Abstract
Purpose of Review
Research has evaluated the potential impact of folate on cancer risk with conflicting findings. Studies have demonstrated increased risk, no effect, and decreased risk. This review summarizes findings of mixed results between folate intake, serum levels, gene polymorphisms, and cancer risk based on meta-analyses from the past five years.
Recent Finding
Low or deficient folate status is associated with increased risk of many cancers. Folic acid supplementation and higher serum levels are associated with increased risk of prostate cancer. Gene polymorphisms may impact risk in certain ethnic groups.
Summary
Folate has been studied extensively due to its role in methylation and nucleotide synthesis. Further prospective studies are needed to clarify optimal levels for nutrient remediation and risk reduction in those at risk, as well as elucidate the association between high intake, high serum levels, and prostate cancer risk. Future considerations for cancer risk may include gene interactions with nutrients and environmental factors.
Similar content being viewed by others
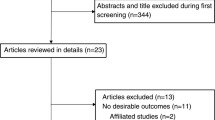
Avoid common mistakes on your manuscript.
Introduction
Folate is an essential water-soluble B vitamin found in foods, including dark-green leafy vegetables and legumes. Folic acid is the synthetic form of the vitamin present in supplements and fortified foods, namely grains and cereals. Dietary folate exists in a reduced state with polyglutamate side chains requiring oxidation and hydrolysis for absorption, whereas folic acid exists as the oxidized pteroylmonoglutamate form making it readily bioavailable [1]. Dietary folate bioavailability ranges from 10 to 98% and is influenced by intestinal pH, enzymatic activity, presence of alcohol and other inhibitors, malabsorption disorders, and the food matrix [1]. To account for the differences in absorption between folate and folic acid, folate equivalents are used. The recommended dietary allowance for adults in the USA is set at 400 micrograms (mcg) of dietary folate equivalents (DFE). One microgram of DFE is equivalent to 1 mcg dietary folate, 0.6 mcg folic acid consumed with food, or 0.5 mcg supplemental folic acid on an empty stomach. The upper limit for folate is 1,000 mcg/day, which is one fifth of the minimum amount known to mask a B12 deficiency [2, 3]. About 5% of the US population gets more than the upper limit, usually due to dietary supplements [4]. There are several ways to assess folate status. Serum levels, are thought to reflect recent intake. Deficiency is categorized as less than 7 nmol/L to less than 10 nmol/L. Red blood cell (RBC) folate represents folate status over months with levels less than 315 to 363 nmol/L suggesting deficiency [1, 3, 5]. Elevated urinary formiminoglutamate (FIGLU) excretion and deoxyuridine suppression tests are additional methods for assessing folate status. Also, elevated homocysteine may be a functional measure of folate status [1].
Due to its role in one-carbon metabolism, folate has been studied extensively as a possible mechanism for cancer development. Folate as 5-Methyltetrahydrofolate (5-MTHF) and cobalamin are required for the conversion of homocysteine to methionine in the methionine pathway. Methionine is converted to S-adenosylmethionine (SAM). SAM is a chief methyl contributor to many reactions in the body, including DNA and RNA methylation [6]. Inadequate production of SAM may lead to decreased methylation of CpG islands in DNA affecting gene transcription, altering expression of tumor suppressor genes and proto-oncogenes [6, 7]. Furthermore, folate deficiency can impair conversion of deoxyuridine monophosphate (dUMP) to deoxythymidine monophosphate (dTMP), the nucleic acid necessary for DNA synthesis and repair [6, 7]. The misincorporation of uracil for thymidine can eventually lead to unstable DNA, DNA strand breaks, and faulty DNA repair [6, 7].
Excess folate intake also poses a concern. Mandatory fortification of wheat flour and enriched cereal grain products aimed at reducing the incidence of neural tube defects has raised questions regarding growth of nascent cancers [3]. Mandatory fortification has been in effect in the USA since 1998, and at least 87 countries have regulations in different stages of implementation [8]. In the USA, a 140 mcg/100 g product is added to fortified foods and in some countries more is added; for example, Chile adds 220 mcg/100 g product [3]. In addition, 35% of US adults may take a supplement containing 400 mcg folic acid, and in some countries, the frequency of people supplementing is even higher. Average total folate intake of 813 mcg/day for men and 724 mcg/day for women has been reported in the USA, with only 15–17% of adults not meeting the daily recommended amount [4].
Folic acid, found in fortified foods and supplements, is converted to tetrahydrofolate in the liver by dihydrofolate reductase (DHFR). It has been reported that folic acid in excess of 400 mcg may saturate the DHFR enzyme, resulting in unreduced folic acid, which has been hypothesized as a potential mechanism for carcinogenesis [3, 9•].
Just as overt folate deficiency and excess intake may interfere with cell replication and survival, reduced enzyme efficiency can also interfere with nutrient metabolism and influence disease risk. Methylenetetrahydrofolate reductase (MTHFR) is a key flavoenzyme that catalyzes the reduction of 5,10-methylenetetrahydrofolate to 5-methyltetrahydrofolate (5-MTHF). It is encoded by the gene MTHFR. There are two well-described MTHFR gene polymorphisms: C677T and A1298C. The C677T variant may occur in close to 20–40% of the population [12, 53]. Both variants are associated with reduced enzyme activity [11]. Enzyme efficiency is reduced by up to 45% for the 677CT variant and by up to 70% for 677TT [10]. The variated form of the enzyme has less affinity to its cofactor, flavin adenine dinucleotide. Cytosolic serine hydroxy methyl transferase (SHMT1) is another key enzyme in folate metabolism. It converts serine and tetrahydrofolate to glycine and 5,10-methylenetetrahydrofolate, respectively. 5,10-methylenetetrahydrofolate is a substrate for purine and pyrimidine synthesis. The combination of folate deficiency and polymorphisms may result in DNA hypo- [12] or hypermethylation [13].
The relationship between folate and cancer risk remains uncertain, as studies have demonstrated positive, negative, and neutral associations. Also of note, antifolates are used in cancer treatment, however that is outside of the scope of this review. Therefore, the purpose of this paper is to summarize current knowledge of folate’s impact on cancer risk and identify opportunities for future work in this area based on a review of meta-analyses from the past 5 years. Many factors contribute to the findings, including the type and dose of the vitamin (dietary, supplemental, and total), serum levels and method of evaluating, cancer type and presence of gene polymorphisms.
Methods
This review was conducted to evaluate the impact of folate on cancer risk. PubMed/MEDLINE and the Cochrane database were searched by combining the key words “folate” or “folic acid” and “cancer risk.” Meta-analyses published in the last 5 years in human adults and in English were included. Titles and abstracts were reviewed to select articles related to cancer risk in adults. Articles were excluded if they were related to cancer treatment, childhood cancer risk, or other disease states. Additional articles were obtained from references of relevant papers. A summary of the review articles selected is shown in Tables 1 and 2.
Supplementation
Several meta-analyses have evaluated the impact of folic acid (FA) supplementation on cancer risk using data from primary prevention trials for a number of conditions reporting cancer incidence. Two meta-analyses of Randomized Controlled Trials (RCTs) and a meta-analysis with 12 RCTs and 7 observational studies, evaluated the impact of FA supplementation, with or without other B vitamins, on cancer risk. Doses of FA ranged between 0.4 and 40 mg/day. Only one study used 5-methyltetrahydrofolate (5-MTHF), the metabolically active form of folate at a dose of 560 mcg/day. However, when that study was removed it did not change results. No significant effect of FA supplementation was found on total cancer risk in two of the analyses [32•, 33•]. One analysis reported a “borderline significant” 7% increase in overall cancer risk; however, the confidence interval (CI) included 1 [34•]. Two analyses also found no significant association between FA supplementation and risk of colorectal, prostate, lung, breast, or hematological cancers [32•, 33•]; however, one found a 24% increased risk of prostate cancer [34•]. One found no effect with less common types of cancer and cancers of unknown origin [33•]; and another found a 53% lower risk of melanoma [32•].
In subgroup analysis, Qin found a 10% increased risk of cancer in studies where > 60% of participants were on lipid-lowering drugs and hypothesized that statin treatment interacting with homocysteine metabolism in the presence of FA supplementation may impact cancer risk [32•]. Wien reported a 21% increased risk of cancer with doses between 0.4 and 1 mg/day, but not with doses higher than 1 mg/day and a 19% increased risk in smokers [34•].
Additional analyses evaluating risk in specific cancer types found a significant 3% decrease in risk of renal cancer with 100-mcg FA supplementation in a dose-response analysis [31]. When comparing highest with lowest intake from food fortification and supplements, two studies reported an inverse association and one study found no effect on risk of colorectal cancer [35,36,37].
Serum Levels
Zhang and colleagues found elevated homocysteine levels and folate deficiency, as determined by serum folate level, to be associated with increased overall risk of cancer in a meta-analysis of 83 case-control studies [11]. Folate level was inversely associated with most cancer types except prostate, bladder, pancreatic, and breast. Protection was seen in studies from Europe, Asia, the Middle East, and Latin America, but not the USA or Australia. Zhao and colleagues found that the highest compared to lowest serum folate level was associated with decreased risk of esophageal cancer [20].
Higher or at least adequate levels may also be protective for cervical cancer. Increased risk of cervical cancer was observed with deficiency per serum folate in Asian, but not American studies. Serum levels used to categorize deficiency were different between studies, and some were higher than what is reported in the literature, which may have impacted results. These findings need to be confirmed using consistent cutoffs categorizing deficiency [28].
Two analyses found increased risk of prostate cancer when comparing highest with lowest serum folate levels. Both included five of the same nested case-control studies [17, 24]. One group included one additional case-control study and one retrospective case-control study [17]. Wang’s dose-response analysis reported a 4% increase in risk with each 5 nmol/L increase in serum folate [24]. After removing a retrospective study that was contributing to significant heterogeneity, Tio’s group found the association was still significant. The study removed was conducted in Jamaica, where risk was much higher with high levels, and the authors posed the question of whether or not folate may play a role in racial differences seen in prostate cancer risk [17, 24].
The studies in these analyses either compared tertile 3 to 1 or compared low level with high level. Low levels were between less than 4.82 nmol/L and less than 10.9 nmol/L and high between less than 10.3 and 58.2 nmol/L. Only one study found an association, but pooled results were consistent with that study. Serum folate was non-fasting, which could impact results and reflect recent intake. Some studies used a level barely considered adequate as the “high” category making it difficult to interpret these findings. The authors mentioned that there was a low incidence of cancers and could have impacted results [24]. Future studies should use serial measurements of RBC folate with clear cutoffs to help clarify this association [17].
Additional analyses found no association when comparing highest with lowest serum levels and risk of renal [31], pancreatic [22], breast [14], or colon cancers [29]. Chuang and colleagues found an inverse association for studies using radioimmunoassay, surfacing issues related to methods, including testing measurements, storage of samples and vitamin degradation, and variations in cohorts and study design [29].
Cancer Types, Intake, and Risk
Folate intake is associated with a protective effect for some cancers, little to no effect for others, and a potential for increased risk with higher intake. When comparing the highest to lowest intake of folate, higher intake was associated with a nearly 50% decreased risk for squamous cell carcinoma of the head and neck (HNSCC) [18]; 35% reduced risk for oral cavity and pharyngeal (OPC) [19]; 41% reduced risk for all histological types of esophageal [21]; 34% reduction in pancreatic [21, 22]; and 16% reduction in bladder cancers [25]. The prospective analysis for pancreatic cancer was only significant when a study was removed that had lower levels of folate intake then the other studies. That study had a mean high intake of 246 mcg/day, an amount below the recommended intake, compared to 350–700 mcg/day in other studies [21]. Heavy alcohol use with low folate status was associated with 4 times the risk of OPC compared with low alcohol and high folate intake [19].
Dose-response analyses have shown that every 100 mcg/day increase in folate intake was associated with a 4.3% decrease in risk of HNSCC [18]; 12% decrease in risk of esophageal cancer in studies other than Asian and American studies [20]; and a 7% lower risk of pancreatic cancer [22].
There was no reduction in risk seen with lung cancer until one study with all females was removed due to heterogeneity. Then, a 10% reduction in risk was observed [23]. The authors attributed this to a higher percentage of smokers in the males [23]. Similarly, no protective effect comparing highest with lowest quantiles of folate intake for risk of ovarian cancer was found until a study was removed due to heterogeneity. Then, a 27% reduced risk was seen with higher intake [26]. It should be noted that highest intake in the removed study had a cutoff that could have included intake below or at the Dietary Reference Intake (DRI) [26].
Meta-analyses of studies for dietary folate and total folate intake found no association with risk of prostate cancer. Low intake in studies ranged from 123.8 mcg/day to 411 mcg/day (diet) and 551.9 mcg/day (total) and high intake reported as 235 mcg/day to 669 mcg/day (diet) and 934.1 mcg/day (total). In many studies, low and high diet intake are both below the recommended daily amount or the cutoffs for high with low overlap, making it difficult to discern a true cut point for risk if one does exist [17, 24].
Recent studies regarding folate intake have found little to no association between folate intake and breast cancer, with the exception of those comparing higher alcohol consumption with lower folate intake [14,15,16, 52]. Chen et al. and Zhang et al. demonstrated a non-linear dose-effect relationship between dietary folate intake level and breast cancer risk [14, 15]. For prospective studies, Chen et al. showed a U-shaped dose-effect relationship for dietary folate intake level and breast cancer risk [14]. There was a significantly decreased breast cancer risk with dietary folate intake between 153 and 400 mcg compared with those of < 153 mcg, but there was not a statistically significant reduced risk with dietary folate intake > 400 mcg compared to < 153 mcg [14]. For case-control studies, Chen et al. found that an increment of 100 mcg dietary folate per day showed a 9% reduction in breast cancer risk [14]. Zhang et al. found a potential J-shape correlation between folate intake and breast cancer risk; daily folate intake of 200–320 mcg was associated with lower breast cancer risk, but the risk increased significantly with a daily folate intake > 400 mcg [15]. Liu et al. found that a 220 mcg/day increment in dietary folate intake was not associated with breast cancer risk [16]. Studies varied in the incremental dose used to assess a dose-effect relationship.
Du and colleagues found a “marginal negative association” between total folate intake and risk of endometrial cancer [27]. An 11% reduction in risk was observed between higher intake and risk; however, the finding was not statistically significant. Interestingly, the association was not found for retrospective studies conducted outside of N. America (11 out of 14 studies). In the highest category of folate intake, which ranged from 205.8 to 987.7 mcg between studies, they reported a 5% elevated risk for every 100 mcg/day increase in folate intake, suggesting a threshold effect. They also suggested that type II or non-estrogen-dependent cancers are more likely to be related to p53 mutations, DNA damage, and changes to cell proliferation, yet the type of endometrial cancer not distinguished in most studies [27].
Gene Polymorphisms
Gene variants associated with the folate metabolic pathway disturb nutrient bioavailability and contribute to a range of diseases. Methylenetetrahydrofolate reductase (MTHFR) directly affects DNA synthesis and methylation and has been associated with increased risk of certain cancers. In a meta-analysis of 134 case-control studies, the MTHFR C677T polymorphism was significantly associated with increased tumor risk [38]. In stratified analysis of the same study, increased risks of stomach and esophageal cancer were observed and increased risk in Asian ethnicity was observed [38]. Mutation of the 677 gene has also been associated with increased risk of lung, hepatocellular, breast, brain, and ovarian cancer in Asian populations and breast cancer in Turkish population [7, 12, 26, 39, 42, 44]. While no association was observed for MTHFR and oral cancer, subgroup analysis revealed a statistically significant increased risk in heavy versus non-heavy drinkers [46]. Two meta-analyses showed no association between MTHFR C677T allele and glioma, while an increased risk of brain tumor and meningioma was associated with the heterozygous genotype [41, 42]. MTHFR TT has been shown to be positively associated with risk of thyroid cancer [48] and increased risk of non-Hodgkin lymphoma in Caucasians [49].
Conversely, MTHFR 677TT may have a protective effect against colorectal cancer, in aggressive forms of prostate cancer, and prostate and non-Hodgkin lymphoma among Asians [30, 45, 49]. Similarly, a decreased risk of oral cancer has been associated with the MTHFR 677CT genotype in Asians [46]. High total and dietary folate intake was inversely associated with colorectal cancer for the wild-type allele, whereas MTHFRTT showed a protective effect for colorectal with high total folate intake compared to low total folate intake [30].
The presence of the MTHFR A1298C variant has been significantly associated with increased risk for cervical cancer, globally and among Asians [40]. Homozygosity for A1298C in Asians has been associated with an increased risk of myeloid leukemia and non-Hodgkin lymphoma [47, 49]. Conversely, a decreased risk of liver cancer has been observed with the homozygous variant MTHFR 1298 CC overall and in Asian populations [43, 44].
Homozygosity for SHMT C1420T has been associated with a protective effect against colorectal cancer and overall cancer risk for Asian ethnicity [50]. Another study found no association between colorectal cancer and SHMT; however, subgroup analysis showed a significant decreased cancer risk with low folate intake in the presence of SHMT1 variation. Folate intake level was not defined. A positive association was found between SHMT1 and risk for non-Hodgkin lymphoma [51].
Conclusion
A number of factors need to be considered when evaluating and comparing studies, including limitations in the current literature. There is a great deal of heterogeneity in the populations studied, including geographical and ethnic differences, presence of polymorphisms, use of data from both pre- and post-fortification time periods, and inclusion of populations with and without fortification. Also, many studies relating to folate intake are observational and use a one-time food frequency questionnaire, which does not consider change in diet over time, and may not account for other lifestyle factors, including alcohol intake. Different methods and cutoffs are used to categorize highest compared to lowest intake and there is variability in serum levels, with a single serum level used to evaluate risk. Cutoffs overlap making it difficult to discern a true cut point if risk of one exists. The length of time for follow up varies between studies and may not be adequate to evaluate impact on risk. This makes it difficult to form a single conclusion regarding the impact of folate on cancer risk. However, there are populations who appear to be at risk due to either inadequate or excess intake.
Inadequate folate intake or deficiency, as measured by serum levels, may increase risk for cancer, including cancers of the head and neck [18], oral cavity and pharynx [19], esophagus [21], pancreatic [21, 22], bladder [25], and cervix. Low intake combined with high alcohol intake may also increase the risk of breast cancer. Gene polymorphisms may add to risk in certain ethnic groups. Clinicians should aim to optimize dietary folate intake and/or consider supplementing intake in individuals at risk for or with known deficiency, for example those with alcohol dependence or malabsorption. Prospective studies are needed to identify what level of folate intake is needed to correct deficiency based on gene status and evaluate whether or not nutrient remediation in high-risk populations can mitigate cancer risk.
In addition, more research is needed to help understand if the protective effects of folate are weaker in populations with a relatively higher intake level from mandatory fortification and/or supplementation. Population concerns regarding fortification and increased cancer risk are challenged in the current research. Fortification status in studies did not have any impact on risk with supplementation [32•, 33•]. Fortification practices vary and increasing use of organic and gluten-free flours, which may not be fortified, may change population intake over time. More prospective, post-fortification work needs to be done in some populations. For example, the association between supplemental intake, elevated serum levels, and increased risk of prostate cancer should be explored in the context of gene variants. Clinicians should weigh the pros and cons of supplementation in the presence of adequate intake with individuals based on all risk factors for disease.
Finally, it is important to note that dozens of enzymes affect the efficiency of folate-related metabolic pathways, and polymorphisms in these enzymes may alter substrate availability of folate and/or other nutrients as well as gene expression. Future research efforts should be aimed at understanding how polymorphisms affect nutrient status in ethnic-specific populations, how nutrient remediation affects enzyme function in the presence of polymorphisms, and how these may influence risk [53]. Also, it is important to remember that additional nutrients are involved in one-carbon metabolism. By studying folate intake or serum levels in isolation, we may be missing the critical role of synergy among nutrients. For the clinician, it is important to evaluate each case individually and consider overall diet pattern in the context of all internal and external aspects that impact disease risk.
References
Papers of particular interest, published recently, have been highlighted as: • Of importance
Gropper SS, Smith JL. Advanced nutrition and human metabolism, 6th Edition [Internet]. Wadsworth Belmont, CA; 2013. p. 344–353. Available from: https://www.cengage.co.uk/books/9781133104056/.
Health NI of Folate. Dietary Supplement Fact Sheet. [Internet]. National Institutes of Health. 2012 [cited 2018 Jun 6]. p. 1–10. Available from: https://ods.od.nih.gov/factsheets/Folate-HealthProfessional/#en2
Crider KS, Bailey LB, Berry RJ. Folic acid food fortification-its history, effect, concerns, and future directions. Nutrients. 2011;3(3):370–84.
Bailey RL, Dodd KW, Gahche JJ, Dwyer JT, Mcdowell MA, Yetley EA, et al. Total folate and folic acid intake from foods and dietary supplements in the United States: 2003–2006. Am J Clin Nutr. 2010:2003–6.
Benoist D. Conclusions of a WHO Technical Consultation on folate and vitamin B 12 deficiencies. Food Nutr Bull. 2008;29(2):238–44.
Liew SC. Folic acid and diseases – supplement it or not? Rev Assoc Med Bras. 2016;62(1):90–100.
Rai V. Folate pathway gene MTHFR C677T polymorphism and risk of lung cancer in Asian populations. Asian Pacific J Cancer Prev. 2014;15(21):9259–64.
Food Fortification Initiative. Global Progress of Industrially Milled Cereal Grains [Internet]. 2018 [cited 2018 Jun 6]. Available from: http://www.ffinetwork.org/global_progress/index.php
• Gruber B. B-group vitamins: chemoprevention? Adv Clin Exp Med [Internet]. 2016;25(3):561–8. Available from: http://www.advances.umed.wroc.pl/en/article/2016/25/3/561/. Review on the role of B vitamins in chemoprevention.
Varela-Rey M, Woodhoo A, Martinez-Chantar ML, Mato JM, Lu SC. Alcohol, DNA methylation, and cancer. Alcohol Res: Curr Rev. 2013;35(1):25–35.
Zhang D, Wen X, Wu W, Guo Y, Cui W. Elevated homocysteine level and folate deficiency associated with increased overall risk of carcinogenesis: meta-analysis of 83 case-control studies involving 35,758 individuals. PLoS One. 2015;10(5):1–16.
Rai V. The methylenetetrahydrofolate reductase C677T polymorphism and breast cancer risk in asian populations. Asian Pacific J Cancer Prev. 2014;15(14):5853–60.
Bistulfi G, Vandette E, Matsui S-I, Smiraglia DJ. Mild folate deficiency induces genetic and epigenetic instability and phenotype changes in prostate cancer cells. BMC Biol. 2010;8:6.
Chen P, Li C, Li X, Li J, Chu R, Wang H. Higher dietary folate intake reduces the breast cancer risk: a systematic review and meta-analysis. Br J Cancer. 2014;110(9):2327–38.
Zhang Y-F, Shi W-W, Gao H-F, Zhou L, Hou A-J, Zhou Y-H. Folate intake and the risk of breast cancer: a dose-response meta-analysis of prospective studies. PLoS One [Internet]. 2014;9(6):e100044. https://doi.org/10.1371/journal.pone.0100044.
Liu M, Cui L-H, Ma A-G, Li N, Piao J-M. Lack of effects of dietary folate intake on risk of breast cancer: an updated meta-analysis of prospective studies. Asian Pac J Cancer Prev [Internet]. 2014;15(5):2323–8. Available from: http://www.ncbi.nlm.nih.gov/pubmed/24716978
Tio M, Andrici J, Cox MR, Eslick GD. Folate intake and the risk of prostate cancer: a systematic review and meta-analysis. Prostate Cancer Prostatic Dis [Internet]. 2014;17(3):213–9. https://doi.org/10.1038/pcan.2014.16.
Fan C, Yu S, Zhang S, Ding X, Su J, Cheng Z. Association between folate intake and risk of head and neck squamous cell carcinoma. Medicine (Baltimore) [Internet]. 2017;96(42):e8182. Available from: http://insights.ovid.com/crossref?an=00005792-201710200-00039
Galeone C, Edefonti V, Parpinel M, Leoncini E, Talamini R, Olshan AF, et al. Folate intake and the risk of oral cavity and pharyngeal cancer: a pooled analysis within the INHANCE Consortium Carlotta. 2016;136(4):904–14.
Zhao Y, Guo C, Hu H, Zheng L, Ma J, Jiang L, et al. Folate intake, serum folate levels and esophageal cancer risk: an overall and dose-response meta-analysis. Oncotarget [Internet]. 2017;8(6):10458–69. Available from: http://www.oncotarget.com/abstract/14432
Tio M, Andrici J, Cox MR, Eslick GD. Folate intake and the risk of upper gastrointestinal cancers: a systematic review and meta-analysis. J Gastroenterol Hepatol [Internet]. 2014;29(2):250–8. https://doi.org/10.1111/jgh.12446.
Lin HL, An QZ, Wang QZ, Liu CX. Folate intake and pancreatic cancer risk: an overall and dose-response meta-analysis. Public Health. 2013;127(7):607–13.
Zhang Y-F, Zhou L, Zhang H-W, Hou A-J, Gao H-F, Zhou Y-H. Association between folate intake and the risk of lung cancer: a dose-response meta-analysis of prospective studies. PLoS One [Internet]. 2014;9(4):e93465. https://doi.org/10.1371/journal.pone.0093465.
Wang R, Zheng Y, Huang J-Y, Zhang A-Q, Zhou Y-H, Wang J-N. Folate intake, serum folate levels, and prostate cancer risk: a meta-analysis of prospective studies. BMC Public Health [Internet]. 2014;14(1):1326. https://doi.org/10.1186/1471-2458-14-1326.
He H, Shui B. Folate intake and risk of bladder cancer: a meta-analysis of epidemiological studies. Int J Food Sci Nutr. 2014;65(3):286–92.
Li C, Chen P, Hu P, Li M, Li X, Guo H, et al. Folate intake and MTHFR polymorphism C677T is not associated with ovarian cancer risk: evidence from the meta-analysis. Mol Biol Rep. 2013;40(12):6547–60.
Du L, Wang Y, Zhang H, Zhang H, Gao Y. Folate intake and the risk of endometrial cancer : A meta- analysis. 2016;7(51):85176–84.
Zhou X, Meng Y. Association between serum folate level and cervical cancer: a meta-analysis. Arch Gynecol Obstet. 2016;293(4):871–7.
Chuang SC, Rota M, Gunter MJ, Zeleniuch-Jacquotte A, Eussen SJPM, Vollset SE, et al. Quantifying the dose-response relationship between circulating folate concentrations and colorectal cancer in cohort studies: a meta-analysis based on a flexible meta-regression model. Am J Epidemiol. 2013;178(7):1028–37.
Kennedy DA, Stern SJ, Matok I, Moretti ME, Sarkar M, Adams-Webber T, et al. Folate intake, MTHFR polymorphisms, and the risk of colorectal cancer: a systematic review and meta-analysis. J Cancer Epidemiol. 2012;2012:14–8.
Mao B, Li Y, Zhang Z, Chen C, Chen Y, Ding C, et al. One-carbon metabolic factors and risk of renal cell cancer: a meta-analysis. PLoS One. 2015;10(10):1–10.
Qin X, Cui Y, Shen L, Sun N, Zhang Y, Li J, et al. Folic acid supplementation and cancer risk: a meta-analysis of randomized controlled trials. Int J Cancer. 2013;133(5):1033–41. Meta-analysis of RCTs reporting the impact of folic acid supplementation on cancer risk using data from primary prevention trials for a number of conditions reporting cancer incidence.
• Vollset SE, Clarke R, Lewington S, Ebbing M, Halsey J, Lonn E, et al. Effects of folic acid on overall and site-specific cancer incidence during the randomised trials: meta-anlayses of the data on 50 000 individuals. Lancet. 2013;381(9871). Meta-analysis of RCTs reporting the impact of folic acid supplementation on cancer risk using data from primary prevention trials for a number of conditions reporting cancer incidence.
• Wien TN, Pike E, Wisløff T, Staff A, Smeland S, Klemp M. Cancer risk with folic acid supplements: a systematic review and meta-analysis. BMJ Open. 2012;2(1):1–13. Meta-analysis of RCTs reporting the impact of folic acid supplementation on cancer risk using data from primary prevention trials for a number of conditions reporting cancer incidence.
Qin T, Du M, Du H, Shu Y, Wang M, Zhu L. Folic acid supplements and colorectal cancer risk: Meta-analysis of randomized controlled trials. Sci Rep [Internet]. 2015. https://doi.org/10.1038/srep12044
Heine-Bröring RC, Winkels RM, Renkema JMS, Kragt L, Van Orten-Luiten ACB, Tigchelaar EF, et al. Dietary supplement use and colorectal cancer risk: a systematic review and meta-analyses of prospective cohort studies. Int J Cancer. 2015;136(10):2388–401.
Liu Y, Yu Q, Zhu Z, Zhang J, Chen M, Tang P, et al. Vitamin and multiple-vitamin supplement intake and incidence of colorectal cancer: a meta-analysis of cohort studies. Med Oncol. 2015;32(1):1–10.
Tang M, Wang S-Q, Liu B-J, Cao Q, Li B-J, Li P-C, et al. The methylenetetrahydrofolate reductase (MTHFR) C677T polymorphism and tumor risk: evidence from 134 case–control studies. Mol Biol Rep [Internet]. 2014;41(7):4659–73. https://doi.org/10.1007/s11033-014-3337-9.
Kaya EF, Karakus N, Ulusoy AN, Özaslan C, Kara N. Association of the MTHFR gene C677T polymorphism with breast cancer in a Turkish population. Oncol Res Treat [Internet]. 2016;39(9):534–8. Available from: https://www.karger.com/Article/FullText/448084
Yi K, Yang L, Lan Z, Xi M. The association between MTHFR polymorphisms and cervical cancer risk: a system review and meta analysis. Arch Gynecol Obstet [Internet]. 2016;294(3):579–88. https://doi.org/10.1007/s00404-016-4037-6.
Lu Q, Dai D, Zhao W, Wang L, Yue Z, Chen X, et al. Association between MTHFR 677C>T polymorphism and risk of gliomas: evidence from a meta-analysis. Tumor Biol. 2013;34(5):2801–7.
Xu C, Yuan L, Tian H, Cao H, Chen S. Association of the MTHFR C677T polymorphism with primary brain tumor risk. Tumor Biol. 2013;34(6):3457–64.
Liang TJ, Liu H, Zhao XQ, Tan YR, Jing K, Qin CY. Quantitative assessment of the association between MTHFR rs1801131 polymorphism and risk of liver cancer. Tumor Biol. 2014;35(1):339–43.
Qi X, Sun X, Xu J, Wang Z, Zhang J, Peng Z. Associations between methylenetetrahydrofolate reductase polymorphisms and hepatocellular carcinoma risk in Chinese population. Tumor Biol. 2014;35(3):1757–62.
Guo S, Jiang X, Chen X, Chen L, Li X, Jia Y. The protective effect of methylenetetrahydrofolate reductase C677T polymorphism against prostate cancer risk: evidence from 23 case-control studies. Gene [Internet]. 2015;565(1):90–5. https://doi.org/10.1016/j.gene.2015.03.067.
Jia J, Ma Z, Wu S. Positive association between MTHFR C677T polymorphism and oral cancer risk: a meta-analysis. Tumor Biol. 2014;35(5):4943–8.
Dong S, Liu Y, Chen J. MTHFR gene polymorphism and risk of myeloid leukemia: a meta-analysis. Tumor Biol. 2014;35(9):8913–9.
Yang Y-M, Zhang T-T, Yuan L, Ren Y. The association between the C677T polymorphism in MTHFR gene and the risk of thyroid cancer: a meta-analysis. Eur Rev Med Pharmacol Sci. 2014;18(15):2097–101.
He J, Liao XY, Zhu JH, Xue WQ, Shen GP, Huang SY, et al. Association of MTHFR C677T and A1298C polymorphisms with non-Hodgkin lymphoma susceptibility: evidence from a meta-analysis. Sci Rep. 2014;4:1–9.
Wang Q, Lu K, Du H, Zhang Q, Chen T, Shu Y, et al. Association between cytosolic serine hydroxymethyltransferase (SHMT1) gene polymorphism and cancer risk: a meta-analysis. Biomed Pharmacother [Internet]. 2014;68(6):757–62. https://doi.org/10.1016/j.biopha.2014.08.002.
Wang Y, Zhang S, Xue W, Zhu M, Zheng L. SHMT1 C1420T polymorphism contributes to the risk of non-Hodgkin lymphoma: evidence from 7309 patients. Chin J Cancer. 2015;34.
Tio M, Andrici J, Eslick GD. Folate intake and the risk of breast cancer: a systematic review and meta-analysis. Breast Cancer Res Treat. 2014;145:513–24.
Marini NJ, Gin J, Ziegle J, Keho KH, Ginzinger D, Gilbert DA, et al. The prevalence of folate-remedial MTHFR enzyme variants in humans. Proc Natl Acad Sci U S A. 2008 Jun;105(23):8055–60.
Author information
Authors and Affiliations
Corresponding author
Ethics declarations
Conflict of Interest
Renee Pieroth, Stephanie Paver, Sharon Day, and Carolyn Lammersfeld declare they have no conflict of interest.
Human and Animal Rights and Informed Consent
This article does not contain any studies with human or animal subjects performed by any of the authors.
Additional information
This article is part of the Topical Collection on Cancer
Rights and permissions
Open Access This article is distributed under the terms of the Creative Commons Attribution 4.0 International License (http://creativecommons.org/licenses/by/4.0/), which permits unrestricted use, distribution, and reproduction in any medium, provided you give appropriate credit to the original author(s) and the source, provide a link to the Creative Commons license, and indicate if changes were made.
About this article
Cite this article
Pieroth, R., Paver, S., Day, S. et al. Folate and Its Impact on Cancer Risk. Curr Nutr Rep 7, 70–84 (2018). https://doi.org/10.1007/s13668-018-0237-y
Published:
Issue Date:
DOI: https://doi.org/10.1007/s13668-018-0237-y