to T-type channel dysfunction and the resultant paroxysmal spike-and-wave discharges, implying the involvement of another, more fundamental mechanism. In our study, we focused on the GABAergic dysfunction. Therefore, we pharmacologically imitated the GABAergic dysfunctions of GAERS in Wistar rats step-by-step. As a result, we revealed that GAT-1 blockage and δGABAA receptor agonism successfully alleviated the allodynia in LVC-injected Wistar rats. Our key findings and hypothesis are schematized in Fig. 7.
Schematic illustration of extrasynaptic δGABAA receptors in nociceptive neuron determining mechanical pain thresholds. Extrasynaptic δGABAA receptors mediate constant background inhibition on the postsynaptic nociceptive neurons, thereby modulating pain thresholds. Direct activation of δGABAA receptors by agonist drugs or indirect activation via increased spillover GABA due to GAT-1 (GABA transporter-1) inhibition may block acute migraine attacks
Periorbital mechanical allodynia and hind paw allodynia are frequently used to measure migraine-like phenotype in rodents [49]. We found that NTG- or LVC-administered GAERS rats were completely free from the periorbital allodynia-inducing effects of these substances at all the time points tested. Despite a slight threshold reduction in the hind paw region upon LVC administration, it did not reach statistical significance. We further verified the resistance by assessing the presence (or absence of) light aversion. Likewise, light aversion was absent in NTG- or LVC-injected GAERS rats compared to the GAERS group, which supported the mechanical threshold experiments.
One of the most well-known characteristics of GAERS rats is that they have overactive T-type calcium channels, which also drive brief spike-and-wave discharges [50]. A simultaneous interruption of consciousness, lasting 5-10 seconds, accompanies spike-and-wave discharges. These spike-and-wave discharges arise during passive wakefulness and are not in an alert state [51]. GAERS rats actively moved in the testing apparatus during behavioral experiments and exhibited exploratory behavior, signifying a fully awake state. In addition, it is already known that spike-and-wave discharges terminate upon an unexpected sensory stimulus [52]. Our preliminary electrocorticographic experiments also showed that spike-and-wave discharges were aborted by finger snapping, clapping, and von Frey filament application (data not shown). Therefore, spike-and-wave discharges (and resultant impaired consciousness) were not a likely confounding factor in our experiments. To entirely exclude any confounding effect of impaired T-type calcium channels and spike-and-wave discharges, we administered ethosuximide at 100 mg/kg to GAERS rats to 1) silence overactive T-type calcium channels, 2) inhibit resultant spike-and-wave discharges and accompanying short-lasting interruption of consciousness. Despite being used clinically, valproate was not appropriate in our experiments due to its wide range of pharmacological targets, which might confound our results. Ethosuximide is an absence epilepsy drug without therapeutic potential in migraine patients, which makes it suitable for our experiments. In our experiments, 100 mg/kg ethosuximide had successfully blocked all spike-and-wave discharges for approximately 2 hours in GAERS electrocorticographic recordings (data were not shown). Subsequently, we administered LVC to GAERS rats to see if they would exhibit increased pain sensitivity. We did not detect any allodynic responses in ethosuximide + LVC-injected GAERS rats, implying that T-type calcium channel dysfunction and spike-and-wave discharges do not contribute to migraine-like phenotype-resistance of GAERS. Notably, tiagabine, gaboxadol, baclofen, and muscimol are capable of aggravating spike-and-wave discharges at the given doses [32, 53, 54]. However, only tiagabine, gaboxadol, and muscimol imitated the GAERS resistance in Wistar rats. This further supports the notion that migraine-like phenotype resistance in GAERS is independent of spike-and-wave discharges.
GABAergic dysfunction is already an established phenomenon in GAERS. Increased tonic inhibitory mechanisms are thought to underlie the GAERS phenotype [31]. GAT-1 loss-of-function has already been demonstrated in GAERS, contributing to the increased inhibitory tonus [28]. To understand if the same mechanism is the causative factor of the resistance, we administered tiagabine to see if it can improve LVC-induced mechanical allodynia. Tiagabine entirely prevented the LVC-induced cephalic and extracephalic allodynia. The tiagabine dose in our study was 5 mg/kg, a dose considerably below 15 mg/kg, which was reported to cause impaired locomotion [55]. In another study, 4,5 mg/kg tiagabine did not alter the total distance traveled in the open field arena test [56]. On the bedside, there are only two studies that investigated the therapeutic potential of tiagabine in migraineurs [57]. In one of the studies, 92% of the patients experienced a reduction in their migraine headache frequency [58]. However, no official clinical trial has been done so far.
After observing that GAT-1 inhibition abolished LVC-induced pain withdrawal responses, we speculated that increased GABA in the synaptic cleft should have an inhibitory effect on nociceptive neurons through their post-synaptic receptors with a “spillover mechanism.” GABAB receptors are shown to be activated by the spillover of GABA [59]. Therefore, we hypothesized that overactivity of GABAB receptors, an essential mediator of inhibitory tonus, could explain the resistance [60, 61]. Within the context of migraine, baclofen was reported to reduce headache frequency, duration, and intensity in 86,2% of patients [62]. However, baclofen is not used to treat migraine headaches. The analgesic effect of baclofen in humans is very limited [63, 64]. Our study did not detect any improvement in allodynic phenotype induced by LVC in Wistar rats. We have ascertained that the baclofen dose (2 mg/kg) was the safest maximum dose, as higher doses were significantly associated with increased sedation/decreased locomotion [65, 66]. In support of our study, 2-hydroxysaclofen did not reverse the inhibitory action of GABA on thalamic neurons, implying GABAergic tonus may primarily act through extrasynaptic GABAA receptors in ventral posteromedial thalamic neurons [67]. Furthermore, it was shown that plasma protein extravasation in a substance P-induced headache model does not involve GABAB receptors [68]. The lack of an antiallodynic effect of baclofen in our study’s LVC-induced acute migraine model can be attributed to the variations in baclofen dosage. Baclofen has a dose-dependent and divergent effect profile in a tonic-clonic epilepsy model, where a low dose of baclofen (1 mg/kg) was found to exacerbate epileptic discharges via inhibition of presynaptic GABAergic neurotransmitter release [69]. Several rodent studies demonstrate baclofen’s analgesic effects [66, 70]. These studies evaluated analgesia with a hot plate test (general thermal nociception) and formalin test (inflammatory pain). On the other hand, migraine models are accepted to be “constellation” pain models, belonging neither to inflammatory nor neuropathic categories [71]. This distinction may underlie why baclofen was ineffective in our acute migraine model. A third reason is the administration route. Many studies used the intrathecal route to administer BAC, which may have a different dose-effect relationship [63, 64, 72, 73]. In our acute migraine model, we have administered 2 mg/kg baclofen through the intraperitoneal route, which is accepted to be more physiological than intrathecal administration. It should also be considered that GABAB receptor’s presence in the trigeminal pain system may not translate to antiallodynic activity. In conclusion, GABAB receptors do not alleviate allodynia induced by LVC, which posits that GABAB receptors may not play a role in GAERS resistance.
Then, we tested for synaptic GABAergic mechanisms by using diazepam and flumazenil. Diazepam is not indicated for migraine attacks or prophylaxis. Sedation and dependence are major limitations for diazepam use [74]. In our preliminary experiments, more than 1 mg/kg diazepam caused behavioral impairment (decreased vigilance and increased sedation) that was incompatible with the further experiments. A 1 mg/kg dose was still effective in behavioral models other than migraines [75]. Diazepam failed to block the LVC-induced mechanical allodynia in normal Wistar rats. To validate that synaptic GABAA receptors do not mediate the resistance in GAERS, we have also administered flumazenil and LVC to GAERS rats. Flumazenil was given subcutaneously to extend the half-life of the drug, as it has a very short half-life when given through other routes [76, 77]. We did not observe any reduction in mechanical thresholds after flumazenil and LVC administration to GAERS rats, further verifying our results. Synaptic GABAA receptors are the primary mediators of phasic inhibition [11]. Phasic inhibition is a short-lasting hyperpolarization that generates and sustains the oscillatory rhythms in neural circuits. On the other hand, tonic inhibition increases the membrane conductance, decreases the likelihood of action potential generation, and creates an input-filtering effect [11, 78]. Ataka et al. reported that tonic inhibitory currents carried more charge than phasic currents in lamina II of the spinal cord, implying tonic currents are more prominent in sensory processing [79].
Extrasynaptic δGABAA receptors are present in all regions of the peripheral and central pain matrix [19,20,21, 80,81,82]. Pyramidal neurons, interneurons, and glial cells are shown to express extrasynaptic δGABAA receptors, which contribute to tonic inhibition [83,84,85]. Therefore, we investigated the role of extrasynaptic δGABAA receptors. δGABAAR agonists gaboxadol and muscimol inhibited the periorbital pain response in LVC-injected Wistar rats. The dose of gaboxadol in our study was much lower than the ataxic 10 mg/kg dose [86, 87]. Muscimol dose was deliberately chosen as 0,75 mg/kg from preliminary data due to higher doses being associated with increased sedation. Gaboxadol entirely blocked LVC-induced periorbital allodynia but did not reverse the hind paw allodynia at 30 minutes. This was remarkably comparable with the GAERS pattern after the LVC administration. This pattern differs from tiagabine’s, which successfully prevented LVC-induced cephalic and extracephalic allodynia at all time points. This may suggest the contribution of different GABAergic receptors other than δGABAA receptors in the case of tiagabine. A primary reason why we did not detect such a contribution could be attributed to the fact that we conducted our experiments with doses suitable and compatible with von Frey testing. This necessitated meticulously chosen doses that would not hamper locomotion, cause sedation, or decrease vigilance. Higher doses of GABAB receptors and synaptic GABAA receptor agonists (baclofen and diazepam) might have been beneficial regarding pain phenotype. Still, these higher doses were not compatible with von Frey testing.
As for muscimol, periorbital mechanical threshold reduction was abolished entirely, yet hind paw threshold reduction was not alleviated. So far, this is the first time a divergence between periorbital and hind paw thresholds has been seen in an acute migraine model. The previous studies provide only a few insights, and further investigations are necessary to explain this phenomenon. In our research, muscimol did not alleviate hind paw allodynia, whereas gaboxadol had a partial antiallodynic effect. Although both are classified as δGABAA receptor agonists, gaboxadol also had agonistic effects on α1- and α2-containing synaptic GABAA receptors [88, 89]. On the other hand, no such information exists for muscimol, which could explain its total lack of effect on extracephalic hypersensitivity. However, both successfully blocked LVC-induced periorbital allodynia, a more direct readout of migraine-related cephalic sensitivity [44, 90]. No clinical study has evaluated the therapeutic potential of gaboxadol and muscimol in migraine headaches. The agents, their mechanisms of action, the aims, and the resultant effects are summarized in Table 2.
Our study presents some limitations. This study cannot claim to explain the full mechanism of resistance in GAERS. Rather, it introduces a novel drug target with a therapeutic potential for migraine attacks. Further studies should focus on the dose-response relationship, side effect profile, and prophylactic potential of tiagabine, gaboxadol, and muscimol within the context of migraine treatment. It is also not established how the contribution of the extrasynaptic GABAergic mechanisms relates to vascular, neuroglial, and dural aspects of migraine pathophysiology. In addition, it is still unknown whether GAERS are also resistant to chronic migraine or medication-overuse headache models, which may have a different pathogenetic origin. Additionally, we have used only male rats for the sake of comparability with the previous research. Future studies should include a female rat group as well.
In conclusion, our study introduced extrasynaptic GABAA receptors as a novel drug target within the context of migraine research and further cemented the role of tonic GABAergic inhibition on the pain matrix. We have also described for the first time a migraine phenotype-resistant rat strain, which could pave the way for the development of other novel therapeutic avenues. Future investigations are still necessary as to the machinery and the role of δGABAA receptors in pain phenotype resistance.
Availability of data and materials
Data will be made available upon reasonable request.
Abbreviations
- NTG:
-
Nitroglycerin
- LVC:
-
Levcromakalim
- GAERS:
-
Genetic Absence Epilepsy of Rats Strasbourg
- CTRL:
-
Control
- SEM:
-
Standard error of the mean
- GAT-1:
-
GABA transporter 1
References
Ashina M, Hansen JM, Olesen J (2013) Pearls and pitfalls in human pharmacological models of migraine: 30 years’ experience. Cephalalgia. 33(8):540–53
Al-Karagholi MA, Ghanizada H, Nielsen CAW, Hougaard A, Ashina M (2021) Opening of ATP sensitive potassium channels causes migraine attacks with aura. Brain. 144(8):2322–32
Bates EA, Nikai T, Brennan KC, Fu YH, Charles AC, Basbaum AI et al (2010) Sumatriptan alleviates nitroglycerin-induced mechanical and thermal allodynia in mice. Cephalalgia. 30(2):170–8
Akerman S, Romero-Reyes M, Karsan N, Bose P, Hoffmann JR, Holland PR et al (2021) Therapeutic targeting of nitroglycerin-mediated trigeminovascular neuronal hypersensitivity predicts clinical outcomes of migraine abortives. Pain. 162(5):1567–77
Alpay B, Cimen B, Akaydin E, Bolay H, Sara Y (2023) Levcromakalim provokes an acute rapid-onset migraine-like phenotype without inducing cortical spreading depolarization. J Headache Pain. 24(1):93
Ashina M, Terwindt GM, Al-Karagholi MA, de Boer I, Lee MJ, Hay DL et al (2021) Migraine: disease characterisation, biomarkers, and precision medicine. Lancet. 397(10283):1496–504
Christensen SL, Rasmussen RH, Ernstsen C, La Cour S, David A, Chaker J et al (2021) CGRP-dependent signalling pathways involved in mouse models of GTN- cilostazol- and levcromakalim-induced migraine. Cephalalgia. 41(14):1413–26
Christensen SL, Rasmussen RH, Cour S, Ernstsen C, Hansen TF, Kogelman LJ et al (2022) Smooth muscle ATP-sensitive potassium channels mediate migraine-relevant hypersensitivity in mouse models. Cephalalgia. 42(2):93–107
Simeone TA, Donevan SD, Rho JM (2003) Molecular biology and ontogeny of gamma-aminobutyric acid (GABA) receptors in the mammalian central nervous system. J Child Neurol. 18(1):39–48. discussion 9
Ghit A, Assal D, Al-Shami AS, Hussein DEE (2021) GABA(A) receptors: structure, function, pharmacology, and related disorders. J Genet Eng Biotechnol. 19(1):123
Farrant M, Nusser Z (2005) Variations on an inhibitory theme: phasic and tonic activation of GABA(A) receptors. Nat Rev Neurosci. 6(3):215–29
Essrich C, Lorez M, Benson JA, Fritschy JM, Luscher B (1998) Postsynaptic clustering of major GABAA receptor subtypes requires the gamma 2 subunit and gephyrin. Nat Neurosci. 1(7):563–71
Wang H, Kuo Z, Fu Y, Chen R, Min M, Yang H (2015) GABAB receptor-mediated tonic inhibition regulates the spontaneous firing of locus coeruleus neurons in developing rats and in citalopram-treated rats. J Physiol. 593(1):161–80
Belelli D, Harrison NL, Maguire J, Macdonald RL, Walker MC, Cope DW (2009) Extrasynaptic GABAA receptors: form, pharmacology, and function. J Neurosci. 29(41):12757–63
Cope DW, Hughes SW, Crunelli V (2005) GABAA receptor-mediated tonic inhibition in thalamic neurons. J Neurosci. 25(50):11553–63
Bianchi MT, Macdonald RL (2002) Slow phases of GABA(A) receptor desensitization: structural determinants and possible relevance for synaptic function. J Physiol. 544(Pt 1):3–18
Brown N, Kerby J, Bonnert TP, Whiting PJ, Wafford KA (2002) Pharmacological characterization of a novel cell line expressing human alpha(4)beta(3)delta GABA(A) receptors. Br J Pharmacol. 136(7):965–74
Rudolph S, Guo C, Pashkovski SL, Osorno T, Gillis WF, Krauss JM et al (2020) Cerebellum-specific deletion of the GABA(A) receptor delta subunit leads to sex-specific disruption of behavior. Cell Rep. 33(5):108338
Ye Z, McGee TP, Houston CM, Brickley SG (2013) The contribution of delta subunit-containing GABAA receptors to phasic and tonic conductance changes in cerebellum, thalamus and neocortex. Front Neural Circuits. 7:203
Marowsky A, Vogt KE (2014) Delta-subunit-containing GABAA-receptors mediate tonic inhibition in paracapsular cells of the mouse amygdala. Front Neural Circuits. 8:27
Hortnagl H, Tasan RO, Wieselthaler A, Kirchmair E, Sieghart W, Sperk G (2013) Patterns of mRNA and protein expression for 12 GABAA receptor subunits in the mouse brain. Neuroscience. 236:345–72
Griffiths JL, Lovick TA (2005) GABAergic neurones in the rat periaqueductal grey matter express alpha4, beta1 and delta GABAA receptor subunits: plasticity of expression during the estrous cycle. Neuroscience. 136(2):457–66
Peng Z, Huang CS, Stell BM, Mody I, Houser CR (2004) Altered expression of the delta subunit of the GABAA receptor in a mouse model of temporal lobe epilepsy. J Neurosci. 24(39):8629–39
Huang TH, Lin YS, Hsiao CW, Wang LY, Ajibola MI, Abdulmajeed WI et al (2023) Differential expression of GABA(A) receptor subunits delta and alpha6 mediates tonic inhibition in parvalbumin and somatostatin interneurons in the mouse hippocampus. Front Cell Neurosci. 17:1146278
Tzeng HR, Lee MT, Fan PC, Knutson DE, Lai TH, Sieghart W et al (2021) alpha6GABA(A) Receptor positive modulators alleviate migraine-like grimaces in mice via compensating gabaergic deficits in trigeminal ganglia. Neurotherapeutics. 18(1):569–85
Cornelison LE, Woodman SE, Durham PL (2020) Inhibition of trigeminal nociception by non-invasive vagus nerve stimulation: investigating the role of GABAergic and serotonergic pathways in a Model of Episodic Migraine. Front Neurol. 11:146
D’Andrea G, Granella F, Cataldini M, Verdelli F, Balbi T (2001) GABA and glutamate in migraine. J Headache Pain. 2(1):s57–s60
Pirttimaki T, Parri HR, Crunelli V (2013) Astrocytic GABA transporter GAT-1 dysfunction in experimental absence seizures. J Physiol. 591(4):823–33
Cain SM, Tyson JR, Choi HB, Ko R, Lin PJC, LeDue JM et al (2018) Ca(V) 3.2 drives sustained burst-firing, which is critical for absence seizure propagation in reticular thalamic neurons. Epilepsia 59(4):778–91
Cope DW, Di Giovanni G, Fyson SJ, Orban G, Errington AC, Lorincz ML et al (2009) Enhanced tonic GABAA inhibition in typical absence epilepsy. Nat Med. 15(12):1392–8
Crunelli V, Leresche N, Cope DW. (2012) GABA-A Receptor Function in Typical Absence Seizures. In: Noebels JL, Avoli M, Rogawski MA, Olsen RW, Delgado-Escueta AV, editors. Jasper’s Basic Mechanisms of the Epilepsies. 4th ed. Bethesda (MD).
Marescaux C, Vergnes M, Bernasconi R (1992) GABAB receptor antagonists: potential new anti-absence drugs. J Neural Transm Suppl. 35:179–88
Carcak N, Dilekoz E, Gulhan R, Onur R, Onat FY, Sara Y (2016) Increased inhibitory synaptic activity in the hippocampus (CA1) of genetic absence epilepsy rats: Relevance of kindling resistance. Epilepsy Res. 126:70–7
Festing MF, Altman DG (2002) Guidelines for the design and statistical analysis of experiments using laboratory animals. ILAR J. 43(4):244–58
Festing MF (2006) Design and statistical methods in studies using animal models of development. ILAR J. 47(1):5–14
Chaplan S, Bach F, Pogrel J, Chung J, Yaksh T (1994) Quantitative assessment of tactile allodynia in the rat paw. J Neurosci Methods. 53(1):55–63
Vuralli D, Wattiez AS, Russo AF, Bolay H (2019) Behavioral and cognitive animal models in headache research. J Headache Pain. 20(1):11
Özsoylar Ö, Akçal D, Çizmeci P, Babacan A, Cahana A, Bolay H (2008) Percutaneous Pulsed Radiofrequency Reduces Mechanical Allodynia in a Neuropathic Pain Model. Anesth Analg. 107(4):1406–11
Dağıdır HG, Topa E, Vuralli D, Bolay H (2023) Medication overuse headache is associated with elevated lipopolysaccharide binding protein and pro-inflammatory molecules in the bloodstream. J Headache Pain. 24(1):150
Oshinsky ML, Gomonchareonsiri S (2007) Episodic Dural Stimulation in Awake Rats: A Model for Recurrent Headache. Headache. 47(7):1026–36
Elliott MB, Oshinsky ML, Amenta PS, Awe OO, Jallo JI (2012) Nociceptive Neuropeptide Increases and Periorbital Allodynia in a Model of Traumatic Brain Injury. Headache. 52(6):966–84
De Logu F, Landini L, Janal MN, Li Puma S, De Cesaris F, Geppetti P et al (2019) Migraine-provoking substances evoke periorbital allodynia in mice. J Headache Pain. 20(1):18
Vuralli D, Arslan B, Topa E, de Morais AL, Gulbahar O, Ayata C et al (2022) Migraine susceptibility is modulated by food triggers and analgesic overuse via sulfotransferase inhibition. J Headache Pain. 23(1):36
Christensen SL, Hansen RB, Storm MA, Olesen J, Hansen TF, Ossipov M et al (2020) Von Frey testing revisited: Provision of an online algorithm for improved accuracy of 50% thresholds. Eur J Pain. 24(4):783–90
Askari-Zahabi K, Abbasnejad M, Kooshki R, Raoof M, Esmaeili-Mahani S, Pourrahimi AM et al (2022) The role of basolateral amygdala orexin 1 receptors on the modulation of pain and psychosocial deficits in nitroglycerin-induced migraine model in adult male rats. Korean J Pain. 35(1):22–32
Farajdokht F, Babri S, Karimi P, Alipour MR, Bughchechi R, Mohaddes G (2017) Chronic ghrelin treatment reduced photophobia and anxiety-like behaviors in nitroglycerin- induced migraine: role of pituitary adenylate cyclase-activating polypeptide. Eur J Neurosci. 45(6):763–72
Al-Hassany L, Boucherie DM, Creeney H, van Drie RWA, Farham F, Favaretto S et al (2023) Future targets for migraine treatment beyond CGRP. J Headache Pain. 24(1):76
Clement A, Christensen SL, Jansen-Olesen I, Olesen J, Guo S (2023) The ATP sensitive potassium channel (K(ATP)) is a novel target for migraine drug development. Front Mol Neurosci. 16:1182515
Deuis JR, Dvorakova LS, Vetter I (2017) Methods Used to Evaluate Pain Behaviors in Rodents. Front Mol Neurosci. 10:284
Tsakiridou E, Bertollini L, de Curtis M, Avanzini G, Pape HC (1995) Selective increase in T-type calcium conductance of reticular thalamic neurons in a rat model of absence epilepsy. J Neurosci. 15(4):3110–7
Lannes B, Micheletti G, Vergnes M, Marescaux C, Depaulis A, Warter JM (1988) Relationship between spike-wave discharges and vigilance levels in rats with spontaneous petit mal-like epilepsy. Neurosci Lett. 94(1–2):187–91
Kozak G, Foldi T, Berenyi A. (2020) Spike-and-wave discharges are not pathological sleep spindles, network-level aspects of age-dependent absence seizure development in rats. eNeuro. 7(1).
Coenen AM, Blezer EH, van Luijtelaar EL (1995) Effects of the GABA-uptake inhibitor tiagabine on electroencephalogram, spike-wave discharges and behaviour of rats. Epilepsy Res. 21(2):89–94
Riban V, Heulard I, Reversat L, Si Hocine H, Verleye M (2022) Stiripentol inhibits spike-and-wave discharges in animal models of absence seizures: A new mechanism of action involving T-type calcium channels. Epilepsia. 63(5):1200–10
Nielsen EB, Suzdak PD, Andersen KE, Knutsen LJ, Sonnewald U, Braestrup C (1991) Characterization of tiagabine (NO-328), a new potent and selective GABA uptake inhibitor. Eur J Pharmacol. 196(3):257–66
Schmitt U, Luddens H, Hiemke C (2000) Behavioral effects of GABA(A) receptor stimulation and GABA-transporter inhibition. Pharmacol Biochem Behav. 65(2):351–6
Krymchantowski AV, Bigal ME, Moreira PF (2002) New and emerging prophylactic agents for migraine. CNS Drugs. 16(9):611–34
Drake M, Kay A, Knapp M, Greathouse NJ. (1999) An open-label trial of tiagabine for migraine prophylaxis. Headache, 39:352.
Scanziani M (2000) GABA spillover activates postsynaptic GABA(B) receptors to control rhythmic hippocampal activity. Neuron. 25(3):673–81
Princivalle AP, Richards DA, Duncan JS, Spreafico R, Bowery NG (2003) Modification of GABA(B1) and GABA(B2) receptor subunits in the somatosensory cerebral cortex and thalamus of rats with absence seizures (GAERS). Epilepsy Res. 55(1–2):39–51
Han HA, Cortez MA, Snead OC, III. (2012) GABA(B) Receptor and Absence Epilepsy. In: Noebels JL, Avoli M, Rogawski MA, Olsen RW, Delgado-Escueta AV, editors. Jasper’s Basic Mechanisms of the Epilepsies. 4th ed. Bethesda (MD).
Hering-Hanit R (1999) Baclofen for prevention of migraine. Cephalalgia. 19(6):589–91
Zuniga RE, Schlicht CR, Abram SE (2000) Intrathecal baclofen is analgesic in patients with chronic pain. Anesthesiology. 92(3):876–80
Slonimski M, Abram SE, Zuniga RE (2004) Intrathecal baclofen in pain management. Reg Anesth Pain Med. 29(3):269–76
Paredes R, Agmo A (1989) Stereospecific actions of baclofen on sociosexual behavior, locomotor activity and motor execution. Psychopharmacology (Berl). 97(3):358–64
Balerio GN, Rubio MC (2002) Baclofen analgesia: involvement of the GABAergic system. Pharmacol Res. 46(3):281–6
Andreou AP, Shields KG, Goadsby PJ (2010) GABA and valproate modulate trigeminovascular nociceptive transmission in the thalamus. Neurobiol Dis. 37(2):314–23
Han JK, Park MK, Park KW, Lee DH (1998). The Actions of Sodium Valproate in Headache model Evoked by Substance-P in rats. Journal of the Korean Neurological Association, 1-7.
Dugladze T, Maziashvili N, Borgers C, Gurgenidze S, Haussler U, Winkelmann A et al (2013) GABA(B) autoreceptor-mediated cell type-specific reduction of inhibition in epileptic mice. Proc Natl Acad Sci U S A. 110(37):15073–8
Shafizadeh M, Semnanian S, Zarrindast MR, Hashemi B (1997) Involvement of GABAB receptors in the antinociception induced by baclofen in the formalin test. Gen Pharmacol. 28(4):611–5
Larson CM, Wilcox GL, Fairbanks CA (2019) The study of pain in rats and mice. Comp Med. 69(6):555–70
Wilson PR, Yaksh TL (1978) Baclofen is antinociceptive in the spinal intrathecal space of animals. Eur J Pharmacol. 51(4):323–30
Minami T, Uda R, Horiguchi S, Ito S, Hyodo M, Hayaishi O (1992) Effects of clonidine and baclofen on prostaglandin F<sub>2</sub>α- induced Allodynia in conscious mice. PAIN RESEARCH. 7(2):129–34
Calcaterra NE, Barrow JC (2014) Classics in chemical neuroscience: diazepam (valium). ACS Chem Neurosci. 5(4):253–60
Treit D, Lolordo VM, Armstrong DE (1986) The effects of diazepam on “fear” reactions in rats are modulated by environmental constraints on the rat’s defensive repertoire. Pharmacol Biochem Behav. 25(3):561–5
Mandema JW, Gubbens-Stibbe JM, Danhof M (1991) Stability and pharmacokinetics of flumazenil in the rat. Psychopharmacology (Berl). 103(3):384–7
Izumi T, Inoue T, Tsuchiya K, Hashimoto S, Ohmori T, Koyama T (1999) Effects of the benzodiazepine antagonist flumazenil on conditioned fear stress in rats. Prog Neuropsychopharmacol Biol Psychiatry. 23(7):1247–58
Sieghart W, Chiou LC, Ernst M, Fabjan J, MS M, Lee MT (2022) alpha6-containing GABA(A) receptors: functional roles and therapeutic potentials. Pharmacol Rev 74(1):238–70
Ataka T, Gu JG (2006) Relationship between tonic inhibitory currents and phasic inhibitory activity in the spinal cord lamina II region of adult mice. Mol Pain. 2:36
Kogelman LJA, Christensen RE, Pedersen SH, Bertalan M, Hansen TF, Jansen-Olesen I et al (2017) Whole transcriptome expression of trigeminal ganglia compared to dorsal root ganglia in Rattus Norvegicus. Neuroscience. 350:169–79
Zhang C, Chen RX, Zhang Y, Wang J, Liu FY, Cai J et al (2017) Reduced GABAergic transmission in the ventrobasal thalamus contributes to thermal hyperalgesia in chronic inflammatory pain. Sci Rep. 7:41439
Bogaj K, Kaplon R, Urban-Ciecko J (2023) GABAAR-mediated tonic inhibition differentially modulates intrinsic excitability of VIP- and SST- expressing interneurons in layers 2/3 of the somatosensory cortex. Front Cell Neurosci. 17:1270219
Datta D, Arion D, Lewis DA (2015) Developmental expression patterns of GABAA receptor subunits in layer 3 and 5 pyramidal cells of monkey prefrontal cortex. Cereb Cortex. 25(8):2295–305
Ferando I, Mody I (2014) Interneuronal GABAA receptors inside and outside of synapses. Curr Opin Neurobiol. 26:57–63
Bovolin P, Santi MR, Puia G, Costa E, Grayson D (1992) Expression patterns of gamma-aminobutyric acid type A receptor subunit mRNAs in primary cultures of granule neurons and astrocytes from neonatal rat cerebella. Proc Natl Acad Sci U S A. 89(19):9344–8
Tornberg J, Segerstrale M, Kulesskaya N, Voikar V, Taira T, Airaksinen MS (2007) KCC2-deficient mice show reduced sensitivity to diazepam, but normal alcohol-induced motor impairment, gaboxadol-induced sedation, and neurosteroid-induced hypnosis. Neuropsychopharmacology. 32(4):911–8
Chandra D, Jia F, Liang J, Peng Z, Suryanarayanan A, Werner DF et al (2006) GABAA receptor alpha 4 subunits mediate extrasynaptic inhibition in thalamus and dentate gyrus and the action of gaboxadol. Proc Natl Acad Sci U S A. 103(41):15230–5
Ebert B, Wafford KA, Deacon S (2006) Treating insomnia: Current and investigational pharmacological approaches. Pharmacol Ther. 112(3):612–29
Smith M, Lindquist CE, Birnir B (2003) Evidence for inhibitory effect of the agonist gaboxadol at human alpha 1 beta 2 gamma 2S GABAA receptors. Eur J Pharmacol. 478(1):21–6
Harriott AM, Chung DY, Uner A, Bozdayi RO, Morais A, Takizawa T et al (2021) Optogenetic Spreading depression elicits trigeminal pain and anxiety behavior. Ann Neurol. 89(1):99–110
Acknowledgments
We are grateful to Prof. Dr. Ege Kavalali for providing us with levcromakalim, tiagabine, and gaboxadol. We express our gratitude to the Global Migraine and Pain Society for granting us permission to use Biorender in preparing Fig 7.
Funding
This study was supported by the Hacettepe University Scientific Research Project Coordination Unit, Türkiye (Grant No: THD-2022–20171) and the Turkish Academy of Sciences (TÜBA).
Author information
Authors and Affiliations
Contributions
BA, FO, HB, and YS designed the study. BA, BC, and EA collected and analyzed the data. BA, BC, EA, FO, HB, and YS drafted the manuscript. All authors reviewed and approved the final manuscript.
Corresponding authors
Ethics declarations
Ethics approval and consent to participate
The study was approved by the review board of Hacettepe University. Animal suffering was minimized in accordance with ARRIVE guidelines.
Consent for publication
Not applicable.
Competing interests
The authors declare no competing interests.
Additional information
Publisher’s Note
Springer Nature remains neutral with regard to jurisdictional claims in published maps and institutional affiliations.
Rights and permissions
Open Access This article is licensed under a Creative Commons Attribution 4.0 International License, which permits use, sharing, adaptation, distribution and reproduction in any medium or format, as long as you give appropriate credit to the original author(s) and the source, provide a link to the Creative Commons licence, and indicate if changes were made. The images or other third party material in this article are included in the article's Creative Commons licence, unless indicated otherwise in a credit line to the material. If material is not included in the article's Creative Commons licence and your intended use is not permitted by statutory regulation or exceeds the permitted use, you will need to obtain permission directly from the copyright holder. To view a copy of this licence, visit http://creativecommons.org/licenses/by/4.0/. The Creative Commons Public Domain Dedication waiver (http://creativecommons.org/publicdomain/zero/1.0/) applies to the data made available in this article, unless otherwise stated in a credit line to the data.
About this article
Cite this article
Alpay, B., Cimen, B., Akaydin, E. et al. Extrasynaptic δGABAA receptors mediate resistance to migraine-like phenotype in rats. J Headache Pain 25, 75 (2024). https://doi.org/10.1186/s10194-024-01777-4
Received:
Accepted:
Published:
DOI: https://doi.org/10.1186/s10194-024-01777-4