Abstract
Paulownia witches’ broom (PaWB) caused by the phytoplasma is a devastating disease of Paulownia trees. It has caused heavy yield losses to Paulownia production worldwide. However, knowledge of the transcriptional and post-transcriptional regulation of gene expression by microRNAs (miRNAs), especially miRNAs responsive to PaWB disease stress, is still rudimentary. In this study, to identify miRNAs and their transcript targets that are responsive to PaWB disease stress, six sequencing libraries were constructed from healthy (PF), PaWB-infected (PFI), and PaWB-infected, 20 mg L−1 methyl methane sulfonate-treated (PFI20) P. fortunei seedlings. As a result, 95 conserved miRNAs belonging to 18 miRNA families, as well as 122 potential novel miRNAs, were identified. Most of them were found to be a response to PaWB disease-induced stress, and the expression levels of these miRNAs were validated by quantitative real-time PCR analysis. The study simultaneously identified 109 target genes from the P. fortunei for 14 conserved miRNA families and 24 novel miRNAs by degradome sequencing. Furthermore, the functions of the miRNA targets were annotated based on Gene Ontology and Kyoto Encyclopedia of Genes and Genomes pathway analysis. The results presented here provide the groundwork for further analysis of miRNAs and target genes responsive to the PaWB disease stress, and could be also useful for addressing new questions to better understand the mechanisms of plant infection by phytoplasma in the future.





Similar content being viewed by others
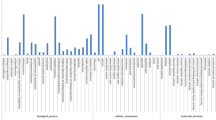
References
Addo-Quaye C, Eshoo TW, Bartel DP, Axtell MJ (2008) Endogenous siRNA and miRNA targets identified by sequencing of the Arabidopsis degradome. Curr Biol 18:758–762
Baric S, Kerschbamer C, Dalla Via J (2006) TaqMan real-time PCR versus four conventional PCR assays for detection of apple proliferation phytoplasma. Plant Mol Biol Rep 24:169–184
Bartel DP (2004) MicroRNAs: genomics, biogenesis, mechanism, and function. Cell 116:281–297
Berg JM, Shi Y (1996) The galvanization of biology: a growing appreciation for the roles of zinc. Science 271:1081–1085
Brodersen P, Voinnet O (2006) The diversity of RNA silencing pathways in plants. Trends Genet 22:268–280
Buhtz A, Springer F, Chappell L, Baulcombe DC, Kehr J (2008) Identification and characterization of small RNAs from the phloem of Brassica napus. Plant J 53:739–749
Cao X, Fan G, Zhao Z, Deng M, Dong Y (2014) Morphological changes of Paulownia seedlings infected phytoplasmas reveal the genes associated with witches’ broom through AFLP and MSAP. PLoS One 9:e112533
Capra M, Nuciforo PG, Confalonieri S, Quarto M, Bianchi M, Nebuloni M, Boldorini R, Pallotti F, Viale G, Gishizky ML, Draetta GF, Di Fiore PP (2006) Frequent alterations in the expression of serine/threonine kinases in human cancers. Cancer Res 66:8147–8154
Chi X, Yang Q, Chen X, Wang J, Pan L, Chen M, Yang Z, He Y, Liang X, Yu S (2011) Identification and characterization of microRNAs from peanut (Arachis hypogaea L.) by high-throughput sequencing. PLoS One 6:e27530
Chuck G, Candela H, Hake S (2009) Big impacts by small RNAs in plant development. Curr Opin Plant Biol 12:81–86
Curley A (1993) Paulownia growing rapidly outside Asia. J Forest 91:41
Ehya F, Monavarfeshani A, Mohseni Fard E, Karimi Farsad L, Khayam Nekouei M, Mardi M, Salekdeh GH (2013) Phytoplasma-responsive microRNAs modulate hormonal, nutritional, and stress signalling pathways in mexican lime trees. PLoS One 8:e66372
Fan G, Zhai X, Niu S, Ren Y (2014) Dynamic expression of novel and conserved microRNAs and their targets in diploid and tetraploid of Paulownia tomentosa. Biochimie 102:68–77
Filipowicz W, Jaskiewicz L, Kolb F, Pillai R (2005) Post-transcriptional gene silencing by siRNAs and miRNAs. Curr Opin Struct Biol 15:331–341
Fornari M, Calvenzani V, Masiero S, Tonelli C, Petroni K (2013) The Arabidopsis NF-YA3 and NF-YA8 genes are functionally redundant and are required in early embryogenesis. PLoS One 8:e82043
Gai YP, Li YQ, Guo FY, Yuan CZ, Mo YY, Zhang HL, Wang H, Ji XL (2014) Analysis of phytoplasma-responsive sRNAs provide insight into the pathogenic mechanisms of mulberry yellow dwarf disease. Sci Rep 4:5378
German MA, Pillay M, Jeong DH, Hetawal A, Luo S, Janardhanan P, Kannan V, Rymarquis LA, Nobuta K, German R, De Paoli E, Lu C, Schroth G, Meyers BC, Green PJ (2008) Global identification of microRNA-target RNA pairs by parallel analysis of RNA ends. Nat Biotechnol 26:941–946
Group T, Firrao G (2004) “Candidatus Phytoplasma”, a taxon for the wall-less, nonhelical prokaryotes that colonize plant phloem and insects. Int J Syst Evol Microbiol 54:1243–1255
Hafner M, Landgraf P, Ludwig J, Rice A, Ojo T, Lin C, Holoch D, Lim C, Tuschl T (2008) Identification of microRNAs and other small regulatory RNAs using cDNA library sequencing. Methods 44:3–12
Hao DC, Yang L, Xiao PG, Liu M (2012) Identification of Taxus microRNAs and their targets with high-throughput sequencing and degradome analysis. Physiol Plantarum 146:388–403
He F, Wang C, Wang F, Yang L (2001) Interaction mechanism between plant resistance gene ang pathogen avirulence gene. Chin J Cell Biol 33:1037–1044
Hiruki C (1999) Paulownia witches’-broom disease important in East Asia. Acta Horticul 469:63–68
Hofacker IL, Fontana W, Stadler PF, Bonhoeffer LS, Tacker M, Schuster P (1994) Fast folding and comparison of RNA secondary structures. Monatsh Chem 125:167–188
Lee I, Hammond RW, Davis RE, Gundersen DE (1993) Universal amplification and analysis of pathogen 16S rDNA for classification and identification of mycoplasmalike organisms. Phytopathology 83:834–842
Lelandais-Briere C, Naya L, Sallet E, Calenge F, Frugier F, Hartmann C, Gouzy J, Crespi M (2009) Genome-wide Medicago truncatula small RNA analysis revealed novel microRNAs and isoforms differentially regulated in roots and nodules. Plant Cell 21:2780–2796
Li J, Jia D, Chen X (2001) HUA1, a regulator of stamen and carpel identities in Arabidopsis, codes for a nuclear RNA binding protein. Plant Cell 13:2269–2281
Li B, Qin Y, Duan H, Yin W, Xia X (2011) Genome-wide characterization of new and drought stress responsive microRNAs in Populus euphratica. J Exp Bot 62:3765–3779
Liu R, Dong Y, Fan G, Zhao Z, Deng M, Cao X, Niu S (2013) Discovery of genes related to witches broom disease in Paulownia tomentosa × Paulownia fortunei by a De Novo assembled transcriptome. PLoS One 8:e80238
Livak KJ, Schmittgen TD (2001) Analysis of relative gene expression data using real-time quantitative PCR and the 2(-Delta Delta C(T)). Methods 25:402–408
Lu S, Sun Y, Shi R, Clark C, Li L, Chiang V (2005) Novel and mechanical stress–responsive microRNAs in Populus trichocarpa that are absent from Arabidopsis. Plant Cell Environ 17:2186–2203
Mackowiak SD (2011) Identification of Novel and Known miRNAs in deep-sequencing data with miRDeep2. Curr Protoc Bioinform 12(10):11–15
Mao W, Li Z, Xia X, Li Y, Yu J (2012) A combined approach of high-throughput sequencing and degradome analysis reveals tissue specific expression of microRNAs and their targets in cucumber. PLoS One 7:e33040
Meyers BC, Axtell MJ, Bartel B, Bartel DP, Baulcombe D, Bowman JL, Cao X, Carrington JC, Chen X, Green PJ, Griffiths-Jones S, Jacobsen SE, Mallory AC, Martienssen RA, Poethig RS, Qi Y, Vaucheret H, Voinnet O, Watanabe Y, Weigel D, Zhu JK (2008) Criteria for annotation of plant MicroRNAs. Plant Cell 20:3186–3190
Morita-Yamamuro C, Tsutsui T, Sato M, Yoshioka H, Tamaoki M, Ogawa D, Matsuura H, Yoshihara T, Ikeda A, Uyeda I, Yamaguchi J (2005) The Arabidopsis gene CAD1 controls programmed cell death in the plant immune system and encodes a protein containing a MACPF domain. Plant Cell Physiol 46:902–912
Mou H, Lu J, Zhu S, Lin C, Tian G, Xu X, Zhao W (2013) Transcriptomic analysis of paulownia infected by paulownia witches’-broom phytoplasma. PLoS One 8:e77217
Nagpal P, Ellis CM, Weber H, Ploense SE, Barkawi LS, Guilfoyle TJ, Hagen G, Alonso JM, Cohen JD, Farmer EE, Ecker JR, Reed JW (2005) Auxin response factors ARF6 and ARF8 promote jasmonic acid production and flower maturation. Development 132:4107–4118
Nakamura H, Yoshikawa N, Takahashi T, Sahashi N, Kubono T, Shoji T (1996) Evaluation of primer pairs for the reliable diagnosis of Paulownia Witches’-Broom disease using a polymerase chain reaction. Plant Dis 80:302–305
Namba S (2002) Molecular biological studies on phytoplasmas. J Gen Plant Pathol 68:257–259
Niu S, Fan G, Xu E, Deng M, Zhao Z, Dong Y (2014a) Transcriptome/degradome-wide discovery of MicroRNAs and transcript targets in two Paulownia australis genotypes. PLoS One 9:e106736
Niu S, Fan G, Zhao Z, Deng M, Dong Y (2014b) High-throughput sequencing and degradome analysis reveal microRNA differential expression profiles and their targets in Paulownia fortunei. Plant Cell Tiss Org 119:457–468
Peng X, Zhao Y, Cao J, Zhang W, Jiang H, Li X, Ma Q, Zhu S, Cheng B (2012) CCCH-type zinc finger family in maize: genome-wide identification, classification and expression profiling under abscisic acid and drought treatments. PLoS One 7:e40120
Rhoades MW, Reinhart BJ, Lim LP, Burge CB, Bartel B, Bartel DP (2002) Prediction of plant microRNA targets. Cell 110:513–520
Sabatini S, Beis D, Wolkenfelt H, Murfett J, Guilfoyle T, Malamy J, Benfey P, Leyser O, Bechtold N, Weisbeek P, Scheres B (1999) An auxin-dependent distal organizer of pattern and polarity in the Arabidopsis root. Cell 99:463–472
Sánchez C, Vielba JM, Ferro E, Covelo G, Solé A, Abarca D, De Mier BS, Díaz-Sala C (2007) Two SCARECROW-LIKE genes are induced in response to exogenous auxin in rooting-competent cuttings of distantly related forest species. Tree Physiol 27:1459–1470
Shukla L, Chinnusamy V, Sunkar R (2008) The role of microRNAs and other endogenous small RNAs in plant stress responses. Biochim Biophys Acta 1779:743–748
Siefers N, Dang KK, Kumimoto RW, Bynum WE, Tayrose G, Holt BF 3rd (2009) Tissue-specific expression patterns of Arabidopsis NF-Y transcription factors suggest potential for extensive combinatorial complexity. Plant Physiol 149:625–641
Sunkar R, Zhu JK (2004) Novel and stress-regulated microRNAs and other small RNAs from Arabidopsis. Plant Cell 16:2001–2019
Sunkar R, Girke T, Jain PK, Zhu JK (2005) Cloning and characterization of microRNAs from rice. Plant Cell 17:1397–1411
Sunkar R, Chinnusamy V, Zhu J, Zhu J (2007) Small RNAs as big players in plant abiotic stress responses and nutrient deprivation. Trends Plant Sci 12:301–309
Unver T, Bakar M, Shearman RC, Budak H (2010) Genome-wide profiling and analysis of Festuca arundinacea miRNAs and transcriptomes in response to foliar glyphosate application. Mol Genet Genomic 283:397–413
Vaucheret H (2006) Post-transcriptional small RNA pathways in plants: mechanisms and regulations. Genes Dev 20:759–771
Wang D, Guo Y, Wu C, Yang G, Li Y, Zheng C (2008) Genome-wide analysis of CCCH zinc finger family in Arabidopsis and rice. BMC Genom 9:44
Wang T, Pan H, Wang J, Yang W, Cheng T, Zhang Q (2014a) Identification and profiling of novel and conserved microRNAs during the flower opening process in Prunus mume via deep sequencing. Mol Genet Genomic 289:169–183
Wang Y, Wang Z, Amyot L, Tian L, Xu Z, Gruber MY, Hannoufa A (2014b) Ectopic expression of miR156 represses nodulation and causes morphological and developmental changes in Lotus japonicus. Mol Genet Genomic 290:471–484
Wei M, Wei H, Wu M, Song M, Zhang J, Yu J, Fan S, Yu S (2013) Comparative expression profiling of miRNA during anther development in genetic male sterile and wild type cotton. BMC Plant Biol 13:66
Whitney LAS, Loreti E, Alpi A, Perata P (2011) Alcohol dehydrogenase and hydrogenase transcript fluctuations during a day–night cycle in Chlamydomonas reinhardtii: the role of anoxia. N Phytol 190:488–498
Wysocka-Diller JW, Helariutta Y, Fukaki H, Malamy JE, Benfey PN (2000) Molecular analysis of SCARECROW function reveals a radial patterning mechanism common to root and shoot. Development 127:595–603
Zhai X, Cao X, Fan G (2010) Growth of Paulownia witches’ broom seedlings treated with methylmethane sulphonate and SSR analysis. Sci Silv Sin 46:176–181
Zhang B, Pan X, Cobb G, Anderson T (2006) Plant microRNA: a small regulatory molecule with big impact. Dev Biol 289:3–16
Zhou Z, Zeng H, Liu Z, Yang Z (2012a) Genome-wide identification of Medicago truncatula microRNAs and their targets reveals their differential regulation by heavy metal. Plant Cell Environ 35:86–99
Zhou ZS, Song JB, Yang ZM (2012b) Genome-wide identification of Brassica napus microRNAs and their targets in response to cadmium. J Exp Bot 63:4597–4613
Acknowledgments
This study was funded by the National Natural Science Foundation of China (Grant Nos. 30271082, 30571496, U1204309), by the Outstanding Talents Project of Henan Province (Grant No. 122101110700), by the Transformation Project of the National Agricultural Scientific and Technological Achievement of China (Grant No. 2012GB2D000271), and by the Science and Technology Innovation Team Project of Zhengzhou City, China (Grant No. 121PCXTD515).
Author information
Authors and Affiliations
Corresponding author
Ethics declarations
Conflict of interest
All authors declare that they have no conflict of interest.
Ethical approval
This article does not contain any studies with human participants or animals performed by any of the authors.
Additional information
Communicated by S. Hohmann.
Electronic supplementary material
Below is the link to the electronic supplementary material.
Rights and permissions
About this article
Cite this article
Niu, S., Fan, G., Deng, M. et al. Discovery of microRNAs and transcript targets related to witches’ broom disease in Paulownia fortunei by high-throughput sequencing and degradome approach. Mol Genet Genomics 291, 181–191 (2016). https://doi.org/10.1007/s00438-015-1102-y
Received:
Accepted:
Published:
Issue Date:
DOI: https://doi.org/10.1007/s00438-015-1102-y