Abstract
Purpose
Giant leucaena is a tropical tree-legume that thrives in alkaline and calcareous soils. It contains large amounts of mimosine, the physiological role of which is not well understood. The goals of this study were to determine i) if mimosine is released by giant leucaena into the rhizosphere ii) if mimosine enhances solubility of Fe present in various forms in alkaline soil, and iii) if mimosine enhances transition metal uptake in giant leucaena.
Methods
Mimosine in the extracts of rhizosphere soil and root exudates was analyzed by high performance liquid chromatography (HPLC) and liquid chromatography/mass spectrometry (LC/MS) to determine if it is released by giant leucaena. Iron concentration in tissue and aqueous samples was determined by ICP-OES analysis.
Results
Mimosine was detected in both the rhizosphere eluates and root exudates. Mimosine added to samples of goethite, hematite and FeCl3 increased Fe solubility in water. Giant leucaena plants grown in alkaline soils and supplied with mimosine had higher levels of chlorophyll, Fe and Mn than the control plants.
Conclusion
Mimosine is released by giant leucaena roots into the rhizosphere, where it interacts with Fe. Mimosine enhances solubility of insoluble Fe-oxides and prevents conversion of ferric Fe to insoluble oxides at an alkaline pH. Mimosine enhances Fe and Mn uptake in plants growing in alkaline soil conditions.
Similar content being viewed by others
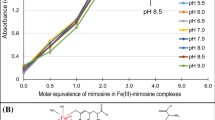
Avoid common mistakes on your manuscript.
Introduction
Approximately 30% of the world’s cultivated lands contain calcareous soils with high pH (Bashir et al. 2010). In aerobic and alkaline soils, Fe is sparingly soluble and as a consequence, plants growing under these conditions often exhibit symptoms of Fe deficiency (Marschner 1995; Ogo et al. 2011). Iron-deficient plants undergo leaf chlorosis, have stunted growth, and produce low yields. Iron is one of the most important soil micronutrients and is involved in many biological pathways such as photosynthesis, respiration and DNA synthesis (Rout and Sahoo 2015). Iron is required by plants at concentrations ranging from 10 nM to 1 mM; however, the amount of free ferric and ferrous Fe in soils is less than 10 fM, which is too low for optimal plant growth and development (Guerinot and Yi 1994; Kim and Guerinot 2007; Marschner 1995). On the other hand, Fe is one of the most abundant elements in the earth comprising roughly 5.6% of the earth’s crust (Morrissey and Guerinot 2009). Although it is highly abundant in the soil, Fe remains a limiting nutrient for plants due to poor its solubility and availability. In alkaline soils, ferrous ions are scarce and Fe is mostly present as insoluble ferric oxides such as goethite (FeOOH) and hematite (Fe2O3) (Wang et al. 2018). Both goethite and hematite are abundant in tropical environments (Journet et al. 2014).
To acquire Fe from the soil, plants utilize one of two strategies. Non-graminaceous plants utilize strategy I, while graminaceous plants utilize strategy II. In strategy I, plant roots secrete organic acids and natural chelates into the rhizosphere, where they bind to and help to solubilize soil Fe. Ferric reductase oxidase enzymes (FRO) also known as ferric chelate reductases, on the root surface, reduce the ferric-chelates formed in the rhizosphere to ferrous ions. Then, an Fe-regulated transporter (IRT) takes up the ferrous ions into the plants. In some strategy I-utilizing plants, protons are secreted by plant roots through H + pumps (AHA). This serves to acidify the soil and helps to solubilize ferric ions, which can then bind to chelating compounds such as organic acids, which are also secreted by plants. In strategy II of Fe uptake from soils, Fe-binding compounds called phytosiderophores are secreted by the transporter of mugineic acid (TOM) efflux protein. One of these phytosiderophores is 2′-deoxymugineic acid (DMA), which binds to ferric ions in soils to form a ferric-DMA complex that is taken up by the yellow stripe–like family of peptide transporters (YSL). Some plants like rice and maize, possess proteins for both strategies of Fe uptake from the soil (Li et al. 2018; Wairich et al. 2019).
The tropical tree-legume Leucaena leucocephala (leucaena) thrives in alkaline and calcareous soils (Brewbaker 2016). Honda and Borthakur (2019) suggested that leucaena may possess dual strategies for Fe uptake from the soil. A number of strategy I and II genes were found to be expressed at high levels in roots, leaves, and stems of giant leucaena treated under various Fe availability conditions (Honda and Borthakur 2020). In the same study, the authors suggested that mimosine plays an important role in both Fe-uptake strategies. Mimosine is a non-proteinogenic amino acid found in all parts of giant leucaena at various concentrations. Mimosine has been shown to bind various metallic cations at acidic, neutral and alkaline pHs (Honda and Borthakur 2020). Mimosine was also found to be a stress-response molecule that has various stress-alleviating roles and fluctuates in giant leucaena when exposed to different environmental stresses (Honda and Borthakur 2021). Mimosine shares structural similarities to other known phytochelators such as caffeic acid, maltol and L-DOPA. Like mimosine, these compounds have various roles during plant stress.
The objective of the present study was to study the role of mimosine as a phytosiderophore in giant leucaena. Mimosine is hypothesized to facilitate Fe-uptake in giant leucaena from alkaline soils by solubilizing Fe-oxides. This hypothesis was tested by conducting experiments to (i) verify that mimosine is released into the rhizosphere; (ii) determine if mimosine can leach from defoliated leaves, (iii) determine if mimosine enhances uptake of metallic cations in giant leucaena growing in alkaline soils; and (iv) quantify the Fe-solubilizing ability of mimosine at an alkaline pH.
Materials and methods
Germination and growth of giant leucaena plants
Mature seeds of giant leucaena variety K636 were collected from the Waimanalo Research Station, University of Hawaii, on the island of Oahu, HI. Mature seeds were scarified by immersing them in concentrated H2SO4 for 10 min with gentle agitation at room temperature. After scarification, seeds were rinsed several times with diH2O and germinated in 21’ × 10’ plastic trays containing a potting soil and vermiculite mix (50/50 v/v) (pH 5.8). Potting soil contained a heterogeneous mixture of organic and inorganic matter. Plants were watered weekly with ¼ strength Hoagland solution (pH 5.8). The seedlings were grown for one-month in a plant growth chamber at 25° C ± 2° C with a 16/8 h light/dark photoperiod with an irradiance of 100 μmol s−1 m−1, and an average humidity between 60–65%.
Collection and determination of mimosine in the rhizosphere soil surrounding giant leucaena roots
It was expected that if giant leucaena plants release mimosine into the soil, mimosine should be present in the soil surrounding its roots. Therefore, to determine the presence of mimosine in the soil, growth media containing a potting-soil-vermiculite mixture (50/50 v/v) (pH 5.8) and previously harboring giant leucaena plants were collected and pooled. Mimosine was eluted by rinsing the used soil several times with 0.1 N HCl. The ‘soil eluate’ was pooled and concentrated using a Labconco freeze dry system (Labconco, Kansas City MO, USA) and then stored at -80° C.
Mimosine in old dried plant tissues
It is possible that defoliated plant parts, both fresh or old, can leach mimosine from their tissues into the ground below. A lot of mimosine (2–5% DW) is present in fresh leucaena foliage; however, it is not known how much mimosine remains in old, dry defoliated leaves. Therefore, to determine if old and dried giant leucaena plant parts still contain mimosine that can be leached out, the leaves, stems and roots of old and dried giant leucaena (> 2 years old) were collected and further dried overnight at 65 °C. To extract mimosine, 1 g of dried tissue was submerged in 30 mL of 0.1 N HCl. The samples were incubated overnight at room temperature with shaking. After incubation, plant tissue extracts were analyzed for the presence of mimosine using HPLC. The amount of mimosine in the plant tissue was determined as a percentage of the plant dry weight. Each sample set contained at least three replications.
Mimosine release from fallen giant leucaena leaves
To determine if fresh and old dried leaves of giant leucaena can leach mimosine into the ground below, 5 g of fresh giant leucaena leaves were placed on the top of pots containing 1 kg DW of unused potting-soil-vermiculite mixture (50/50 v/v) (pH 5.8). In second experiment, 2 g of dried defoliated giant leucaena leaves were placed on the top of pots containing 1 kg DW of unused soil-vermiculite mixture. To mimic rainfall, 100 mL of diH2O was sprinkled on the surface of the growth media every day for one-week. The soil flow-through water was collected each day for each experimental set and then combined. The soil flow-through water experimental set was analyzed for the presence of mimosine. The amount of mimosine leached from leaves was calculated and determined as an amount (μg) of the growth media DW (g). Each sample set contained at least three replications.
Mimosine on plants growing directly below leucaena foliage
In addition to root exudate and leaching from defoliated leaves, it is possible that during periods of rain, mimosine could be washed from the surface of leucaena plant leaves to the area below. To test this, the foliage of Vitex rotundifolia that was growing directly below the foliage of giant leucaena, and the foliage of the same species, but growing away from giant leucaena foliage, were collected after a period of moderate rain. The leaf samples were rinsed several times in 0.1 N HCl and then the eluate analyzed for the presence of mimosine following the methods described above. Plants growing away from the leucaena foliage was used as the control. Each sample set contained at least four replications.
Collection of giant leucaena root exudates
To determine if mimosine is secreted in the giant leucaena root exudates, one-month old seedlings were transferred from potting-soil-vermiculite mixture (50/50 v/v) (pH 5.8) to hydroponic growth media containing ¼ strength Hoagland solution (pH 5.8) and grown for one-week to allow the plants to adjust to new media conditions. After one-week, the plants were transferred to fresh hydroponic media containing ¼ strength Hoagland solution (pH 5.8) and then grown for one week. Hydroponic media were collected and replaced daily. The collected media were pooled separately and then freeze dried using a Labconco freeze dry system. All samples were stored at—80° C until needed.
To determine if pH affects secretion of mimosine, one-month old giant leucaena seedlings were transferred from potting-soil-vermiculite mixture (50/50 v/v) (pH 5.8) to hydroponic media containing ¼ strength Hoagland solution (pH 5.8) and grown for one-week to allow plants to adjust to new media conditions. After one-week, the plants were transferred to fresh hydroponic media containing ¼ strength Hoagland solution and buffered to either pH 6.0, 7.0, or 8.0 using 0.05 M MES or 0.1 M Tris–HCl and then grown for one-week. Hydroponic media were collected and replaced daily. The collected media were pooled separately for each pH and then concentrated using a Labconco freeze dry system. All samples were stored at—80° C until needed. After one week, all the plants were dried and the amount of mimosine secreted determined as a percentage of the plant dry weight. Each sample set contained four biological replications.
To determine if nitrogen availability affects secretion of mimosine, one-month old leucaena seedlings were transferred from potting-soil-vermiculite mixture (50/50 v/v) (pH 5.8) to hydroponic growth media containing ¼ strength Hoagland solution (pH 5.8) and grown for one-week to let plants adjust to new media. After one-week, leucaena plants were transferred to fresh hydroponic media containing ¼ strength Hoagland solution (pH 5.8) but no nitrogen and supplemented with either 0, 0.2, 0.8, or 3.2 mM ammonium nitrate and then grown for two-weeks. Hydroponic media were collected and replaced daily. The collected media were pooled separately for each concentration of ammonium nitrate and then concentrated using a Labconco freeze dry system. All samples were stored at—80° C until needed. After one-week, all plants were dried and the amount of mimosine secreted determined as a percentage of the plant dry weight. Each sample set contained four biological replications.
High performance liquid chromatographic analyses of old dried giant leucaena tissue extracts, rhizosphere soil eluate, root exudates, and leaf wash of plants growing directly below giant leucaena foliage
All soil eluates and root exudates samples were diluted with diH2O and filtered to remove insoluble material. Mimosine in the samples was determined with a Waters 2695 high performance liquid chromatography (HPLC) system equipped with a photodiode array detector (Waters, Millford, MA, USA) and a Phenomenex C18 column (Phenomenex, Torrance, CA, USA). Mimosine was eluted with 0.02 M o-phosphoric acid aqueous solution at a constant flow rate of 1 mL/min and detected at A280 nm. A mimosine authentic standard (Millipore-Sigma, St. Louis, MO, USA) was used to identify mimosine and calibrate concentrations.
Liquid chromatography/mass spectrometry (LC/MS) analyses of rhizosphere soil eluate and root exudates
To confirm the presence of mimosine in the soil eluate, and in the root exudates, rhizosphere soil eluates and root exudates were collected, pooled and stored in the -80° C. Sample preparation methods can be found in the supp material (S1). Mimosine in the lyophilized samples was analyzed on an Agilent 6410 Triple Quadrupole LC/MS (Agilent Technologies, Santa Clara, CA, USA). The LC/MS was operated at electrospray ionization (20 eV) and the negative MRM mode to detect ions of mass to charge ratio (m/z) of 197.1 (M-H) and the product ion m/z 110.0 (hydroxy-pyridone ring of mimosine).
Mimosine supplied to leucaena plants growing in alkaline soils
Metal concentrations
To determine if mimosine supplied to plants enhances uptake of Fe, giant leucaena seedlings were grown in alkaline soil (pH 8.0–8.5) collected from the Waimanalo Research station. The Fe concentration of soil was previously determined to be ~ 1.98 ± 0.05 µg/g DW (extractable soil Fe). Plants were grown for one-month and supplied with either water only, water + 100 μM EDDHA, or water + 100 μM mimosine. All solutions were adjusted to pH 8.0–8.5 using NaOH. After one-month, whole plants (leaves, stems, and roots) were harvested, dried and ground into powder using mortar and pestle. Samples were sent to the College of Tropical Agriculture and Human Resources Agricultural Diagnostic Services Center (CTAHR ADSC) for determination of metal concentration in whole plant tissues by ICP-OES. Each sample set contained at least four biological replications.
Chlorophyll concentration
Giant leucaena seedlings were grown in alkaline soil for one-month and supplied with either water only, water + 100 μM EDDHA, or water + 100 μM mimosine. After one-month, 50 mg of fresh leaflets were collected from plants and then chlorophyll extracted in a non-destructive method using modified methods described by Richardson et al. (2002) and Siebeneichler et al. (2019). In this method, 50 mg of fresh leaves was submerged in 3 mL of dimethyl sulfoxide (DMSO) and heated at 65° C for 30 min. The DMSO extract was removed and transferred to a separate tube and stored at 4° C. This process was repeated until leaflets were transparent. The absorbances of DMSO extracts were assessed at 470, 645, and 663 nm using pure DMSO as the blank. Chlorophyll concentrations were quantified and determined as a % of plant fresh weight (FW) using the equations from Siebeneichler et al. (2019). Each sample set contained ten replications.
Iron solubilizing properties of mimosine
An amount of 50 mg of goethite or hematite was weighed and placed in 10 mL centrifuge tubes containing either diH2O (control), diH2O + 1 mM EDDHA, diH2O + 1 mM mimosine, diH2O + 2 mM mimosine, or diH2O + 3 mM mimosine. All samples were adjusted to pH 8.0 using 0.1 M Tris–HCl. Samples were then mixed for 1 h at room temperature and centrifuged at 5000 × g for 10 min. The soluble fractions were then collected and sent to the CTAHR ADSC for determination of Fe concentration by ICP-OES. Each sample set contained six replications.
Fe(III)-mimosine complexes were prepared at 1:0, 1:1, 1:2 and 1:3 molar ratios using FeCl3. Fe(III)-EDDHA was also prepared at 1:1 molar ratio. All samples were adjusted to pH 8.0 using 0.1 M Tris–HCl. Samples were then mixed for 1 h at room temperature and centrifuged at 16,000 × g for 5 min. The soluble fractions were collected and sent to the CTAHR ADSC for determination of Fe concentration by ICP-OES. Each sample set contained six replications.
Statistical analysis
An analysis of variance (ANOVA) test followed by a Tukey post-hoc test was used for data analysis.
Results
Mimosine in the rhizosphere soil and root exudates of giant leucaena
Mimosine in the rhizosphere soil eluates and giant leucaena root exudates was identified by comparison with the standard mimosine, at a retention time of 2.7 min (Fig. 1). The identities were confirmed by LC/MS MRM analyses (Figs. 2 and 3). A fragment at a m/z of 110.1, corresponded to the hydroxy-pyridone group of mimosine ([M] + -H, m/z = 197.1). The difference in the retention times of HPLC and MS/MRM data are due to the different types of solvent and column used and, the day to day differences in method development. These results indicate that giant leucaena roots release mimosine into the rhizosphere.
MS1 mass chromatogram of (a) commercial mimosine standard and MS2 multiple reactions monitoring (MRM) chromatograms of (b) fragmented commercial mimosine (standard). MS1 mass chromatogram of (c) HPLC purified mimosine in the giant leucaena root exudates and MS2 multiple reactions monitoring (MRM) chromatograms of (d) fragmented mimosine in the giant leucaena root exudate
MS1 mass chromatogram of (a) commercial mimosine standard and MS2 multiple reactions monitoring (MRM) chromatograms of (b) fragmented commercial mimosine (standard). MS1 mass chromatogram of (c) HPLC purified mimosine in the giant leucaena rhizosphere soil eluate and MS2 multiple reactions monitoring (MRM) chromatograms of (d) fragmented mimosine in the giant leucaena rhizosphere soil eluate
Mimosine in old dried plant tissues
The extracts of old dried leaves, stems and roots all had peaks at 2.7 min indicating mimosine was present in the giant leucaena tissues (Fig. 4a-c). The old dry leaves contained 0.16% DW mimosine. Roots had the lowest amount of mimosine relative to leaves, which had twofold more mimosine than the roots (Fig. 4d). These results suggest that mimosine remains stable inside old plant parts.
Mimosine release from fallen giant leucaena leaves
Both sets of soil eluates (old dried and fresh leaves) produced a peak at 2.7 min indicating that mimosine was leaching from leaves into the soil below (Fig. 5). The amount of mimosine that leached from old, dry and defoliated giant leucaena leaves were found to be 1.15 ± 0.22 μg/g of soil DW when fresh leaves were placed on the top of the soil and water applied, and 0.54 ± 0.38 μg/g soil DW when old dried leaves were used.
Mimosine secretion at pH 6.0, 7.0, and 8.0
The giant leucaena plants grown at pH 8.0 by far secreted the most mimosine, followed by those grown at pH 7.0 and 6.0 (Fig. 6). These results indicate that more mimosine was secreted in the root exudates when the plants were grown at an alkaline pH.
Mimosine secretion at different levels of combined nitrogen
Giant leucaena plants supplied with increasing amounts of ammonium nitrate secreted much more mimosine than those not supplied with nitrogen (Fig. 7). The results indicate a positive correlation between the amounts of mimosine secreted and nitrogen source provided to the plants.
Mimosine on plants growing directly below giant leucaena foliage
The leaf wash of plants not growing directly beneath leucaena plants served as the negative control and did not produce a peak at 2.7 min. The leaf wash of plants growing directly below leucaena foliage had peaks at 2.7 min indicating that mimosine was present on the leaf surface of these plants (Fig. 8). These results indicate that mimosine was transferred from giant leucaena foliage to the surface of leaves of plants growing understory.
Metal concentration of giant leucaena
Giant leucaena plants supplied with mimosine had higher Fe and Mn concentrations than the control plants (Fig. 9). Interestingly, giant leucaena plants supplied with mimosine also had lower concentrations of the heavy metal’s Cr and Cd. Not surprisingly, plants supplied with EDDHA also had higher Fe, Mn, Ni, Cu, Pb, and Co and concentrations than control plants. The results suggest that mimosine supplied to giant leucaena, enhances Fe uptake from alkaline soils.
Metal concentrations of giant leucaena whole plants that were grown in alkaline soil (pH 8.0–8.5) for one month and supplied weekly with either water (control), EDDHA, or mimosine. Error bars indicate standard error of four replicates. Bars sharing a letter of the same case do not differ by Tukey post-hoc test (P < 0.05)
Chlorophyll concentration of giant leucaena
Giant leucaena plants supplied with mimosine had higher total chlorophyll concentrations than plants supplied with just water (Fig. 10). Plants supplied with EDDHA had higher total chlorophyll concentrations than control plants. The results suggest that mimosine supplied to giant leucaena plants enhances chlorophyll synthesis.
Total chlorophyll concentration of giant leucaena plants grown in alkaline soil for one month and supplied with either water (control), EDDHA, or mimosine. Error bars indicate standard error of ten replicates. Bars sharing a number or a letter of the same case do not differ by Tukey post-hoc test (P < 0.05)
Mimosine and solubility of Fe oxides
Mimosine significantly enhanced extraction of Fe from goethite and hematite (Fig. 11a and b). Interestingly, mimosine bound to ferric ions at pH 8.0, was able to limit the conversion of ferric to insoluble ferric oxides (Fig. 11c). The results suggest that mimosine can extract and solubilize Fe from insoluble mineral oxides. These results also suggest that mimosine can prevent the conversion of ferric ions to insoluble ferric oxides.
Discussion
Phytosiderophores such as DMA and nicotianamine (NA), are known to be secreted in the root exudates of plants, and to be involved in the uptake of Fe from the soil and its transport within plants (Suzuki et al. 2006; Tsednee et al. 2014). Phytosiderophores increase the solubility of soil Fe through chelation and formation of Fe-phytosiderophore complexes (Kobayashi et al. 2014; Roberts et al. 2004). In a study conducted by Walter et al. (2017), it was found that Fe mobilization by DMA was increased by application of lime to soils. Oburger et al. (2014) found that DMA was secreted at significantly different rates by wheat plants growing in calcareous soils. DMA is known to be released under Fe deficiency conditions (Cakmak et al. 1994; Gries et al. 1995; Ma 2005). In the present study, it was found that root secretion of mimosine by giant leucaena was highest when plants were grown under alkaline conditions. When Fe is less soluble and sparingly available to plants, it was found that mimosine binds to Fe(III) best at alkaline pH 8.0 and 8.5 (Honda and Borthakur 2020). In the same study, it was shown that mimosine-Fe complexes are hydrophilic under alkaline conditions, and that mimosine was able to extract Fe directly from alkaline soils. Previously, Soedarjo and Borthakur (1998) observed that mimosine was released in the root exudates of leucaena plants. They also found that mimosine secretion and the mimosine concentration of leaves were higher in Rhizobium-inoculated plants. However, these results were not confirmed by LC/MS analysis. In the present study, LC/MS analyses confirmed the presence of mimosine in the giant leucaena root exudates and in the soils surrounding giant leucaena roots. It was also found that secretion of mimosine was higher when giant leucaena plants were supplied with increasing amounts of nitrogen. It is known that the mimosine concentration of giant leucaena tissues increases with higher availability of nitrogen (Honda and Borthakur 2021). Mimosine contains two nitrogen molecules, and therefore, it is not surprising that supplying plants with exogenous nitrogen sources, or inoculating plants with nitrogen-fixing Rhizobium increases both the mimosine concentration of plants and the secretion of mimosine in the root exudates.
In strategy I plants, phenolic compounds are known to be secreted in plant root exudates in response to Fe deficiency conditions (Jin et al. 2007). Specifically, secretion of phenolic coumarins have been shown to be increased from Arabidopsis thaliana roots in response to Fe deficiency at increased pH (Sisó-Terraza et al. 2016b). Secretion of phenolic coumarins have been shown to be important in tolerance of A. thaliana to Fe deficiency at elevated pH (Schmid et al. 2014; Schmidt et al. 2014). In the present study, secretion of mimosine in root exudates was found to be increased in giant leucaena plants that were grown at increasing pHs. Like other phenolic compounds, mimosine secretion is increased under Fe-limiting conditions, such as alkaline pH. In another study conducted by Sisó-Terraza et al. (2016a), it was found that flavins secreted by Beta vulgaris improved Fe nutrition. They also found that flavins enabled B. vulgaris to mine Fe from ferric-oxides through reduction. Honda and Borthakur (2020) found that Phaseolus coccineus supplied with various Fe-mimosine complexes had higher Fe concentrations than plants supplied with only Fe. They also found that plants supplied with Fe-mimosine complexes accumulated more mimosine than plants supplied with only mimosine, suggesting that Fe-mimosine complexes were taken up by P. coccineus plants. In the present study, giant leucaena plants growing in alkaline soil and supplied with mimosine had increased Fe concentrations. Similar to secreted flavins, mimosine also increased Fe mobilization in giant leucaena, which may also be due to Fe mining or through reduction of Fe-oxides. In the present study, mimosine was found to solubilize Fe from the insoluble Fe mineral oxides goethite and hematite at an alkaline pH. It was also found that mimosine limited the formation of insoluble Fe-oxides at pH 8.0. Enhancing the solubility of soil Fe by mimosine could explain why leucaena is well-adapted to alkaline soils.
In the present study, giant leucaena plants growing in alkaline soils and supplied with mimosine had higher Fe and Mn concentrations than control plants. The presence of mimosine facilitated Fe and Mn uptake, however, not Cu, Co or Zn. The ability of mimosine to extract and solubilize Fe from the alkaline soil is the likely reason for the increase in Fe concentrations in whole plant tissues. The increased Fe solubility leads to increased Fe uptake by giant leucaena plants. Mimosine can bind Fe in at least three different ways, and has been suggested to form polynuclear metal ligand complexes with Fe (Honda and Borthakur 2020). Citrate is also known to form polynuclear species with Fe (Silva et al. 2009). The formation of polynuclear metal–ligand species may increase the amount of Fe that is solubilized and made available to plants. Besides increasing the solubility and availability of soil Fe, it is possible that mimosine chelated to Fe may serve as a substrate for FRO enzymes that reduce Fe(III) to Fe(II). Iron-citrate and Fe-malate complexes have been shown to be substrates for leaf FRO enzymes (Gonzalez-Vallejo et al. 1999). Citrate is secreted in the root exudate of plants and secretion has been shown to be increased in plants under Fe deficiency (Tiffin 1966a, b). Citrate can bind Fe under physiological conditions (Silva et al. 2009). It is possible that mimosine, which is a bidentate ligand, could serve as a substrate for FRO when bound to Fe in its various forms.
Giant leucaena can release mimosine into the soil utilizing multiple mechanisms. Mimosine can be secreted in the root exudate, leach from fresh and old defoliated leaves and tissues, and can be washed from the leaf surface by rain. Honda and Borthakur (2019) found that dried leucaena leaves still contained mimosine. Similarly, the present study found that old dried leucaena plant parts still contained considerable amounts of mimosine, which could leach from these tissues into the soil. The various ways mimosine is released by giant leucaena into the ground would explain the presence of mimosine in the rhizosphere soil eluate. The mimosine released into the ground may serve to extract Fe from the soil and make it more abundant and available to plant roots. Defoliated tissues may provide a constant reservoir of mimosine that is released during rain. The ability of mimosine to extract and solubilize Fe at alkaline pH, its presence in the soil, and the multiple mechanisms of mimosine release into the soil, can explain the adaptability of giant leucaena to alkaline soils, where Fe is less abundant and available to plants.
Conclusion
Mimosine is released by giant leucaena into the rhizosphere soil through root exudates. Secretion of mimosine was the highest when giant leucaena was grown under alkaline conditions. Giant leucaena plants supplied with mimosine had higher Fe concentrations than the control plants. The increase in Fe concentration in giant leucaena was likely due to enhanced Fe solubility and uptake. Mimosine can solubilize Fe from two common forms of Fe in the soil, goethite and hematite, both of which being insoluble Fe oxides. Mimosine is also able to limit the formation of insoluble ferric-oxides under an alkaline pH. The ability of mimosine to enhance Fe solubility may be a major factor for giant leucaena’s tolerance to alkaline soils.
Data availability
Not applicable
Code availability
Not applicable
References
Bashir K, Ishimaru Y, Nishizawa NK (2010) Iron uptake and loading into rice grains. Rice 3:122–130. https://doi.org/10.1007/s12284-010-9042-y
Brewbaker JL (2016) Breeding leucaena: tropical multipurpose leguminous tree. Plant Breed Rev 40:43–121
Cakmak S, Gülüt KY, Marschner H, Graham RD (1994) Effect of zinc and iron deficiency on phytosiderophore release in wheat genotypes differing in zinc efficiency. J Plant Nutrit 17:1–17. https://doi.org/10.1080/01904169409364706
Gonzalez-Vallejo EB, Gonzalez-Reyes JA, Abadıa A, Lopez-Millan AF, Yunta F, Lucena JJ, Abadıa J (1999) Reduction of ferric chelates by leaf plasma membrane preparations from Fe-deficient and Fe-sufficient sugar beet. Aust J Plant Physiol 26:601–611. https://doi.org/10.1071/PP99002
Gries D, Brunn S, Crowley DE, Parker DR (1995) Phytosiderophore release in relation to micronutrient metal deficiencies in barley. Plant Soil 172:299–308. https://doi.org/10.1007/BF00011332
Guerinot ML, Yi Y (1994) Iron: nutritious, noxious, and not readily available. Plant Physiol 104:815–820. https://doi.org/10.1104/pp.104.3.815
Honda MDH, Borthakur D (2019) Mimosine concentration in Leucaena leucocephala under various environmental conditions. Trop Grasslands-Forrajes Tropicales 7:164–172. https://doi.org/10.17138/TGFT(7)164-172
Honda MDH, Borthakur D (2020) Mimosine facilitates metallic cation uptake by plants through formation of mimosine-cation complexes. Plant Mol Biol 102:431–445. https://doi.org/10.1007/s11103-019-00956-1
Honda MDH, Borthakur D (2021) Mimosine is a stress-response molecule that serves as both an antioxidant and osmolyte in giant leucaena (Leucaena leucocephala subsp. glabrata) during environmental stress conditions. Plant Stress 2:100015. https://doi.org/10.1016/j.stress.2021.100015
Jin CW, You GY, He YF, Tang CX, Wu P, Zheng SJ (2007) Iron deficiency induced secretion of phenolics facilitates the reutilization of root apoplastic iron in red clover. Plant Physiol 144:278–285. https://doi.org/10.1104/pp.107.095794
Journet E, Balkanski Y, Harrison SP (2014) A new data set of soil mineralogy for dust-cycle modeling. Atmos Chem Phys 14:3801–3816. https://doi.org/10.5194/acp-14-3801-2014
Kim SA, Guerinot ML (2007) Mining iron: uptake and transport in plants. FEBS Lett 2007:2273–2280. https://doi.org/10.1016/j.febslet.2007.04.043
Kobayashi T, Nakanishi Itai R, Nishizawa NK (2014) Iron deficiency responses in rice roots. Rice 7:27. https://doi.org/10.1186/s12284-014-0027-0
Li A, Zhou X, Chen J, Chen R (2018) Is there a strategy I iron uptake mechanism in maize? Plant Sig Behav 13:e1161877
Ma JF (2005) Plant root responses to three abundant soil minerals: silicon, aluminum and iron. Crit Rev Plant Sci 24:267–281. https://doi.org/10.1080/07352680500196017
Marschner H (1995) Mineral Nutrition of Higher Plants. Vol. 2nd ed. Academic Press, Boston
Morrissey J, Guerinot ML (2009) Iron uptake and transport in plants: the good, the bad, and the ionome. Chem Rev 109:4553–4567
Oburger E, Gruber B, Schindelegger Y, Schenkeveld WDC, Hann S, Kraemer SM, Wenzel WW, Puschenreiter M (2014) Root exudation of phytosiderophores from soil-grown wheat. New Phytol 203:1161–1174. https://doi.org/10.1111/nph.12868
Ogo Y, Itai RN, Kobayashi T, Aung MS, Nakanishi H, Nishizawa NK (2011) OsIRO2 is responsible for iron utilization in rice and improves growth and yield in calcareous soil. Plant Mol Biol 75:593–605
Richardson AD, Duigan SP, Berlyn GP (2002) An evaluation of noninvasive methods to estimate foliar chlorophyll content. New Phytol 153:185–194. https://doi.org/10.1046/j.0028-646X.2001.00289.x
Roberts LA, Pierson AJ, Panaviene Z, Walker EL (2004) Yellow stripe1. Expanded roles for the maize iron-phytosiderophore transporter. Plant Physiol 135:112–120. https://doi.org/10.1104/pp.103.037572
Rout GR, Sahoo S (2015) Role of iron in plant growth and metabolism. Rev Agric Sci 3:1–24. https://doi.org/10.7831/ras.3.1
Schmid NB, Giehl RFH, Döll S, Mock H-P, Strehmel N, Scheel D, Kong X, Hider RC, von Wirén N (2014) Feruloyl-CoA 6'-Hydroxylase1-dependent coumarins mediate iron acquisition from alkaline substrates in Arabidopsis. 164: 160–172. https://doi.org/10.1104/pp.113.228544
Schmidt H, Gunther C, Weber M, Sporlein C, Loscher S, Bottcher C, Schobert R, Clemens S (2014) Metabolome analysis of Arabidopsis thaliana roots identifies a key metabolic pathway for iron acquisition. PLoS One 9:e102444
Siebeneichler SC, Barbosa JS, Cruz AMM, Ramos MAD, Fernandes HE, Nascimento VL (2019) Comparison between extraction methods of photosynthetic pigments in Acacia mangium. Commun Plant Sci 9:1–5. https://doi.org/10.26814/cps2019001
Silva AMN, Kong XL, Parkin MC, Cammack R, Hider RC (2009) Iron(III) citrate speciation in aqueous solution. Dalton Trans 2009:8616–8625. https://doi.org/10.1039/b910970f
Sisó-Terraza P, Luis-Villarroya A, Fourcroy P, Briat JF, Abadía A, Gaymard F, Abadía J, Álvarez-Fernández A (2016a) Accumulation and secretion of coumarinolignans and other coumarins in Arabidopsis thaliana roots in response to iron deficiency at high pH. Front Plant Sci 7:1711. https://doi.org/10.3389/fpls.2016.01711
Sisó-Terraza P, Rios JJ, Abadía J, Abadía A, Álvarez-Fernández, (2016b) A Flavins secreted by roots of iron-deficient Beta vulgaris enable mining of ferric oxide via reductive mechanisms. New Phytol 209:733–745. https://doi.org/10.1111/nph.13633
Soedarjo M, Borthakur D (1998) Mimosine, a toxin produced by the tree-legume Leucaena provides a nodulation competition advantage to mimosine-degrading Rhizobium strains. Soil Biol Biochem 30:1605–1613. https://doi.org/10.1016/S0038-0717(97)00180-6
Suzuki M, Takahashi M, Tsukamoto T, Watanabe S, Matsuhashi S, Yazaki J, Kishimoto N, Kikuchi S, Nakanishi H, Mori S, Nishizawa N (2006) Biosynthesis and secretion of mugineic acid family phytosiderophores in zinc-deficient barley. Plant J 48:85–97. https://doi.org/10.1111/j.1365-313X.2006.02853.x
Tiffin LO (1966a) Iron translocation I: plant culture, exudate sampling, iron-citrate analysis. Plant Physiol 41:510–514. https://doi.org/10.1104/pp.41.3.510
Tiffin LO (1966b) Iron translocation. II. Citrate iron ratios in plant stem exudates. Plant Physiol 41:515–518. https://doi.org/10.1104/pp.41.3.515
Tsednee M, Yang SC, Lee DC, Yeh KC (2014) Root-secreted nicotianamine from Arabidopsis halleri facilitates zinc hypertolerance by regulating zinc bioavailability. Plant Physiol 166:839–852. https://doi.org/10.1104/pp.114.241224
Walter M, Kraemer SM, Schenkeveld WDC (2017) The effect of pH, electrolytes and temperature on the rhizosphere geochemistry of phytosiderophores. Plant Soil 418:5–23. https://doi.org/10.1007/s11104-017-3226-9
Wang L, Putnis CV, Hövelmann J, Putnis A (2018) Interfacial precipitation of phosphate on hematite and goethite. Minerals 8:207. https://doi.org/10.3390/min8050207
Wairich A, de Oliveira BHN, Arend EB, Duarte GL, Ponte LR, Sperotto RA, Ricachenevsky FK, Fett JP (2019) The combined strategy for iron uptake is not exclusive to domesticated rice (Oryza sativa). Sci Rep 9:1–7. https://doi.org/10.1038/s41598-019-52502-0
Funding
This project was supported by a McIntire-Stennis grant.
Author information
Authors and Affiliations
Contributions
Experiments for this manuscript were conducted by MDHH with discussions with DB. Diagrams, images, and tables were created by MDHH. The manuscript was written by MDHH in discussion with DB. The document was reviewed and edited by DB.
Corresponding author
Ethics declarations
The authors declare that no funds, grants, or other support were received during the preparation of this manuscript. There are no financial or non-financial interests of the authors that are directly or indirectly related to the work submitted for publication.
Ethics approval
Not applicable
Consent to participate
Not applicable
Consent for publication
Not applicable
Conflict of interest
The authors declare they have no conflict of interest.
Additional information
Responsible Editor: Jian Feng Ma.
Publisher’s note
Springer Nature remains neutral with regard to jurisdictional claims in published maps and institutional affiliations.
Supplementary Information
Below is the link to the electronic supplementary material.
Rights and permissions
Open Access This article is licensed under a Creative Commons Attribution 4.0 International License, which permits use, sharing, adaptation, distribution and reproduction in any medium or format, as long as you give appropriate credit to the original author(s) and the source, provide a link to the Creative Commons licence, and indicate if changes were made. The images or other third party material in this article are included in the article’s Creative Commons licence, unless indicated otherwise in a credit line to the material. If material is not included in the article’s Creative Commons licence and your intended use is not permitted by statutory regulation or exceeds the permitted use, you will need to obtain permission directly from the copyright holder. To view a copy of this licence, visit http://creativecommons.org/licenses/by/4.0/.
About this article
Cite this article
Honda, M.D.H., Borthakur, D. Mimosine facilitates Fe uptake by Leucaena leucocephala subsp. glabrata in alkaline soils by solubilizing Fe-oxides. Plant Soil 484, 279–292 (2023). https://doi.org/10.1007/s11104-022-05789-4
Received:
Accepted:
Published:
Issue Date:
DOI: https://doi.org/10.1007/s11104-022-05789-4