Abstract
Samples of road dust (RD) from petrol stations (n = 20) located in Rzeszów (Podkarpackie, Poland) were analysed to find potential markers of traffic-related pollution. The level (μg/g) of: Mo, U, As, Hg, Th, Pb, Ni, Cu, Cr, Be, Sr, Zr, Zn, Mn and Fe were measured using field portable X-ray spectroscopy (FP-XRF) as a direct, rapid and ‘white analytical technique’. Our research demonstrated for the first time the utility of using FP-XRF for environmental toxicological assessment of RD from petrol stations. The qualitative and quantitative composition of the dust samples tested provides valuable research material for creating and updating regulations and finding pollution hotspots such as petrol stations. It was possible to find potential markers of traffic-related pollution (e.g., Zr and Fe forms of disk brakes or brake pads). Certain elements of the study give ambiguous signals related to fuel distribution and road traffic. They can come from various sources of environmental contamination. The adoption of evidence-based policies to minimise pollution, conserve the environment, and protect the well-being of people living or working near gas stations is anticipated to be aided by these findings for policymakers, regulatory authorities, and stakeholders. Incorporating toxicological risk assessment framework, this study pioneers the investigation of human exposure to hazardous elements in RD, an in-depth evaluation of both carcinogenic and non-carcinogenic health risks associated with such exposure including lifetime average daily dose. Our findings contribute novel insights into the environmental toxicology of urban areas, emphasising the need for tailored interventions to mitigate these risks.
Similar content being viewed by others
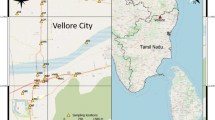
Avoid common mistakes on your manuscript.
Introduction
The identification of sources of toxic elements is very important for the prevention of pollution and the protection of human health and environmental studies. Road dust (RD) has become a major source of environmental pollution, particularly in large cities. Reports on the composition of RD in certain locations have highlighted the presence of toxic metal(loid) concentrations, which are often considered markers of traffic-related pollution (Migaszewski et al. 2021). These markers include single elements such as Sb, Zn, Cu, and Pb, or multiple element markers such as Pt group elements (PGE). Recent studies have also focused on organic pollutants as potential traffic markers (Habre et al. 2021). There are many reports on the composition of RD in certain areas, mainly in large cities; however, there is a lack of evaluation of RD from petrol stations (Alsubaie et al. 2019). Urban RD, which consists of a complex mixture of particles from various sources, poses a significant threat to both the environment and human health. Despite growing concern about RD pollution, there remains a dearth of scientific investigations focused specifically on the elemental composition of RD at petrol stations (Rybak et al. 2022). To date, there have been no studies on hazardous elements in RD collected from petrol stations. This is a significant gap in the research, as petrol stations are known to be a potential source of pollution due to the handling and storage of petroleum products. The lack of research on this topic raises concerns about the possible impact of toxic elements on the environment, the health of petrol station workers and customers, as well as the equipment used to deliver fuel (Olowoyo et al. 2022). A possible scenario is the release of toxic elements into the environment through accidental spills or leaks from storage tanks or pipelines. Another scenario could involve the transfer of toxic elements from fuel pumps to the hands of workers and customers at the petrol station, leading to potential health risks. The most significant hypothetical scenario proposes that RD from cars, transported during refuelling, can lead to exposure to toxic elements. This exposure occurs through inhalation, not only during the refuelling process, but also during cleaning activities (Hilpert et al. 2019). This scenario highlights the potential risks associated with exposure to toxic elements for both petrol station employees and drivers, who may accidentally track dust containing these elements to their homes or other locations. Exposure to hazardous elements can have significant negative effects on human health, including respiratory and neurological problems, developmental delays, and cancer (Zamanian et al. 2018). Increasing industrial activities, the number of vehicles on the roads and the population in large cities cause air pollution in the urban environment and ultimately affect human health (Safiur Rahman et al. 2019; Rahman et al. 2021b). In recent decades, human activities have led to pollution from RD and roadside soil containing metal (oid) in urban areas (Rahman et al. 2021c). Moreover, in industrial areas, increased human activities generate high emissions of metals that contaminate the environment and eventually affect human health (Rahman et al. 2021a).
Thus, it is important to investigate the levels of toxic elements in the RD from petrol stations, as this information could be used to improve regulations and safety measures related to refuelling and cleaning. Despite the importance of investigating the levels of toxic elements in RD from petrol stations, this area of research has been largely overlooked.
Therefore, this study is a crucial first step to filling this knowledge gap and providing valuable information to consumers, petrol station operators, and environmental toxicologists. The study will involve collecting and analysing samples of RD (n = 20) from most petrol stations in the city of Rzeszów (Podkarpackie, Poland) to determine the levels of hazardous elements (Mo, U, As, Hg, Th, Pb, Ni, Cu, Cr, Be, Sr, Zr, Zn, Mn, Fe). By conducting such studies, we can better understand the sources and transport of toxic elements in the environment and develop strategies to minimise their impact. This information can also inform regulations and policies to protect human health and the environment. Petrol stations, which serve as major hubs for vehicular activities, are expected to exhibit elevated levels of various pollutants in the surrounding environment. Elemental analysis of RD at petrol stations will enable identification of pollution hotspots, highlighting areas of elevated contamination that require immediate attention and remediation. Therefore, it is imperative to investigate the concentration of toxic elements in RD collected from petrol stations and fill the current research gap (Li et al. 2017).
For this purpose, the elemental analysis of RD samples was performed using field portable X-ray fluorescence (FP-XRF) in direct mode. The main advantage of using an FP-XRF analyser is its ability to provide rapid, direct, and nondestructive elemental analysis of environmental samples. It allows the determination of multiple elements in a single measurement, making it a cost-effective and efficient technique for large-scale environmental studies. Additionally, FP-XRF analysers require minimal sample preparation and do not generate waste compared to traditional laboratory-based techniques such as inductively coupled plasma mass spectrometry (ICP-MS) (Parsons et al. 2013). Furthermore, the difficult, tedious, time-consuming, expensive, and environmentally burdensome step of sample digestion was omitted, as the direct in situ analysis mode was used. In general, the use of the FP-XRF methodology for the elemental analysis of RD samples from petrol stations provides a fast, reliable, ‘white’ and cost-effective method to obtain information on the composition of potentially hazardous materials in the environment. The FP-XRF technique aligns with the principles of white analytical chemistry (WAC), making it a ‘white analytical technique’. WAC, an extension of green analytical chemistry, considers ecological factors alongside analytical (red) and practical (blue) criteria to ensure method quality. Analogous to the RGB colour model where red, green, and blue light combine to produce white, the white analytical method embodies harmony among its analytical, ecological, and practical characteristics (Kobylarz et al. 2023). This information is crucial to assessing the potential risk to human health and the environment and developing effective strategies to mitigate this risk. This study aims to elucidate the importance of performing a hazardous elements analysis of RD in petrol stations using FP-XRF for the first time from an environmental and human health point of view. Using FP-XRF as a ‘white’ analytical technique, we can assess the presence and concentrations of hazardous elements within the dust of the petrol station, thus contributing to a comprehensive understanding of associated risks and possible mitigation strategies without using classical methods (ICP-MS, ICP-OES, AAS).
The idea of the conducted research is presented schematically in the form of five main stages (A–E) as a workflow in Fig. 1.
Materials and Methods
Samples Collection and Storage
To collect the samples, clear zip-lock bags were held close to the ground and a sufficient amount of RD was collected using a hand brush. Dust was collected directly along the fuel dispenser (dust usually accumulates in areas sheltered from the wind, along the distributor threshold). The selection of the sampling area location (in front of the distributor threshold) was dictated by practical considerations, an important environmental exposure, and based on an interview with petrol station employees (where they find dust the most and most often during cleaning). This location is very useful, as it gives exposure to both the station’s customers (one-time/accidental exposure) and the people working there (occupational/continuous exposure). The brush was gently wiped on the bag to remove any loose particles. Samples were collected during the day to avoid moisture accumulation due to dew formation. The sampling process was carried out consistently at all petrol stations (n = 20) to ensure uniformity in the collected samples. After the samples were collected, they were immediately sealed and labelled with the appropriate station number and location information from the Global Positioning System. The localisation of the collected samples summarised on the map—Fig. 2. More details about the investigated samples are summarised in Table S1 (see Supplementary Information 1; SI1). Samples were stored in cardboard packaging to protect them from external contamination and ensure their integrity until they were analysed in the laboratory. Before XRF measurement, the samples were transferred to polyethylene XRF sample cups with a depth of 20 mm. For smaller samples, quartz wool was added to ensure full contact with the polyester film at a minimum depth of 10 mm. As was mentioned in the introduction, Fig. 1 illustrates the process of: A) collection of RD samples, B) storing of samples, C) preparation of XRF sample cups, D) measurement by the FP-XRF technique and E) toxicological risk assessment.
Measurement of Investigated Elements by FP-XRF Spectrometer
The determination of hazardous elements (Mo, U, As, Hg, Th, Pb, Ni, Cu, Cr, Be, Sr, Zr, Zn, Mn, and Fe) was made by applying the FP-XRF spectrometer (Niton XL3t 950 He GOLDD + portable XRF spectrometer, Thermo Scientific). A methodological approach was used based on parameters from our previously published articles by Milan and Jurowski (2024) and Frydrych and Jurowski (2023). The instrument was placed nose-up on a shielded laboratory test stand and remotely activated by a laptop via a USB connection. Samples were placed centrally on the 8 mm (50 mm2) X-ray beam, with the polyester film facing downward, and counted for 90 s (3 × 30 s) in a low-density standardless soil mode (40 μA and 50 kVp). The measurements were repeated at three different locations toward the perimeter of each sample surface. The measured spectra obtained were quantified by coefficients of fundamental parameters to determine the concentrations of hazardous elements in μg/g, with a measurement error of 2σ (95% confidence). Results were downloaded to the laptop via Niton data transfer software (NDT). The use of an FP- XRF spectrometer in low-density soil mode was appropriate for the measurement of trace elements in the samples of RD samples. Additionally, this technique allows for the measurement of samples in situ, which is important for field environmental studies. The calibration was verified throughout the measurements by analysis of Niton reference materials: multielement reference samples (NIT-500-705, QC Standard) 1) 180-694A, SdAR-L2 Soil, Low Metals, PP (QC Standard), 2) 180-647, SiO2 (99.995% PP), 3) 180-706 pp, USGS SdAR-M2 (Control Sample), 4) 180-661, RCRApp 05092201 (1000Ba, 500Ag, As, Cd, Cr, Pb, Se). The measured levels of the reference samples were within 5% of the specified values, ensuring the precision of the results obtained from the RD samples.
Toxicological Risk Assessment of Hazardous Elements in Road Dust from Petrol Stations and Health Risk Assessment
A comprehensive risk assessment was carried out to assess the potential human exposure to elements contained in gas station RD. The methodology used in this study is consistent with the model methodology proposed in the work of Faiz et al. (2012) and Zheng et al. (2015) using the assumptions of the Environmental Protection Agency (EPA). To determine the elements that RD from gas stations can expose people to, a thorough risk assessment was conducted. The approach taken in this study is in line with the model approach put forward employing EPA assumptions in the work of Faiz et al. Our investigations include a thorough evaluation of health endpoints, both cancer and non-cancer, in line with this approach. This comprehensive evaluation takes into account possible exposures that align with the findings of Faiz et al. It should be mentioned, however, that the focus of our research is specifically on RD from Rzeszów petrol stations. This distinction is crucial because, for example, latitude can have an impact on the characteristics and makeup of RD. As a result, our study offers important insights into the possible dangers of elemental contaminants from RD, helping to improve knowledge of the material’s health concerns in a more localised manner. A person can potentially be exposed to this RD while refuelling (during a gust of wind, the dust can be suspended in the air and can be inhaled during refuelling). From the point of view of worker exposure, this is also very important, as this dust is cleaned up by sweeping from this area (sweeping produces smoke and dust with suspended RD that can be inhaled during sweeping).
Non-Carcinogens Dose
The following relationships are used to estimate the non-cancerous dose of heavy metals for each of the three equations (Eqs. 1–3) (Faiz et al. 2012):
where:
AT Averaging time: for carcinogens ED × 365 days; for non-carcinogens 70 × 365 = 25550 days (Ferreira-Baptista and De Miguel 2005), BW Average body weight; 15 kg (US EPA 2015), C Concentration of trace element in RD (exposure point concentration), ED Exposure duration of adult; 24 years (US EPA 2015), EF Exposure frequency (site specific); 180 day year−1 (US EPA 2015), IngR Ingestion rate; 200 mg day−1 (US EPA 2015); InhR Inhalation rate; 7.6 m3 day−1 (US EPA 2015); PEF Particle emission factor; 1.36 × 109 m3 kg−1 (US EPA 2015).
Since site-specific biometric factors are not readily available, the US-EPA has provided the values for these components. Doses of non-carcinogens were collected through ingestion, inhalation, and skin contact and provided for the current investigation. The study includes the cumulative dose of non-carcinogen metals by eating, inhalation, and skin contact.
Carcinogens Dose
The lifetime average daily dose (LADD) for carcinogens (As, Cd, Co, Cr, Ni, etc.) is used to estimate the cancer risk. For every exposure path, LADD is computed as a weighted average using the following equation (Eq. 4) (Faiz et al. 2012):
where:
AT Averaging time: for carcinogens ED × 365 days; for non-carcinogens 70 × 365 = 25550 days (Ferreira-Baptista and De Miguel 2005); BWadult Body weight of adult (US EPA 2015); BWchild Body weight of child (US EPA 2015); C Concentration of trace element in RD (exposure point concentration); CR Contact (absorption) rate, IngR Ingestion CR, InhR Inhalation CR, Dermal CR = SA × SL × ABS; EDadult Exposure duration of adult; 24 years (US EPA 2015); EDchild Exposure duration (site specific); 6 years (US EPA 2015); EF Exposure frequency (site specific); 180 day year−1 (US EPA 2015); LADD values were calculated for carcinogens.
Health Risk Assessment
As will be discussed later, health risk assessment can be performed using the patterns that that are given here.
Hazard Index (HI)
HI is a number that considers the combined effect of doses received through ingestion, inhalation, and dermal contact. It is described as (Eq. 5) (Faiz et al. 2012):
where Di and RfD stand for element I dose and, respectively, the corresponding reference dose of the elemet (Ferreira-Baptista and De Miguel 2005). The non-cancer risk, or hazard quotient (HQ), is the ratio Di:RfD (Ferreira-Baptista and De Miguel 2005). The risk of elements from ingestion, inhalation, and skin contact is estimated using HI. HI places a safe limit on the noncancerous risk of elemental dose such that HI > 1 is not recommended. The EPA provided the reference dose value for ingestion, inhalation, and dermal contact, which was used to compute the HI values (US EPA 2015).
Hazard Quotient (HQ).
By dividing the non-cancer dose by the chronic reference exposure level of each metal, we were able to calculate the non-cancer HQ for both the dermal and ingestion pathways. Only the metal(oid)s for which chronic reference dose data were available in the literature (As, Cr, Cu, Fe, Mo, Ni, Pb, U, Zn, Zr) could have their HQ calculated (see Supplementary Information SI3). It is described as (Eq. 6) (Faiz et al. 2012):
If HQ or HI < 1, there will be no obvious risk to the exposed population from exposure to element in RD from the petrol station. If HQ or HI ≥ 1, the risk will be considered unacceptable. As HQ or HI increases, the risk also increases.
Results and Discussion
Hazardous Element Profile of Road Dust from Petrol Stations in Rzeszów (Podkarpackie, Poland)
The level (μg/g) of all hazardous elements investigated (Mo, U, As, Hg, Th, Pb, Ni, Cu, Cr, Be, Sr, Zr, Zn, Mn and Fe) in all samples (n = 20) is shown in Fig. 3 as the profile of hazardous elements in RD from petrol stations in Rzeszów (Podkarpackie, Poland). Descriptive statistics (presence in samples, minimum, maximum, mean) are shown in Table 1.
The results showed that all RD samples tested (n = 20) contained Mo, Zr, Sr, U, Th, Pb, As, Hg, Zn, Cu, Ni, Fe, Mn, Cr, Ti, Sc, and Ba. RD collected from petrol station distributors in Rzeszów is characterised by an averaged elemental composition. Fe (9357.41 ± 117.43 µg/g), Ti (2334.56 ± 79.75 µg/g), Mn (181.58 ± 36.02 µg/g), Zn (165.88 ± 9.44 µg/g), Zr (109.18 ± 3.09 µg/g), Sc (106.35 ± 8.11 µg/g), Sr (89,70 ± 2,71 µg/g), Ba (79.95 ± 33.18 µg/g), Cr (46.61 ± 16.07 µg/g), Cu (31.44 ± 2.33 µg/g), Ni (26.07 ± 6.40 µg/g), Pb (11.52 ± 3.48 µg/g), Th (11.37 ± 2.79 µg/g), Hg (7.98 ± 1.53 µg/g), As (5.79 ± 1.69 µg/g), U (5.07 ± 0.81 µg/g) and Mo (4.61 ± 0.44 µg/g).
Interestingly, a test sample (RD1) contained uranium (5.07 ± 0.81 µg/g). Only three samples tested (RD4, RD5, RD14) contained Ba in their composition (the most in the RD14 sample 114.16 µg/g ± 27.09). Surprisingly, the samples RD1, RD2, RD 4, RD6, RD7, RD8 and RD20 contained arsenic (As) in a mean concentration mean 5.79 ± 1.69 μg/g. These elements are commonly associated with vehicle emissions, fuel combustion, and tyre and brake wear. Another research indicated that RD contained high levels of As, prompting a thorough examination of the factors that influence it and possible health hazards. This investigation involved integrating physical and chemical data, mineralogical analysis, multivariate analysis, and a simulated probabilistic risk assessment model (Rahman et al. 2022). Furthermore, the presence of heavy metals (As, Pb, and Hg) indicates the potential contribution of gasoline and diesel combustion, as well as other anthropogenic activities near the petrol stations. Their presence underscores the importance of implementing pollution control measures to mitigate the potential health risks posed by prolonged exposure to these contaminants.
Toxicological Risk Evaluation of Components Under Investigation
We test RD samples from gas stations because they contain potentially toxicity substances. The method outlined in “Toxicological Risk Assessment of Hazardous Elements in Road Dust from Petrol Stations and Health Risk Assessment” was used to calculate the non-cancer and cancer exposure doses for each element discovered in RD samples. The results of exposure dose estimates, accounting for oral, dermal, and inhalation routes, for both cancer and non-cancer scenarios are shown in Tables 2, 3, 4. The average concentrations for both were calculated.
Dose calculations are based on certain toxicity standards. Due to the inaccessibility of site-specific biometric factors, the values for these factors were taken from the US EPA (2015). The above expressions were derived to provide conservative dose estimates so that remedial actions can be taken to safely impact human health at the gas station. Non-carcinogens doses from ingestion, inhalation, and dermal contact were obtained for the current study and are given in Table 2. Mean dose resulting from ingestion, inhalation and dermal contact of non-carcinogenic metals are also given in this table. From this data it can be seen that a maximum dose of 5.79 × 10–7 mg/(kg day) and a minimum dose of 2.07 × 10–4 mg/(kg day) were obtained for elements Cu. Elements Fe and Zn have ingestion dose in 6.15 × 10–2 and 1.09 × 10–3 mg/(kg day) range with dose for Ni is 4.80 × 10–7 mg/(kg day) of dermal range. For lead, the average dose is 2.53 × 10–5 mg/(kg day). Lead was identified in the study despite the availability of unleaded gasoline. Since most elements are found in soil, the presence of exposed soil sections close to the sampling location affects the likelihood of exposure to those elements. The dose of most of the elements from the three modes lies within the threshold range of 10−2–10−67 mg/(kg day) which is considered safe. Inhalation doses are higher (10–12–10–16 mg/(kg day). The suspension of dust in inhaled air seems to be particularly dangerous taking into account the results obtained and environmental factors (US EPA 2015).
The LADD values for carcinogens were calculated and are given in Table 3. These data show that the mean LADD ranges between 1.11 × 10–2 mg/(kg day) for Zn and 5.34 × 10–5 mg/(kg day). Sources of these elements include industries that require monitoring and regulation to reduce the risk to people who come into contact with these exposure points when refuelling.
Higher HI values are obtained for Fe, Mo, Cu using maximum and minimum values 8.81 × 10–3 mg/(kg day) and 5.18 × 10–3 mg/(kg day). The HQ (HQing, HQinh, HQder) and HI values for lead are: 2.16 × 10–2, 6.05 × 10–13, 6.06 × 10–5 and 2.17 × 10–2 (for HI). For all three pathways, the average metal HQ values decline as follows: ingestion > dermal contact > inhalation. This ranking agrees with the findings of other research (Khairy et al. 2011; Du et al. 2013). These results mean that adults are not exposed to potential non-cancer health risks from heavy metals in RD when refueling their vehicles. Similar results were obtained in work related to contact with RD while at a bus stop (Wei et al. 2015).
Assessment of Hazardous Elements Including Environmental Toxicology Context
RD, which consists of a heterogeneous mixture of particles derived from various vehicle emissions, tyre wear, road abrasion, and atmospheric deposition, has gained considerable attention due to its detrimental impacts on environmental quality and public health. Although previous studies have investigated the composition and health effects of RD in general, there is a distinct knowledge gap regarding the elemental characterisation of RD specifically obtained from petrol stations. This novel research seeks to address this gap by evaluating the elemental constituents of petrol station dust and its potential implications for the environment and human well-being. So far, no studies have focused on a detailed elemental description of RD found at petrol stations; this dust can come from both soil and cars or fuels and exhaust gases. Degradation of road paints releases large amounts of glass microbeads into RD, which is then washed away and carried by the wind into the environment. Dust microbeads have many characteristics of the best technogenic marker of traffic pollution, e.g., microscopic dimensions, low density, high thermal stability, and good weather resistance (Migaszewski et al. 2021). We suspect that this dust can pollute the environment and threaten people (inhalation exposure during refuelling by customers or during dust sweeping by employees).
It is worth paying attention to the presence of Pb in the range of 3.78 μg/g ± 3.05–45.930 ± 2.87 μg/g, which may be characteristic of the dust that comes from the fuel stations. It should be taken into account that from the 1960s Pb was removed from gasoline, but removal was completed in 2021 (Angrand et al. 2022). According to the US Environmental Protection Agency, Pb may be from the lead paint used (eg, painted fuel pump kerb edges) that has deteriorated in the form of chips and dust or from previous renovation activities and has ended up in lead dust or from the past (eg, dust coming from the vicinity of the road as a result of emissions into the air from vehicles fuelled with leaded petrol) (US EPA 2013). Lead is ingested and inhaled into the body through sources such as soil, food, lead dust, everyday goods and the workplace (Charkiewicz and Backstrand 2020). The authors of this research emphasise that toxic exposure to Pb remains a significant public health problem in Poland. Because toxic components such as lead are so detrimental to human health and even life, it is especially crucial to continuously advance understanding in this area. The amounts of exposure and absorption must be established in the case of Pb because the symptoms of Pb poisoning frequently do not manifest for several years. Insoluble lead compounds have been associated with airway inflammation, which can lead to airway cancer (Khan and Strand 2018). Deficits in childhood neurobehavioral and cognitive development are known to be caused by this element in home dust. According to Potgieter-Vermaak et al. (2012), exposure to RD poses dangers, since it contains Pb and Cr. Our studies showed the presence of Cr in 13 samples (RD1, RD5–RD11 and RD13, RD16, RD20, RD21 (in the range 22.94 ± 15.23 µg/g–94.68 ± 16.10 µg/g). Lead chromate was commonly used as a pigment on coloured road signs until restrictions led to the development of safer alternatives (Turner and Filella 2023). In this study, chromium was detected (> 5–50 mg/kg) in 81 samples at concentrations of 20–20,000 mg/kg and most often in yellow paints, and the concentrations covariate with the concentrations of Pb (Turner and Filella 2023). Li et al. (2015) evaluated the health effects of RD, discovering that children who lived close to industrial regions had a higher risk of exposure to Pb, Cr and Cu. Non-carcinogenic health risks were associated with the presence of higher concentrations of Ba, Pb, and Cu n RD in high-traffic areas (Liu et al. 2014). Short-term exposure to Cr has been found to trigger allergic responses and respiratory discomfort in humans. Lung cancer has been associated with exposure to Cr for an extended period (Wilbur et al. 2012). Publications from the 1990s indicate the occurrence of respiratory tract cancer in petrol station employees (Grandjean and Andersen 1991; Lagorio et al. 1994). Dust curing can cause allergic reactions such as coughing and sneezing, as well as pulmonary fibrosis (Habybabady et al. 2018). Huang et al. found that As is the hazardous element in their assessment of the risk posed by the ingestion and inhalation of these substances. The risk of cancer associated with exposure to this element through RD was high in children (Xu et al. 2013). As has been linked in a meta-analysis to conditions such as anemia and leukopenia, as well as cardiovascular disease, birth defects, neurological and cognitive problems, diabetes, ototoxicity, and peripheral vascular diseases. In the mentioned study, a correlation was also discovered between increased amounts of arsenic exposure and lung cancers (Kaur et al. 2011). Elemental pollutants of RD can also come from road wear and urban dust mainly generated by construction emissions), engine exhaust, brake wear, and tyre wear (Amato et al. 2011). According to Apeagyei et al. (2011), the Zn and Ca present in the RD samples were probably related to tyre dust. The results of the measurements carried out on worn brake pads showed high concentrations of, for example, Fe (mean 28.091 µg/g), Ca (mean 9185 µg/g), and Pb (73 µg/g). The authors compared dust from urban roads with dust from rural roads; Fe and Ti were significantly higher in dust from urban roads (Apeagyei et al. 2011). Industrial activity has a different effect on metal concentrations in soil and dust, with the highest concentrations of Pb (373 mg/kg) and Zn (1022 mg/kg) found in the refining industry (Gabarrón et al. 2017). An environmental study conducted in Beijing (China) in 2020 found that the use of pesticides and fertilisers and traffic-related exhaust fumes were identified as critical sources of heavy metals in the spring (Men et al. 2020). A study by Luo et al. (2022) in key cities in northwest China showed that contamination in Pb dust storms (eg mean 46.2 mg/kg) and Zn (233.2 mg/kg) was mainly from transport sources. Cd (5.1 mg/kg) and Pb (146.6 mg/kg) from Jinan RD (China) may have a potential pathogenic risk (Wang et al. 2023). The authors of the cited papers propose that the regions and sources of pollution that contribute to the increase in Pb and Cd levels should be monitored. Taking into account the data from the cited literature and our results, it can be assumed that the tested samples of RD from petrol stations are characteristic of traffic-related pollution, for example, Fe and Zr from vehicle brake linings (Apeagyei et al. 2011; Migaszewski et al. 2021). Taking this as a premise, we decided to assess whether the Fe:Zr ratio could be singled out as a potential marker of traffic-related pollution. In our study, Fe: Zr ratio (Supplementary Information 2, SI2) showed that the highest ratio in the RD1 sample RD1 (No 1 from Fig. 1; Fe: Zr = 190.3) and the smallest ratio was in the RD7 sample RD7 (No 7 from Fig. 1; Fe: Zr = 78.4). The results are highly divergent, with no overlap in the range of zones defined in Fig. 1. It cannot be stated that they are in the same or similar ratio in individual samples of RD. An attempt was made to assess the critical assessment of the Fe: Zr ratio to assess the hot spots of brake lining contamination and assess the hot zone and the cold zone of elemental contamination in the city of Rzeszów. These zones could not be assessed. There is no doubt that the investigated petrol stations constitute a hot spot, but the resulting hot spots do not form distinctive combinations that could form the basis for defining the relevant exposure zones (no analogies). Therefore, a critical analysis of the results leads to the conclusion that petrol stations in Rzeszow constitute hot spots, but characteristic zones of exposure to hazardous elements cannot be defined. Research conducted in Xi’an (China) on the analysis of metals in RD from petrol stations showed that Mn and Ni come mainly from natural sources; Cr, Cu, Pb, Cr and Zn come from traffic poles (Li et al. 2017). FP-XRF elemental analysis of RD at Rzeszów petrol stations can identify pollution hotspots, indicating increased areas of pollution areas requiring immediate attention and corrective action in the city area. Such results were obtained in the work of Li et al. (2017)—the high concentration of heavy metals is located on the inner and second ring road of Xi’an, China. Furthermore, the metal content in the south is higher than in the north. In these studies, Ni, for example, was shown to average 33.05 mg/kg, Pb 96.63 mg/kg (Li et al. 2017). Another study refers to the fact that heavy metals in particulate matter in another part of the world have a significant impact because particulate matter can penetrate human organs and systems, causing a number of diseases (Mahmoud et al. 2023). This study assessed dust samples from 20 different types of roads in a busy area of Doha during the winter of 2016–2017. The study used indicators of heavy metal pollution levels such as Igeo and IPI. Igeo was found to be moderate to highly polluted, except for Cu. The IPI indicated a high level of pollution, with Zn being the most abundant pollutant. Mahmoud et al. (2023) proposes conducting intensive research to investigate the chemical behaviour and sources of heavy metals in urban dust to enable comprehensive control of particulate matter emissions, which is consistent with our approach.
There are studies relating to cities in Poland, which may be particularly helpful in interpreting our study, taking into account environmental, geographical, and legal factors. The study by Zgłobicki and Telecka (2021) assessed the level of hazard and cancer risk index for Cd, Cr, Cu, Ni, Pb and Zn contained in street dust collected in 2013 and 2018 in 62 points located in various parts of a small/medium-sized city (Lublin, Poland). For example, in relation to our results, the study confirms that the average hazard index (HI) for the individual elements included in the cited study and ours reached very low levels (< 0.01).
In our study, observing the correlations between petrol stations in the city centre and the suburbs, it cannot be said that those in the city centre are more polluted. The results of individual elements from different stations are characterised by different levels of impurities. The research we propose may be helpful in the analysis of the current and implementation of new urban pollution control areas in the context of road transport. This is related to external and internal guidelines on maintaining an appropriate level of cleanliness and taking care of the intervals to maintain the cleanliness of general accessible places in petrol stations with RD in the context of environmental and public health regulations. Identifying and quantifying specific hazardous elements present in petrol station dust can inform the design and implementation of effective pollution control measures. By understanding the contribution of various elements to overall levels of pollution, appropriate mitigation strategies can be devised, such as better vehicle maintenance practices, emission control technologies and improved dust control measures, to reduce environmental contamination and minimise human health risks.
Conclusions
The elemental analysis by FP-XRF spectroscopy as a ‘white analytical technique’ of RD collected from petrol stations in Rzeszów (Podkarpackie, Poland) represents a significant scientific endeavour with substantial environmental quality and human health implications. The novelty of study lies in its pioneering application of FP-XRF as a ‘white analytical technique’ for the rapid, direct and non-destructive analysis of hazardous elements in RD, setting a new standard for environmental assessments. The findings of Rzeszów provide a critical foundation for identifying pollution hotspots and determining sources of traffic-related pollution, particularly potential markers such as Fe and Zr derived from brake linings. This information is invaluable for source allocation, exposure, and health risk assessments, facilitating the formulation of targeted mitigation strategies.Our studies of hazardous elements in the dust of petrol stations have allowed the identification of primary sources that contribute to pollution in the vicinity. Based on our critical research, it was not possible to determine the hot zone in Rzeszów in which pollution in the city centre is higher than in the suburbs, but it is possible to observe places where the Fe/Zr ratio is the highest where it can be identified as a potential marker of traffic-related pollution. Therefore, it can be suspected that Fe and Zr are potential markers of brake lining contamination. This knowledge can facilitate targeted interventions and regulatory measures to mitigate specific pollutant emissions, thus improving overall environmental health. These findings are expected to guide policymakers, regulatory bodies, and stakeholders in adopting evidence-based measures to reduce pollution, protect the environment, and protect the well-being of individuals living or working near petrol stations. An important solution proposed by Isaifan and Baldauf (2020) is to plant more trees, which can influence the concentration of air pollutants we breathe by directly removing them or avoiding emissions and secondary formation of pollutants in the atmosphere. It will be crucial to consider detailed development plans and other related documents not only within the city in our study (Rzeszow, Poland) but also general guidelines for approving residential and school buildings near main roads and petrol stations. Identifying and determining hazardous elements in petrol stations provides valuable information to assess possible human exposure. Understanding the pathways through which these contaminants can enter the human body is a future perspective for evaluating associated health risks and implementing effective risk management strategies. Undoubtedly, this is a complex environmental problem; therefore, more research is needed to solve this problem. Research presents a challenge because of the large number of variable factors that can affect the quantitative and qualitative composition of RD. When it comes to non-cancerous effects, eating is the primary mode of exposure, followed by skin contact and breathing in gas station RD. Both carcinogenic and non-cancer health risks are within the acceptable range.
This study underscores the importance of developing evidence-based policies and regulatory frameworks to minimise environmental pollution and protect public health, particularly for people residing or working near petrol stations. Policymakers, regulatory authorities, and stakeholders are urged to take advantage of these findings to formulate targeted measures that address identified pollution sources and hotspots. Moreover, emphasising the need for further research, our results study recommends expanding the scope of environmental assessments to include a broader range of pollutants and employing not only FP-XRF but other portable instruments (eg. portable gas chromatographs, portable Infrared/Raman spectrometers) for a more comprehensive evaluation of environmental risks. Future studies should also explore the effectiveness of pollution control technologies and dust management practices in reducing exposure risks.
Lastly, for the first time we applied and planned environmental studies using FP-XRF as a ‘white analytical technique’ for direct and rapid toxicological analysis of hazardous elements in RD. Our study details a robust methodology for sample collection, preparation, and analysis using FP-XRF, serving as a procedural blueprint for environmental scientists and researchers undertaking similar assessments. The streamlined approach ensures high-quality, reliable data acquisition, crucial for accurate environmental and toxicological evaluations.
Data Availability
The raw data supporting the conclusion of this article will be made available by the authors without undue reservation.
Abbreviations
- EPA :
-
The environmental protection agency
- FP-XRF :
-
Field portable X-ray fluorescence
- HI :
-
Hazard index
- HQ :
-
Hazard quotient
- ICP-MS :
-
Inductively coupled plasma mass spectrometry
- LADD :
-
Lifetime average daily dose
- NDT :
-
Niton data transfer software
- PGE :
-
Pt group elements
- RD :
-
Road dust
- WAC :
-
‘White analytical technique’
References
Alsubaie A, Jaafar M, Al-Dabbous AN et al (2019) A comparison of elemental presence in UK and Kuwait road dust. Radiat Phys Chem 155:341–347. https://doi.org/10.1016/j.radphyschem.2018.08.020
Amato F, Pandolfi M, Moreno T et al (2011) Sources and variability of inhalable road dust particles in three European cities. Atmos Environ 45:6777–6787. https://doi.org/10.1016/j.atmosenv.2011.06.003
Angrand RC, Collins G, Landrigan PJ, Thomas VM (2022) Relation of blood lead levels and lead in gasoline: an updated systematic review. Environ Health 21:138. https://doi.org/10.1186/s12940-022-00936-x
Apeagyei E, Bank MS, Spengler JD (2011) Distribution of heavy metals in road dust along an urban-rural gradient in Massachusetts. Atmos Environ 45:2310–2323. https://doi.org/10.1016/j.atmosenv.2010.11.015
Charkiewicz AE, Backstrand JR (2020) Lead toxicity and pollution in Poland. Int J Environ Res Public Health 17:4385. https://doi.org/10.3390/ijerph17124385
Du Y, Gao B, Zhou H et al (2013) Health risk assessment of heavy metals in road dusts in urban parks of Beijing, China. Procedia Environ Sci 18:299–309. https://doi.org/10.1016/j.proenv.2013.04.039
Faiz Y, Siddique N, Tufail M (2012) Pollution level and health risk assessment of road dust from an expressway. J Environ Sci Health A Tox Hazard Subst Environ Eng 47:818–829. https://doi.org/10.1080/10934529.2012.664994
Ferreira-Baptista L, De Miguel E (2005) Geochemistry and risk assessment of street dust in Luanda, Angola: a tropical urban environment. Atmos Environ 39:4501–4512. https://doi.org/10.1016/j.atmosenv.2005.03.026
Frydrych A, Jurowski K (2023) Portable X-ray fluorescence (pXRF) as a powerful and trending analytical tool for in situ food samples analysis: a comprehensive review of application - State of the art. TrAC, Trends Anal Chem 166:117165. https://doi.org/10.1016/j.trac.2023.117165
Gabarrón M, Faz A, Acosta JA (2017) Effect of different industrial activities on heavy metal concentrations and chemical distribution in topsoil and road dust. Environ Earth Sci 76:1–13. https://doi.org/10.1007/s12665-017-6449-4
Grandjean P, Andersen O (1991) Lung cancer in filling station attendants. Am J Ind Med 20:763–768. https://doi.org/10.1002/ajim.4700200607
Habre R, Girguis M, Urman R et al (2021) Contribution of tailpipe and non-tailpipe traffic sources to quasi-ultrafine, fine and coarse particulate matter in southern California. J Air Waste Manage Assoc 71:209–230. https://doi.org/10.1080/10962247.2020.1826366
Habybabady RH, Sis HN, Paridokht F et al (2018) Effects of dust exposure on the respiratory health symptoms and pulmonary functions of street sweepers. Malays J Med Sci 25:76–84
Hilpert M, Rule AM, Adria-Mora B, Tiberi T (2019) Vent pipe emissions from storage tanks at gas stations: implications for setback distances. Sci Total Environ 650:2239–2250. https://doi.org/10.1016/j.scitotenv.2018.09.303
Isaifan RJ, Baldauf RW (2020) Estimating economic and environmental benefits of urban trees in desert regions. Urban for Urban Green. https://doi.org/10.3389/fevo.2020.00016
Kaur S, Kamli MR, Ali A (2011) Role of arsenic and its resistance in nature. Can J Microbiol 57:769–774. https://doi.org/10.1139/w11-062
Khairy MA, Barakat AO, Mostafa AR, Wade TL (2011) Multielement determination by flame atomic absorption of road dust samples in Delta Region Egypt. Microchem J 97:234–242. https://doi.org/10.1016/j.microc.2010.09.012
Khan RK, Strand MA (2018) Road dust and its effect on human health: a literature review. Epidemiol Health 40:e2018013. https://doi.org/10.4178/epih.e2018013
Kobylarz D, Michalska A, Jurowski K (2023) Field portable X-ray fluorescence (FP-XRF) as powerful, rapid, non-destructive and ‘white analytical tool’ for forensic sciences - State of the art. TrAC, Trends Anal Chem 169:117355. https://doi.org/10.1016/j.trac.2023.117355
Lagorio S, Forastiere F, Iavarone I et al (1994) Mortality of filling station attendants. Scand J Work Environ Health 20:331–338. https://doi.org/10.5271/sjweh.1389
Li X, Shi X, Wang A, Li Y (2017) Heavy metals contamination and assessment in gas station dust of Xi’an, a mega-city of China. Environ Earth Sci 76:288. https://doi.org/10.1007/s12665-017-6582-0
Li P, Xin J, Wang Y et al (2015) Association between particulate matter and its chemical constituents of urban air pollution and daily mortality or morbidity in Beijing City. Environ Sci Pollut Res Int 22:358–368. https://doi.org/10.1007/s11356-014-3301-1
Liu E, Yan T, Birch G, Zhu Y (2014) Pollution and health risk of potentially toxic metals in urban road dust in Nanjing, a mega-city of China. Sci Total Environ 476–477:522–531. https://doi.org/10.1016/j.scitotenv.2014.01.055
Luo H, Wang Q, Guan Q et al (2022) Heavy metal pollution levels, source apportionment and risk assessment in dust storms in key cities in Northwest China. J Hazard Mater 422:126878. https://doi.org/10.1016/j.jhazmat.2021.126878
Mahmoud N, Al-Shahwani D, Al Thani H, Isaifan R (2023) Risk assessment of the impact of heavy metals in urban traffic dust on human health. Atmosphere 14:1049. https://doi.org/10.3390/atmos14061049
Men C, Liu R, Xu L et al (2020) Source-specific ecological risk analysis and critical source identification of heavy metals in road dust in Beijing, China. J Hazard Mater 388:121763. https://doi.org/10.1016/j.jhazmat.2019.121763
Migaszewski ZM, Gałuszka A, Dołęgowska S, Michalik A (2021) Glass microspheres in road dust of the city of Kielce (south-central Poland) as markers of traffic-related pollution. J Hazard Mater 413:125355. https://doi.org/10.1016/j.jhazmat.2021.125355
Milan J, Jurowski K (2024) Hazardous elements in plastic and rubber granules as infill material from sports facilities? Field Portable-XRF spectroscopy as ‘white analytical technique’ reveals hazardous elements in fall sports facilities in Rzeszów (Podkarpackie, Poland). Sci Total Environ 916:170280. https://doi.org/10.1016/j.scitotenv.2024.170280
Olowoyo JO, Lion N, Unathi T, Oladeji OM (2022) Concentrations of Pb and other associated elements in soil dust 15 years after the introduction of unleaded fuel and the human health implications in Pretoria, South Africa. Int J Environ Res Public Health 19:10238. https://doi.org/10.3390/ijerph191610238
Parsons C, Margui Grabulosa E, Pili E et al (2013) Quantification of trace arsenic in soils by field-portable x-ray fluorescence spectrometry: considerations for sample preparation and measurement conditions. J Hazard Mater 262:1213–1222. https://doi.org/10.1016/j.jhazmat.2012.07.001
Potgieter-Vermaak S, Rotondo G, Novakovic V et al (2012) Component-specific toxic concerns of the inhalable fraction of urban road dust. Environ Geochem Health 34:689–696. https://doi.org/10.1007/s10653-012-9488-5
Rahman MS, Jolly YN, Akter S et al (2021a) Sources of toxic elements in indoor dust sample at export processing zone (EPZ) area: Dhaka, Bangladesh; and their impact on human health. Environ Sci Pollut Res 28:39540–39557. https://doi.org/10.1007/s11356-021-13167-3
Rahman MS, Kumar P, Ullah M et al (2021b) Elemental analysis in surface soil and dust of roadside academic institutions in Dhaka city, Bangladesh and their impact on human health. Environ Chem Ecotoxicol 3:197–208. https://doi.org/10.1016/j.enceco.2021.06.001
Rahman MS, Kumar S, Nasiruddin M, Saha N (2021c) Deciphering the origin of Cu, Pb and Zn contamination in school dust and soil of Dhaka, a megacity in Bangladesh. Environ Sci Pollut Res 28:40808–40823. https://doi.org/10.1007/s11356-021-13565-7
Rahman MS, Saha N, Kumar S et al (2022) Coupling of redundancy analysis with geochemistry and mineralogy to assess the behavior of dust arsenic as a base of risk estimation in Dhaka Bangladesh. Chemosphere 287:132048. https://doi.org/10.1016/j.chemosphere.2021.132048
Rybak J, Wróbel M, Krzyżyńska R et al (2022) Is Poland at risk of urban road dust? Comparison studies on mutagenicity of dust. Environ Pollut 314:120337. https://doi.org/10.1016/j.envpol.2022.120337
Safiur Rahman M, Khan MDH, Jolly YN et al (2019) Assessing risk to human health for heavy metal contamination through street dust in the Southeast Asian Megacity: Dhaka, Bangladesh. Sci Total Environ 660:1610–1622. https://doi.org/10.1016/j.scitotenv.2018.12.425
Turner A, Filella M (2023) Lead and chromium in European road paints. Environ Pollut 316:120492. https://doi.org/10.1016/j.envpol.2022.120492
US EPA O (2013) Protect your family from sources of lead. https://www.epa.gov/lead/protect-your-family-sources-lead. Accessed 22 May 2023
US EPA O (2015) Superfund soil screening guidance. https://www.epa.gov/superfund/superfund-soil-screening-guidance. Accessed 12 Dec 2023
Wang X, Liu E, Yan M et al (2023) Contamination and source apportionment of metals in urban road dust (Jinan, China) integrating the enrichment factor, receptor models (FA-NNC and PMF), local Moran’s index, Pb isotopes and source-oriented health risk. Sci Total Environ 878:163211. https://doi.org/10.1016/j.scitotenv.2023.163211
Wei X, Gao B, Wang P et al (2015) Pollution characteristics and health risk assessment of heavy metals in street dusts from different functional areas in Beijing, China. Ecotoxicol Environ Saf 112:186–192. https://doi.org/10.1016/j.ecoenv.2014.11.005
Wilbur S, Abadin H, Fay M et al (2012) Toxicological profile for chromium. Agency for Toxic Substances and Disease Registry (US), Atlanta, GA
Xu S, Zheng N, Liu J et al (2013) Geochemistry and health risk assessment of arsenic exposure to street dust in the zinc smelting district, Northeast China. Environ Geochem Health 35:89–99. https://doi.org/10.1007/s10653-012-9463-1
Zamanian Z, Sedaghat Z, Mehrifar Y (2018) Harmful outcome of occupational exposure to petrol: assessment of liver function and blood parameters among gas station workers in Kermanshah City. Iran Int J Prev Med 9:100. https://doi.org/10.4103/ijpvm.IJPVM_296_16
Zgłobicki W, Telecka M (2021) Heavy metals in urban street dust: health risk assessment (Lublin City, E Poland). Appl Sci 11:4092. https://doi.org/10.3390/app11094092
Zheng X, Zhao W, Yan X et al (2015) Pollution characteristics and health risk assessment of airborne heavy metals collected from beijing bus stations. Int J Environ Res Public Health 12:9658–9671. https://doi.org/10.3390/ijerph120809658
Funding
The authors declare that no funds, grants, or other support were received during the preparation of this manuscript.
Author information
Authors and Affiliations
Contributions
The experiments of this work were designed by Kamil Jurowski and Adrian Frydrych. The experiments were performed by Adrian Frydrych and Kamil Jurowski. Kamil Jurowski and Adrian Frydrych analyzed the data, wrote the manuscript, and edited the figures and table reviewed the manuscript. KJ: Writing—original draft, Writing—review & editing, Visualization; Conceptualization, Investigation, Data curation, Writing—original draft, Writing—review & editing, Visualization, Project administration. AF: Writing—original draft, Writing—review & editing, Visualization; Measurement; Data curation; Collecting samples.
Corresponding author
Ethics declarations
Competing Interest
The authors declared that no conflict of interest.
Ethical Approval
Not applicable.
Additional information
Publisher's Note
Springer Nature remains neutral with regard to jurisdictional claims in published maps and institutional affiliations.
Supplementary Information
Below is the link to the electronic supplementary material.
Rights and permissions
Open Access This article is licensed under a Creative Commons Attribution 4.0 International License, which permits use, sharing, adaptation, distribution and reproduction in any medium or format, as long as you give appropriate credit to the original author(s) and the source, provide a link to the Creative Commons licence, and indicate if changes were made. The images or other third party material in this article are included in the article's Creative Commons licence, unless indicated otherwise in a credit line to the material. If material is not included in the article's Creative Commons licence and your intended use is not permitted by statutory regulation or exceeds the permitted use, you will need to obtain permission directly from the copyright holder. To view a copy of this licence, visit http://creativecommons.org/licenses/by/4.0/.
About this article
Cite this article
Frydrych, A., Jurowski, K. Hazardous Elements in Road Dust from Petrol Stations in Rzeszów (Podkarpackie, Poland): Toxicological Assessment Using Field Portable X-ray Fluorescence (FP-XRF) as ‘White Analytical Technique’. Expo Health (2024). https://doi.org/10.1007/s12403-024-00644-6
Received:
Revised:
Accepted:
Published:
DOI: https://doi.org/10.1007/s12403-024-00644-6