Abstract
Background and Aims
Barley breeding has increased yield over the last century, but the associated changes in the phenotype are largely unknown. Our aim was to quantify the rate of genetic gain in a collection of Australian barley cultivars representing seven decades of breeding, and the associated changes in the phenotype.
Methods
Thirteen barley cultivars were grown in the field at Roseworthy and Mintaro, South Australia, to evaluate shifts in phenology, yield, and its components. A subset of five cultivars was grown under controlled conditions to probe for changes in root traits and nutrient uptake.
Results
Grain yield increased at 16.0 ± 5.3 kg ha−1 yr−1 or 0.43 ± 0.15% yr−1 at Roseworthy, where average yield was 3.1 t ha−1. There was no relation between yield and year of registration at Mintaro, where severe, extended frost disrupted reproduction. Changes in phenology with year of registration were not apparent. The main drivers of yield gain were grain number per m2 and harvest index, with a minor contribution of shoot biomass. Root length density, specific root length, root extension rate, and nutrient uptake per cm of root length increased with year of registration.
Conclusions
The rate of genetic gain of Australian barley aligned with rates reported for other breeding programs worldwide and compared to 21.0 ± 2.3 kg ha−1 yr−1 for actual yield in Australian farms between 1961 and 2019. Changes in the growth and functionality of the root system highlight the indirect effects of selective pressure for yield and agronomic adaptation.
Similar content being viewed by others
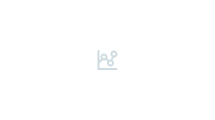
Avoid common mistakes on your manuscript.
Introduction
The first barley crop in Australia was 3 ha at Farm Cove, after the arrival of the First Fleet in 1788 (Sparrow and Doolette 1975), and currently spans 4.4 Mha, with an average yield of 2.9 t ha−1 (ABARES 2021), and a yield gap from 0.6 to 3.8 t ha−1 (https://yieldgapaustralia.com.au/). European cultivars were unsuitable to the dry and hot Australian environment, and the initial improvement of the crop was based on the British cultivar “Chevallier”, grown in the UK in the nineteenth century (Horne 1952; Sparrow and Doolette 1975). In 1903, a South Australian farmer developed the first local cultivar, Prior, from a selection of Chevallier. Prior was central to Australian barley production for more than 60 years. By 1954, Allan R. Callaghan highlighted the need for barley improvement, and lack of progress in comparison with wheat (Trumble 2001). As a result, the first breeding program was created in South Australia, releasing Clipper in 1968 (Friedt et al. 2011).
Plant breeding has increased the yield of barley at rates between 0.4% y−1 and 1.1% y−1 in Canada (Bulman et al. 1993), Italy (Martintello et al. 1987), Nordic countries (Ortiz et al. 2002; Peltonen-Sainio and Karjalainen 1991), Spain (Muñoz et al. 1998) and the US (Boukerrou and Rasmusson 1990; Wych and Rasmusson 1983). Higher yield associated with shorter stem, higher harvest index and more spikes and grains per unit area (Abeledo et al. 2003c). Studies on genetic gain in barley yield and associated changes in phenotype are lagging in Australia. In parallel to breeding advances, better agronomy and its interaction with breeding are required for yield progress.
Traits such as root length, rooting depth, and degree of root-soil contact are linked to water and nutrient uptake and adaptation to dry environments, and can contribute to improving nutrient use efficiency (Li et al., 2015; Palta and Turner, 2019; Tian et al., 2014). However, these traits involve trade-offs, and matching root phenotype to particular environments is not straightforward (Hoad et al. 2001; Kitomi et al. 2015; Manschadi et al. 2006; Manschadi et al. 2008; Schwinning and Ehleringer 2001; Tardieu 2012). Selective pressure for yield could indirectly select for root traits that putatively match the target environment. For example, selection for yield and agronomic adaptation of wheat in winter-rainfall environments of Australia over the last five decades reduced the size of the root system with no implications for water uptake, indicating root redundancy, and increased nitrogen uptake per unit root length that more than compensated for smaller root system (Aziz et al., 2017). The lack of synchrony between nutrient availability and crop growth contributes to the loss of mobile nutrients like nitrogen (Liao et al., 2004). Early and more extensive horizontal root growth in the 0.2–0.7-m soil profile contributed to superior nitrogen uptake in vigorous wheat lines (Liao et al., 2006). Developing thinner roots by stem elongation could improve the amount and rate of water and nutrient uptake in wheat (Palta and Nobel 1989). Robinson (2018) found no correlation between seminal root angle and grain yield in pot trials with 216 breeding lines from the Northern Region Barley Breeding program in Australia. Karunarathne et al. (2020) identified markers on barley chromosomes 1H(1), 3H(1), and 7H(2) associated with shoot length, relative shoot length, root dry weight and molecular mechanisms driving nitrogen use efficiency in barley. Candidate genes were identified including the high-affinity nitrate transporter 2.7 (HvNRT2.7), several transcriptional factors, protein kinases, and members of the asparagine synthetase gene family. However, the relevance of these findings is uncertain because plants were grown in a highly artificial hydroponic system.
The aims of this study were (i) to quantify the rate of genetic gain and related changes in phenology and yield components, and (ii) to probe for changes in root traits and nutrient uptake associated with selection for yield and agronomic adaptation in Australian barley representing seven decades of breeding.
Methods
A historic collection of 13 barley cultivars (Table 1) adapted to winter-rainfall environments of Australia was phenotyped for phenology, yield and its components at Roseworthy (-34.54, 138.688) and Mintaro (-33.884, 138.774), South Australia in 2018. A subset of the collection (five cultivars, plus Proctor) were phenotyped for root traits and nutrient uptake under controlled conditions (Table 1). With support from local breeders, the historic collection was set according to three criteria: a focus on two-rowed phenotypes, proven agronomic adaptation and widespread grower uptake.
Genetic gain in yield, shifts in phenology , and yield components
The soil was a calcareous loam (Calcarosol) at Roseworthy, and a medium-heavy clay (Vertosol) at Mintaro (Isbell 1996). Sowing date was 27 May 2018 at Roseworthy and 1 June 2018 at Mintaro. Plots were six rows, 10 m long, 0.26 m between rows, and a target plant density of 180 plants m−2. Adjacent plots were spaced at 0.7 m. Crops were rainfed, fertilised with 230 kg ha−1 of single super phosphate (8.8% P) banded 3–4 cm below the seed, and 140 kg ha−1 of urea (46% N) broadcasted at 2-leaves. Crops were monitored weekly to control weeds, pests and diseases. Experimental design was a randomised complete block design with three replicates.
Crops were monitored weekly to determine the time to heading (GS 5.5) and maturity (GS 9.0) using the phenological scale of Zadoks et al. (1974). At maturity, shoots were sampled in two central rows (0.52 m2). Samples were oven dried at 70 °C during 48 h, weighted and threshed for estimating the yield and its components: shoot biomass and harvest index, grain number and grain weight. Grain weight was estimated in subsamples of 300 grains. Protein in grain was measured with Micro-NIR-1700ES spectroscopy (VIAVI Solutions Inc, AZ, US).
Root phenotyping
Six cultivars (Table 1, plus the variety Proctor, year of registration 1952) were grown in 1-m deep, glass-walled rhizo-boxes (Supplementary Fig. 1) to map the growth and distribution of the root system with the method described by Liao et al. (2006) and Aziz et al. (2017). The soil was sourced from the top 3 cm of a sandy loam from Roseworthy (Supplementary Table 1); it was sterilised and packed to a bulk density of approximately 1.35 g cm−3. At sowing, the equivalent of 100 kg N ha−1 was incorporated (Osmocote ® All Purpose Controlled Release Fertiliser, NSW, Australia) and mixed into the 0.1-m topsoil. Four plants were grown in each rhizo-box. We used a randomised complete block design with four replicates per cultivar. Plants were grown in chambers at 23/14 °C day/night with a day length of 12 h. Water was applied to field capacity just before sowing, and 800 cm3 were applied every 10 days after the second visible node.
We mapped the growth of the root system using transparent film and drawing roots on the transparent wall of each rhizo-box to avoid repeated measurements. Roots were measured weekly from emergence until roots reached the bottom of the box to avoid artifacts (Passioura 2006). Transparent films were scanned with WinRhizo (Régent Instrument Inc., Quebec, Canada) to estimate root length. At harvest, shoots were counted, oven dried during 48 h at 70 °C, and grinded for nutrient analysis (Thomas Wiley® model 4, Swedesboro, NJ, US) and). Nutrients (N, P, K, S, Ca, Mg, Na, B, Cu, Mn, Fe, Al, and Mb) were measured with an ICP-OES (Inductively Coupled Plasma Optical Emission Spectrometer) Perkin Elmer Optima 7300 (PerkinElmer, Inc., MA, USA). The soil was divided into 5 sections: 0–21 cm; 21–42 cm; 42–63 cm, 63–84 cm and 84–100 cm; roots were washed, separated with a 2 mm mesh and dried to determine dry weight.
Statistical analysis
We used analysis of variance (ANOVA) to probe for the effects of cultivar, location and their interaction on phenology, yield and its components in the field experiment, and for the effect of cultivar on root and nutrient traits. We calculated the absolute (e.g., kg ha−1 yr−1) and relative (% yr−1) rate of genetic change of each trait as the slope of the least square regression between the trait and the year of registration of the cultivar. The relative rate was calculated in relation to the newest cultivar (Fischer et al. 2014). Following updated statistical recommendations we avoid the wording “statistically significant”, “non-significant”, or the variations thereof, thus avoiding dichotomisation based on an arbitrary discrete p-value (Wasserstein et al. 2019). Instead, we report p as a continuous quantity, and Shannon information transform [s = -log2(p)] as a measure of the information against the tested hypothesis (Greenland 2019). Although s is a function of p, the additional information provided is not redundant. With the base-2 log, the units for measuring this information are bits (binary digits). For example, the chance of seeing all heads in 4 tosses of a fair coin is 1/24 = 0.0625. Thus, p = 0.05 conveys only s =—log2(0.05) = 4.3 bits of information, “which is hardly more surprising than seeing all heads in 4 fair tosses” (Greenland 2019).
Results
Growing conditions
Crops grew under warmer and drier conditions at Roseworthy compared to Mintaro (Fig. 1). Minimum temperature during the first three weeks after sowing averaged 6.5 °C at Roseworthy and 3.8 °C at Mintaro. Mean temperature during the growing season, from May to December averaged 13.5 °C at Roseworthy and 11.8 °C at Mintaro, where 23 days recorded minima below 0 °C, particularly during grain set. Rainfall during the first three weeks after sowing was 29 mm at Roseworthy and 50 mm at Mintaro. Seasonal rainfall was 228 mm at Roseworthy and 324 mm at Mintaro.
Phenology
Time to heading varied with all three sources of variation, cultivar, location, and interaction (Supplementary Table 2). It ranged from 654 °C day for Sloop at Mintaro to 779 °C day for Flagship and Commander at Roseworthy (Table 1), and was not related to year of registration (p = 0.097, s = 3.4 at Roseworthy, p = 0.2371, s = 2.1 at Mintaro).
Grain yield and its components, and grain protein
Yield responded to all three sources of variation (Supplementary Table 2) and was unrelated to phenology (p = 0.32, s = 1.6). Grain yield increased at 16.0 ± 5.3 kg ha−1 yr−1 or 0.43 ± 0.15% yr−1 at Roseworthy (Fig. 2). This rate compares with 21.3 kg ha−1 yr−1 for Australian farms (Fig. 3a) and aligned with rates of genetic gain in other countries (Fig. 3b). Frost before heading (Fig. 1) affected the early ear cohorts at Mintaro, and yield measured in a later cohort did not associate with year of registration (Fig. 2a).
(a) Comparison of grain yield in a collection of historic barley cultivars grown at Roseworthy (this study), and actual yield in farmers’ fields. (b) Comparison of genetic yield gain of barley in Australia (this study) and breeding programs in other countries. The absolute rate of genetic gain is higher in higher-yielding environments, captured in a correlation with environmental mean yield, the average of all varieties in each study. Lines are least square regressions. Sources of data: (a) Farmers’ yield is from FAO (https://www.fao.org/faostat/en/), (b) Argentina (Abeledo et al. 2003a, b), Australia (this study), Brazil (Rodrigues et al. 2020), Canada (Bulman et al. 1993; Jedel and Helm 1994), Italy (Martintello et al. 1987), Spain (Muñoz et al. 1998), Turkey (Kaya and Akçura 2022), UK (Riggst et al. 1981), and US (Wych and Rasmusson 1983).
Grain number varied with all three sources of variation (Supplementary Table 2); it increased with the year of registration at 35 ± 15 grains m−2 yr−1 or 0.37 ± 0.17% yr−1 at Roseworthy, with no trend at Mintaro (Fig. 4a, Table 2). Grain weight varied with cultivar and location, with no interaction (Supplementary Table 2) and was unrelated to year of registration (Fig. 4b).
Harvest index and biomass both varied with cultivar and location, but not with interaction (Supplementary Table 2). Harvest index increased at 0.094 ± 0.03% yr−1 or 0.23% yr−1 at Roseworthy and at a lower rate at Mintaro (Fig. 4c, Supplementary Table 2). Shoot biomass increased with the year of registration at 28 ± 14 kg ha−1 yr−1 or 0.31 ± 0.17% yr−1 at Roseworthy, with no apparent trend at Mintaro.
Grain protein concentration varied with cultivar and did not vary with location or interaction (Supplementary Table 2). At Mintaro, grain protein concentration decreased with the year of registration at 0.05% yr−1; no trend was apparent at Roseworthy (Fig. 2b).
Root traits
Cultivars differed in several root traits (Supplementary Table 3, Fig. 5). Root length density increased with the year of cultivar registration between 1942 and 1980s, and levelled off afterward (Fig. 5a). Specific root length increased with the year of registration at a rate of 2.8 cm g−1 yr−1 or 0.47% yr−1 indicating thinner roots in modern cultivars (Fig. 5b). Shoot biomass did not associate with year of registration (Fig. 5c). There was a weak trend of increase of root biomass per tiller with the year of registration at 0.27 ± 0.14 mg root shoot−1 yr−1 or 0.33 ± 0.18% yr−1. The root extension rate increased at 0.25 cm d−1 yr−1 or 0.5% yr−1 (Fig. 5e). Time to reach 1-m depth was shortened at 2.3 ± 0.05 d every 10 years or 0.31% yr−1 (Fig. 5f).
(a) Root length density, (b) specific root length, (c) root biomass, (d) root biomass per shoot, (e) root extension rate, and (f) days to reach 1 m as a function of the year of registration of historical barley cultivars. Error bars are two standard errors of the mean. Lines are least squares regressions.
Uptake and concentration of nutrients
Nutrient uptake per plant, nutrient uptake per unit root length and concentration of nutrient in shoot varied with cultivar (Supplementary Table 4). Table 2 lists the genetic gains in absolute and relative terms calculated as the slopes of least square regression between trait and year of registration. Nutrient uptake decreased with year of registration for K, S, Ca, Mg and Na, and did not vary for the other nutrients. Nutrient uptake per unit root length decreased with the year of registration for N, K, S, Ca, Mg, Na, B, Cu, Mn and Mb, with no change for the other nutrients. Concentration of nutrients in shoot increased with year of registration for P and decreased for K, S, Ca, Mg and Na.
Discussion
Australian barley breeders select for yield, phenology, plant height, resistance to lodging, head loss caused by stem breakage, grain and feed quality, and resistance to disease (Friedt et al. 2011). Early breeding was based on limited backcrossing to incorporate resistance traits and with a scarce number of European parents that, during the 1970s and 1980s, benefited from the introgression of genes from African landraces that contributed to match phenology to the environment, and more recent introgression of genes from germplasm from US, Japan and Europe (Friedt et al. 2011). Subsoil chemical constraints such as boron toxicity are widespread in our environments, and barley breeders have selected for adaptation to these constraints, with consequences for root and nutrient traits as discussed below.
The rate of genetic yield between 1942 and 2013 was slightly smaller than the actual rate of yield gain in farms, suggesting a narrowing yield gap and the need to enhance genetic gains (Fig. 3a). Higher absolute rates were associated with higher yielding environments (Fig. 3b), as found for wheat, maize and rice (Liu et al. 2021). The relative rate of genetic gain in yield is usually independent of environmental yield potential (Fischer et al. 2014). The rate of genetic gain for yield in Australia aligned with those reported for other breeding programs worldwide (Fig. 3b), and could be used to benchmark estimates of genetic gain calculated with the breeder’s equation.
Genetic gain in yield associated with harvest index and grain number, in common with barley elsewhere (Abeledo et al. 2003b, c; Bulman et al. 1993; Jedel and Helm 1994; Kaya and Akçura 2022; Martintello et al. 1987; Muñoz et al. 1998; Peltonen-Sainio and Karjalainen 1991; Rajala et al. 2016; Riggst et al. 1981; Rodrigues et al. 2020; Wych and Rasmusson 1983), and in common with wheat (Calderini et al. 1999; Sadras and Lawson 2011; Slafer et al. 1990, 1994). Harvest index was below 50%. Improvements in the harvest index of barley associated with plant height (Austin et al. 1980; Bertholdsson and Kolodinska Brantestam 2009; Bingham et al. 2012; Bulman et al. 1993; Rajala et al. 2016). Future improvement in biomass partitioning to grain might be feasible against theoretical limits of 64% for wheat (Foulkes et al. 2011) and 60% in oats (Peltonen-Sainio 1991). The actual limit for barley is unknown. Early generation selection for harvest index in wheat successfully culled low yielding lines, and enriched populations with high yielding lines before testing the lines for yield at plot level (Cann et al. 2022). Barely breeding in South Australia and Western Australian has used backcrossing and marker assisted selection for disease resistance; New South Wales and Queensland have used mass selection for early-generation screening and double haploid lines were used in the to improve breeding efficiency.
Genetic gain in yield of barley in Argentina related to higher grain number, higher spike number per unit land, and higher number of spikelet primordia per main shoot, with no changes in harvest or plant height (Abeledo et al. (2003b). Breeding in Italy and North America also increased grain number and also associated to spike number per unit land (Martintello et al. 1987; Wych and Rasmusson 1983). The biomass improvement observed in our experiment at Roseworthy was in agreement with studies in Canada and Argentina (Abeledo et al. 2003b; Bulman et al. 1993).
We did not find shifts in phenology with the year of registration in our collection of cultivars, similar to a study with a Canadian barley sample from 1910 to 1988 (Bulman et al. (1993). Lack of association between days to anthesis and year of release in barley was reported for UK 1880–1980, US 1920–1984, and Spain 1937–1993 (Boukerrou and Rasmusson 1990; Muñoz et al. 1998; Wych and Rasmusson 1983). One exception is the longer time to anthesis in newer cultivars in a series from 1910 to 1987 in Canada (Jedel and Helm 1994). Australian breeders incorporated earliness into their germplasm through the Eam1 gene contained the earliest introduced European line (Prior), which contributed to adaption to hot springs (Friedt et al. 2011). Voss-Fels et al. (2018) suggested that selection for lines with the VERNALIZATION1 (VRN1-1) allele, which is common in Australian barley varieties and simultaneously induces early flowering and maintains “steep-cheap-deep” root systems, provides a double mechanism of adaptation to drought by modifying phenology with improved water or nutrient uptake. This was based on the association between VRN1-1 and narrow root growth in young plants and prolonged root growth at 60–80 cm depth after flowering.
High concentration of boron is widespread in local soils (Brennan and Adcock 2004; Cartwright et al. 1986; Sadras et al. 2002). Nable (1988) suggested the B exclusion could be adaptive in barley. In our, experiment B amount per cm or root decreased with the year of release. Germplasm and major QTL for boron tolerance have been identified in barley (Cartwright et al. 1987, 1986; Jefferies et al. 1999; Nable 1988; Sutton et al. 2007). Besides improving boron tolerance, the QTL on chromosome 4H associate with root length, and QTL on 4HL associate with aluminium/acid tolerance (Raman et al. 2002, 2003). The gene Mel1 was mapped on chromosome 4HS related to Mn uptake efficiency and the assessment of 95 Australian parental lines suggested that two Restriction Fragment Length Polymorphism (RFLP) markers could be used for its improvement. Australian breeders developed cultivars adapted to aluminium, manganese and boron toxicity (Jefferies et al. 1999). For instance, Sloop and Gairdner were developed with backcrosses and molecular markers for tolerance to both boron toxicity and root disease (Barr et al. 1998; McDonald et al. 2010). We also found a lower total uptake of K, Ca, Mg, Na, Al and Mn per plant in newer varieties. The combined selective pressure for yield and adaptation to local soils has delivered substantial shifts in root and nutrient-related traits (Fig. 5, Table 3). Furthermore, new barley varieties had thinner roots than older ones, with putative advantages for uptake of water and nutrients. Nitrogen uptake per cm root length decreased in modern cultivars compared to old ones, with no differences in total N uptake per plant or in root biomass. Thinner roots were associated with advantages in environments with scarce resources (Barraclough et al. 1989; Brunel-Saldias et al. 2020; Corneo et al. 2016; Melino et al. 2015) but only when they are not redundant (Passioura 1983). Thinner roots were also observed in the modern Australian wheat in the 20–40 cm layer of the soil profile (Aziz et al. 2017). Smaller root xylem vessels are associated with thinner roots, delaying water uptake (Condon et al. 2004; Palta et al. 2011; Richards and Passioura 1989; Vadez 2014).
Our method proved to be reliable to detect shifts in root traits in a historic collection of wheat (Aziz et al. 2017), but results have to be interpreted carefully due to both a small cultivar sample and artefacts from growing plants in containers in controlled conditions (Passioura 2006; Rich et al. 2020). Confirming this research with field studies that involve the assessment of the association between grain yield, nutrient uptake, root traits and water use would contribute to understand the breeding impact on drought adaptation. Newer varieties of barley have higher root length density and a faster rate of root vertical development than older varieties. This is in contrast to the selection for yield in wheat, which favoured smaller root systems (Aziz et al. 2017).
Conclusion
Selection for yield and agronomic adaptation in Australian barley over seven decades returned rates of yield improvement that aligned with expectations for these low-yielding conditions. We found some expected shifts in crop traits associated selection for yield, such as the increase in harvest index, and overlooked shifts in root and nutrient-related traits. Selection for yield in environments with widespread subsoil chemical constraints, and direct selection for adaptation to these constraints returned higher specific root length and faster deepening of the root system; these findings need validation in field experiments.
References
ABARES (2021) State data underpinning. Australian Bureau of Agricultural and Resource Economics and Sciences, Canberra
Abeledo L, Calderini D, Slafer G (2003a) Genetic improvement of yield responsiveness to nitrogen fertilization and its physiological determinants in barley. Euphytica 133(3):291–298
Abeledo LG, Calderini DF, Slafer GA (2003b) Genetic improvement of barley yield potential and its physiological determinants in Argentina (1944–1998). Euphytica 130:325–334
Abeledo LG, C. DF, Slafer GA (2003c) Physiological changes associated with genetic improvement of grain yield in barley. In: Slafer GA, Molina-Cano JL, Savin R, Araus JL, Romagosa I (Editors), Barley Science: Recent Advances from Molecular Biology to Agronomy of Yield and Quality. Food Product Press, New York, pp. 361–386
Austin RB et al (1980) Genetic improvements in winter wheat yields since 1900 and associated physiological changes. J Agric Sci 94(3):675–689
Aziz MM, Palta JA, Siddique KHH, Sadras VO (2017) Five decades of selection for yield reduced root length density and increased nitrogen uptake per unit root length in Australian wheat varieties. Plant Soil 413:181–192
Barr AR et al (1998) RFLP mapping of a new cereal cyst nematode resistance locus in barley. Plant Breed 117(2):185–187
Barraclough PB, Kuhlmann H, Weir AH (1989) The Effects of Prolonged Drought and Nitrogen Fertilizer on Root and Shoot Growth and Water Uptake by Winter Wheat. J Agron Crop Sci 163(5):352–360
Bertholdsson NO, KolodinskaBrantestam A (2009) A century of Nordic barley breeding–Effects on early vigour root and shoot growth, straw length, harvest index and grain weight. Eur J Agron 30(4):266–274
Bingham IJ, Karley AJ, White PJ, Thomas WTB, Russell JR (2012) Analysis of improvements in nitrogen use efficiency associated with 75 years of spring barley breeding. Eur J Agron 42:49–58
Boukerrou L, Rasmusson DD (1990) Breeding for High Biomass Yield in Spring Barley. Crop Sci 30(1):cropsci1990.0011183X003000010007x
Brennan RF, Adcock KG (2004) Incidence of Boron Toxicity in Spring Barley in Southwestern Australia. J Plant Nutr 27(3):411–425
Brunel-Saldias N, Ferrio JP, Elazab A, Orellana M, del Pozo A (2020) Root Architecture and Functional Traits of Spring Wheat Under Contrasting Water Regimes. Front Plant Sci 11. https://doi.org/10.3389/fpls.2020.581140
Bulman P, Mather DE, Smith DL (1993) Genetic improvement of spring barley cultivars grown in eastern Canada from 1910 to 1988. Euphytica 71:35–48
Calderini DF, Reynolds MP, Slafer GA (1999) Genetic wheat yield and main physiological changes associated with them during the 20th century. In: Satorre EH, Slafer GA (eds) Wheat: Ecology and Physiology of Yield Determination. Food Product Press, New York, pp 351–377
Cann DJ, Hunt JR, Rattey A, Harris FAJ, Porker KD (2022) Indirect early generation selection for yield in winter wheat. Field Crop Res 282:108505
Cartwright B, Rathjen AJ, Sparrow DHB, Paull JG, Zarcinas BA (1987) Boron tolerance in Australian varieties of wheat and barley. In: Gabelman WH, Loughman BC (Editors), Genetic Aspects of Plant Mineral Nutrition: Proceedings of the Second International Symposium on Genetic Aspects of Plant Mineral Nutrition, organized by the University of Wisconsin, Madison, June 16–20, 1985. Springer Netherlands, Dordrecht, pp. 139–151
Cartwright B, Zarcinas BA, Spouncer LR (1986) Boron toxicity in South Australian barley crops. Aust J Soil Res 37:351–359
Condon AG, Richards RA, Rebetzke GJ, Farquhar GD (2004) Breeding for high water-use efficiency. J Exp Bot 55(407):2447–2460
Corneo PE, Suenaga H, Kertesz MA, Dijkstra FA (2016) Effect of twenty four wheat genotypes on soil biochemical and microbial properties. Plant Soil 404(1):141–155
Fischer RA, Byerlee D, Edmeades GO (2014) Crop yields and global food security. Will yield increase continue to feed the world? ACIAR, Canberra
Fitzsimmons RW, Wrigley CW (1984) Australian Barleys: Identification of varieties, grain defects and foreign seeds. CSIRO, Melbourne, Australia
Foulkes MJ, et al. (2011) Raising yield potential of wheat. III. Optimizing partitioning to grain while maintaining lodging resistance. J Exp Bot 62(2):469–486
Friedt W, Horsley RD, Harvey BL, Poulsen DME, Lance RCM, Ceccarelli S, Grando S, Capettini F (2011) Barley breeding history, progress, objectives, and technology. Editor:Ullrich, S.E. Book Title:Barley: Production, Improvement, and Uses Publisher:Blackwell Publishing Ltd. pp. 637
Greenland S (2019) Valid P-Values Behave Exactly as They Should: Some Misleading Criticisms of P-Values and Their Resolution With S-Values. Am Stat 73(sup1):106–114
Hoad SP, Russell G, Lucas ME, Bingham IJ (2001) The management of wheat, barley, and oat root systems. Adv Agron 74:193–246. https://doi.org/10.1016/S0065-2113(01)74034-5
Horne FR (1952) Development of new varieties of malting barley. J Inst Brew 58:185–188
Isbell RF (1996) The Australian soil classification. The Australian soil classification.
Jedel PE, Helm JH (1994) Assessment of Western Canadian Barleys of Historical Interest: I. Yield and Agronomic Traits. Crop Sci 34(4):cropsci1994.0011183X003400040015x
Jefferies SP, et al. (1999) Mapping of chromosome regions conferring boron toxicity tolerance in barley (Hordeum vulgare L.). Theor Appl Genet 98(8):1293–1303
Karunarathne SD, Han Y, Zhang XQ, Zhou G, Hill CB, Chen K, Tefera A, Chengdao L (2020) Genome-wide association study and identification of candidate genes for nitrogen use efficiency in barley (Hordeum vulgare L.). Front Plant Sci 11. https://doi.org/10.3389/fpls.2020.571912
Kaya Y, Akçura M (2022) Impact of Climate Change on Some Barley Varieties with Different Growth Habit. Turk J Agric Nat Sci 9(1):107–118
Kitomi Y et al (2015) QTLs underlying natural variation of root growth angle among rice cultivars with the same functional allele of DEEPER ROOTING 1. Rice 8(1):16
Li P et al (2015) A genetic relationship between nitrogen use efficiency and seedling root traits in maize as revealed by QTL analysis. J Exp Bot 66(11):3175–3188
Liao M, Ian RP, Fillery IRP, Palta JA (2004) Early vigorous growth is a major factor influencing nitrogen uptake in wheat. Funct Plant Biol 31:121–129
Liao M, Palta JA, Fillery IRP (2006) Root characteristics of vigorous wheat improve early nitrogen uptake. Aust J Agric Res 57(10):1097–1107
Liu L, Sadras VO, Xu J, Hu C, Yang X, Zhang S (2021) Chapter Five - Genetic improvement of crop yield, grain protein and nitrogen use efficiency of wheat, rice and maize in China, Editor(s): Donald L. Sparks, Advances in Agronomy, Academic Press, Volume 168:203–252. https://doi.org/10.1016/bs.agron.2021.02.005
Manschadi AM, Christopher J, Devoil P, Hammer GL (2006) The role of root architectural traits in adaptation of wheat to water-limited environments. Funct Plant Biol 33(9):823–837
Manschadi AM, Hammer GL, Christopher JT, deVoil P (2008) Genotypic variation in seedling root architectural traits and implications for drought adaptation in wheat (Triticum aestivum L.). Plant Soil 303(1–2):115–129
Martintello P, Delocu G, Boggini G, Odoardi M, Stanca AM (1987) Breeding Progress in Grain Yield and Selected Agronomic Characters of Winter Barley (Hordeum vulgare L.) over the Last Quarter of a Century. Plant Breed 99(4):289–294
McDonald GK, Eglinton JK, Barr AR (2010) Assessment of the agronomic value of QTL on chromosomes 2H and 4H linked to tolerance to boron toxicity in barley (Hordeum vulgare L.). Plant Soil 326(1):275–290
Melino VJ, Fiene G, Enju A, Cai J, Buchner P, Heuer S (2015) Genetic diversity for root plasticity and nitrogen uptake in wheat seedlings. Funct Plant Biol 42:942–956. https://doi.org/10.1071/FP15041
Menz I (2010) Australian Barley Varieties: A reference guide. Barley Australia Ltd, NSW, Australia, 100 pp
Muñoz P, Voltas J, Araus JL, Igartua E, Romagosa I (1998) Changes over time in the adaptation of barley releases in north-eastern Spain. Plant Breed 117:531–535
Nable RO (1988) Resistance to boron toxicity amongst several barley and wheat cultivars: A preliminary examination of the resistance mechanism. Plant Soil 112(1):45–52
Ortiz R, Nurminiemi M, Madsen S, Rognli OA, Bjørnstad Å (2002) Genetic gains in Nordic spring barley breeding over sixty years. Euphytica 126(2):283–289
Palta J, Nobel PS (1989) Root respiration for Agave deserti: Influence of temperature, water status and root age on daily patterns. J Exp Bot 40:181–186
Palta JA et al (2011) Large root systems: Are they useful in adapting wheat to dry environments? Funct Plant Biol 38(5):347–354
Palta JA, Turner NC (2019) Crop root system traits cannot be seen as a silver bullet delivering drought resistance. Plant Soil 439(1):31–43
Passioura JB (1983) Roots and drought resistance. Agric Water Manag 7(1–3):265–280
Passioura JB (2006) The perils of pot experiments. Funct Plant Biol 33(12):1075–1079
Peltonen-Sainio P (1991) Productive oat ideotype for northern growing conditions. Euphytica 54(1):27–32
Peltonen-Sainio P, Karjalainen R (1991) Genetic Yield Improvement of Cereal Varieties in Northern Agriculture since 1920. Acta Agric Scand 41(3):267–273
Rajala A, Peltonen-Sainio P, Jalli M, Jauhiainen L, Hannukkala A, Tenhola-Roininen T, Ramsay L, Manninen O (2017) One century of Nordic barley breeding: nitrogen use efficiency, agronomic traits and genetic diversity. J Agric Sci 155:582–598. https://doi.org/10.1017/S002185961600068X
Raman H, Moroni JS, Sato K, Read BJ, Scott BJ (2002) Identification of AFLP and microsatellite markers linked with an aluminium tolerance gene in barley ( Hordeum vulgare L.). Theor Appl Genet 105:458–464
Raman H et al (2003) Mapping of genomic regions associated with net form of net blotch resistance in barley. Aust J Agric Res 54:1359–1367
Rich SM, Christopher J, Richards R, Watt M (2020) Root phenotypes of young wheat plants grown in controlled environments show inconsistent correlation with mature root traits in the field. J Exp Bot 71(16):4751–4762
Richards RA, Passioura JB (1989) A breeding program to reduce the diameter of the major xylem vessel in the seminal roots of wheat and its effect on grain yield in rain-fed environments. Aust J Agric Res 40(5):943–950
Riggst TJ et al (1981) Comparison of spring barley varieties grown in England and Wales between 1880 and 1980. J Agric Sci 97(3):599–610
Robinson H, Kelly A, Fox G, Franckowiak J, Borrell A, Hickey L (2018) Root architectural traits and yield: exploring the relationship in barley breeding trials. Euphytica 214:151. https://doi.org/10.1007/s10681-018-2219-y
Rodrigues O, Minella E, Costenaro E (2020) Genetic Improvement of Barley (Hordeum vulgare, L.) in Brazil: Yield Increase and Associated Traits. Agric Sci 11:425–438
Sadras V, Roget D, O’Leary G (2002) On-farm assessment of environmental and management constraints to wheat yield and efficiency in the use of rainfall in the Mallee. Aust J Agric Res 53(5):587–598
Sadras VO, Lawson C (2011) Genetic gain in yield and associated changes in phenotype, trait plasticity and competitive ability of South Australian wheat varieties released between 1958 and 2007. Crop Pasture Sci 62(7):533–549
Schwinning S, Ehleringer JR (2001) Water use trade-offs and optimal adaptations to pulse-driven arid ecosystems. J Ecol 89(3):464–480
Slafer GA, Andrade FH, Satorre EH (1990) Genetic-improvement effects on pre-anthesis physiological attributes related to wheat grain-yield. Field Crop Res 23(3–4):255–263
Slafer GA, Satorre EH, Andrade FH (1994) Increases in grain yield in bread wheat from breeding and associated physiological changes. In: Genetic Improvement of Field Crops. 488 pages. Editor: Slafer G.A. Pages 1–68. CRC Press. https://doi.org/10.1201/9781003210238
Sparrow DHB, Doolette JB (1975) Barley. In: A. Lazenby and E.M. Matheson (Editors), Australian Field Crops: Volume 1. Wheat and other temperate cereals. Angus & Robertson Publishers, Australia
Sutton T et al (2007) Boron-Toxicity Tolerance in Barley Arising from Efflux Transporter Amplification. Science 318(5855):1446–1449
Tardieu F (2012) Any trait or trait-related allele can confer drought tolerance: just design the right drought scenario. J Exp Bot 63(1):25–31
Tian H, Smet ID, Ding Z (2014) Shaping a root system: regulating lateral versus primary root growth. Trends Plant Sci 19(7):426–431
Trumble HPC (2001) The early history of the barley improvement Program, History of Agriculture in SA. PIRSA, pp. 14. https://pir.sa.gov.au/__data/assets/pdf_file/0006/331359/Trumble_paper_final.pdf. Accessed 20 June 2022
Vadez V (2014) Root hydraulics: The forgotten side of roots in drought adaptation. Field Crop Res 165:15–24
Voss-Fels KP et al (2018) VERNALIZATION1 Modulates Root System Architecture in Wheat and Barley. Mol Plant 11(1):226–229
Wasserstein RL, Schirm AL, Lazar NA (2019) Moving to a World Beyond “p < 0.05”. Am Stat 73(sup1):1–19
Wych RD, Rasmusson DC (1983) Genetic Improvement in Malting Barley Cultivars Since 1920. Crop Science, 23 (6):1037–1040. https://doi.org/10.2135/cropsci1983.0011183X002300060004x
Zadoks JC, Chang TT, Konzak CF (1974) A decimal code for the growth stages of cereals. Weed Res 14(6):415–421
Acknowledgements
We thank the Yitpi Foundation and GRDC-SARDI bilateral (project DAS00166) for the research funding; The Faulkner family for farm facilities; Nigel Wilhelm and his team for setting and maintaining the field trials; Barley quality lab, The University of Adelaide, for analytical facilities; Z. H. Chow, J. Fernández-López, T. Lenz, A. O’dea, and H. Tura for field and lab work.
Funding
Open Access funding enabled and organized by CAUL and its Member Institutions
Author information
Authors and Affiliations
Corresponding author
Ethics declarations
Competing Interest
The authors declare no conflict of interest.
Additional information
Responsible Editor: Martin Weih.
Publisher's Note
Springer Nature remains neutral with regard to jurisdictional claims in published maps and institutional affiliations.
Supplementary Information
Below is the link to the electronic supplementary material.
Rights and permissions
Open Access This article is licensed under a Creative Commons Attribution 4.0 International License, which permits use, sharing, adaptation, distribution and reproduction in any medium or format, as long as you give appropriate credit to the original author(s) and the source, provide a link to the Creative Commons licence, and indicate if changes were made. The images or other third party material in this article are included in the article's Creative Commons licence, unless indicated otherwise in a credit line to the material. If material is not included in the article's Creative Commons licence and your intended use is not permitted by statutory regulation or exceeds the permitted use, you will need to obtain permission directly from the copyright holder. To view a copy of this licence, visit http://creativecommons.org/licenses/by/4.0/.
About this article
Cite this article
Cossani, C.M., Palta, J. & Sadras, V.O. Genetic yield gain between 1942 and 2013 and associated changes in phenology, yield components and root traits of Australian barley. Plant Soil 480, 151–163 (2022). https://doi.org/10.1007/s11104-022-05570-7
Received:
Accepted:
Published:
Issue Date:
DOI: https://doi.org/10.1007/s11104-022-05570-7