Abstract
In the Southern Ocean, zooplankton research has focused on krill and macro-zooplankton despite the high densities of micro- and meso-zooplankton. We investigated their community structure in relation to different sea ice conditions around Japan’s Syowa Station in Lützow-Holm Bay, in the summers of 2011 and 2012. Zooplankton samples were collected using vertical hauls (0–150 m), with a closing net of 100-μm mesh size. The results of cluster analysis showed that the communities in this region were separated into fast ice, pack ice, and open ocean fauna. The fast ice fauna had lower zooplankton abundance (393.8–958.9 inds. m−3) and was dominated by cyclopoid copepods of Oncaea spp. (54.9–74.8 %) and Oithona similis (6.6–19.9 %). Deep-water calanoid copepods were also found at the fast ice stations. Pack ice and open ocean fauna had higher zooplankton abundance (943.6–2,639.8 inds. m−3) and were characterized by a high density of foraminiferans in both years (6.6–61.9 %). Their test size distribution indicated that these organisms were possibly released from melting sea ice. The pteropod Limacina spp. was a major contributor to total abundance of zooplankton in the open ocean zone in 2012 (26.4 %). The physical and/or biological changes between 2 years may affect the abundance and distribution of the dominant zooplankton taxa such as cyclopoid copepods, foraminiferans, and pteropods. Information on the relationships between the different species associated with sea ice will help to infer the possible future impacts of climate change on the sea ice regions.
Similar content being viewed by others
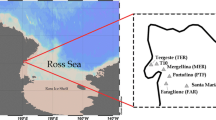
Avoid common mistakes on your manuscript.
Introduction
Zooplankton are secondary producers in marine ecosystems and play an important role as food for pelagic fish and air-breathing predators such as seals, whales, and penguins in the Southern Ocean (e.g. Hempel 1985a). While various factors defining zooplankton distribution in the Southern Ocean have been discussed, sea ice plays a crucial, highly dynamic, and variable role in the life cycles of organisms including zooplankton in the sea ice region (Massom and Stammerjohn 2010). In fact, Antarctic zooplankton communities are usually divided into three ice-associated faunal zones: (1) the northern ice-free zone, dominated by copepods and Salpa thompsoni; (2) the seasonal pack ice zone, dominated by Antarctic krill Euphausia superba; and (3) the fast ice zone, where the ice krill Euphausia crystallorophias is dominant (Hempel 1985b; Hosie 1994; Loeb 2007). However, these distributional data for macro-zooplankton are mostly based on information obtained from sampling with 200–500 μm nets or coarser nets such as the RMT (Rectangular Mid-water Trawl) 8. Recently, the increasing use of finer plankton nets (60–200 μm) has provided a more realistic view of the ecological significance of meso- (200–2,000 μm) and micro-zooplankton (20–200 μm), such as small calanoid and cyclopoid copepods, foraminiferans, and appendicularians (Metz 1996; Atkinson 1998; Schnack-Schiel et al. 1998; Atkinson and Sinclair 2000; Dubischar et al. 2002). Micro- and meso-zooplankton have been estimated to have a high abundance in the seasonal ice zone (Chiba et al. 2001; Swadling et al. 2010). Although their roles in the pelagic food web under sea ice are being gradually identified, the dynamics of these smaller zooplankton communities within the sea ice regions are still poorly understood.
Lützow-Holm Bay, where Japan’s Syowa Station is located, is a region where fast ice cover persists during summer, and some studies have been made of the zooplankton community structure around the shore-based stations of this bay (e.g. Fukuchi and Sasaki 1981; Fukuchi and Tanimura 1981; Fukuchi et al. 1985; Tanimura et al. 1986; Tanimura et al. 2008). The marine biological monitoring program of the sea ice region by ship-based sampling began with the 52nd Japanese Antarctic Research Expedition (JARE-52; 2010/2011 season). The aim of this program was to investigate biological production and mechanisms in relation to sea ice. The objectives of the present study were to examine the abundance and distribution patterns of micro- and meso-zooplankton communities in Lützow-Holm Bay during the austral summer, and their relationship to sea ice distribution. To achieve this objective, it was assumed that sea ice distribution would affect the distribution of micro- and meso-zooplankton communities in the sea ice regions as well as that of macro-zooplankton.
Materials and methods
Sampling
The survey was conducted during two cruises of the Japanese icebreaker Shirase through the sea ice zone in Lützow-Holm Bay. The first took place from February 9 to 24, 2011 (JARE-52) and the second from February 14 to March 4, 2012 (JARE-53; Fig. 1). Zooplankton samples were collected using a closing net (mouth diameter 0.75 m, mesh size 100 μm; Table 1) at nine stations (five in 2011 and four in 2012) with contrasting sea ice environments (Fig. 1): fast ice (four stations: 52A, 52B, 53A, and 53B), pack ice (three stations: 52C, 52D, and 53C), and ice-free open ocean (two stations: 52BP and 53BP). To prevent the sea ice from being mixed into the net, an ice fence was employed and the net was closed as it reached the surface (Fig. 2; Takahashi et al. 2012). The net was equipped with a flow meter to estimate the volume of water filtered and was vertically hauled from a depth of 150 m to the surface at stations where the bottom was deeper than 150 m, or from 5 m above the bottom to the surface at stations where the bottom was shallower than 150 m (Table 1). All samples were fixed immediately with buffered 5 % formaldehyde and seawater solution.
A number of environmental variables were also measured during the cruise. Temperature and salinity were measured at 1-m intervals down to the seafloor using a CTD (SBE 55 ECO, Sea-Bird Electronics, Bellevue, Washington, DC, USA). Water samples for measurement of chlorophyll a (Chl a) concentration were collected from three to seven depths in the upper 150 m with a Niskin bottle mounted on the CTD. Chl a concentration was determined fluorometrically with a Turner Designs fluorometer (model 10AU). For the purpose of comparison with zooplankton data, temperature and salinity data were averaged across the sampling layers. And Chl a concentrations were integrated vertically (e.g. Suzuki et al. 1998; Uitz et al. 2006).
Daily values of sea ice concentration were obtained from the National Snow and Ice Data Center (NSIDC) in Boulder, Colorado, USA. These data were derived from passive microwave data collected by the Special Sensor Microwave Imager (SSMI) (Cavalieri et al. 1996) and the Advanced Microwave Scanning Radiometer for EOS (AMSR-E) (Cavalieri et al. 2004).
Analysis
In the laboratory, zooplankton samples were split using a Motoda box splitter (Motoda 1959) so that approximately 1,000 individuals were counted per sample. The samples were identified to the lowest possible taxonomic level (generally species or genus) with a stereomicroscope. Members of the Ostracoda, Polychaeta, Salpa, Foraminifera, Chaetognatha, and Echinodermata were not identified to species level (Table 2). The copepods Oithona similis and Oncaea spp. were classed as adult or copepodite, with adult Oncaea spp. further identified to species level and classified as male or female. The maximum diameter of the test was measured for individual foraminiferans because the test size gives an indication of the growth stage. The test sizes of approximately 100 foraminiferans were measured per sample, except in cases where there were <100 individuals. Individual counts were converted to the number of individuals per 1 m3 for each station.
Abundance data were log-transformed (log10 [n + 1]) to decrease any bias in abundance, and a similarity matrix was constructed using the Bray–Curtis similarity index (Field et al. 1982). For grouping the samples, group average linkage was performed based on similarity. The SIMPER (similarity percentage) routine identified those species contributing to the similarity within the observed clusters. These statistical analyses were conducted using the PRIMER v6 software package (Clarke and Gorley 2006). The Shannon index (H′) was chosen to show the species diversity in each cluster group.
Results
Environmental variables
The average temperature and salinity ranged from −1.80 to 0.63 °C and 33.97 to 34.45 PSU, respectively (Table 1). A few stations contributed no data as the CTD did not work. Vertically integrated Chl a concentrations ranged from 1.08 to 80.13 mg m−2 (Table 1). Both temperature and salinity tended to increase from the fast ice stations to the open ocean stations each year, Chl a concentration showed a similar trend, with the exception of station 52A.
Community structure
A total of 42 zooplankton species/taxa were identified from the nine stations (Table 2). Zooplankton abundance ranged from 393.8 to 2,639.8 inds. m−3 (Fig. 3). Values were lower at the stations under fast ice and greater at those under pack ice or in the open ocean. Copepods (including nauplius stages) were generally the dominant zooplankton component, with the copepods O. similis and Oncaea spp. being the most abundant copepod taxa at all stations. For example, at the fast ice stations (52A, 52B, 53A, and 53B), Oncaea spp. accounted for 54.9–74.8 % of the total zooplankton abundance. Foraminiferans were also highly abundant at pack ice and open ocean stations, comprising 6.6–61.9 % of the total zooplankton community. Limacina spp. occurred in high numbers at pack ice and open ocean stations in 2012 (10.3 and 26.4 %, respectively). Copepod nauplii were also abundant, accounting for 4.3–21.8 % under pack ice and in the open ocean (Fig. 3).
Cluster analysis revealed three groups at ≥72.2 % similarity (Fig. 4). The groups were clearly separated by the interface between fast ice and pack ice. Group 1 comprised all four stations located under fast ice in 2011 and 2012. Groups 2 and 3 comprised the five stations located under pack ice or in the open ocean, Group 2 with the two stations in 2012 and Group 3 with the three stations in 2011.
The species contributing most to the similarity within Group 1 was Oncaea spp., followed by O. similis, Microcalanus pygmaeus, and Ctenocalanus citer (Table 3). Group 2 was characterized by O. similis, copepod nauplii, Oncaea spp., Limacina spp., and foraminiferans. Group 3 was mainly composed of foraminiferans, Oncaea spp., O. similis, copepod nauplii, and C. citer. The values for average abundance and species diversity were the highest in samples within Group 2, followed by Group 3 and then Group 1 (Table 3; Fig. 4). The average abundance of zooplankton in samples within Group 2 was more than triple that of samples within Group 1, while the species richness of samples within each group was almost the same (Table 3). The organisms contributing most to the difference between Groups 1 and 3 were foraminiferans, followed by copepod nauplii, Fritillaria spp., M. pygmaeus, O. similis, and echinoderms (Table 4). For Groups 1 and 2, Limacina spp. and foraminiferans were the most influential contributors to the dissimilarity. The species causing most of the dissimilarity between Groups 2 and 3 was Limacina spp., followed by Fritillaria spp., Calanoides acutus, Scolecithricella minor, copepod nauplii, and polychaetes (Table 4).
Population structure
The abundance of O. similis ranged from 47.9 to 491.4 inds. m−3 (Fig. 5a), and their pattern of abundance follows a similar pattern to that of total zooplankton abundance in this study (Fig. 3). Copepodite stages were dominant at all stations, accounting for 65.5–94.2 % of total abundance (Fig. 5a). The abundance of Oncaea spp. varied from 96.0 to 670.0 inds. m−3 (Fig. 5b). It was highest at pack ice station 52C in 2011 (472.0 inds. m−3), while in 2012, the highest value occurred at fast ice station 53A (670.0 inds. m−3). The species distribution and stage composition at each station were similar between 2011 and 2012: adults were dominant under fast ice, contributing 50.4–76.5 %, and adult abundance gradually decreased toward the open ocean. In contrast, copepodites were most abundant at the open ocean stations (79.4 % in 2011 and 83.7 % in 2012) and decreased in abundance toward the fast ice. Adult Oncaea antarctica occurred in abundance only at the northern pack ice and open ocean stations, while adult Oncaea curvata occurred at the southern pack ice and fast ice stations. Adult male O. curvata tended to be dominant in the fast ice area.
The test size distribution patterns for foraminiferans in 2011 and 2012 were similar (Fig. 6). At the open ocean stations, 52BP and 53BP, size ranges were 77.8–320.4 and 86.4–330.2 μm, respectively, and a peak in abundance occurred at approximately 175 μm. The peak at pack ice station 52D was the same as that found at the open ocean stations. However, at this station, the size range was 110–354.8 μm. The smaller individuals found at the open ocean stations were not present at station 52 D, and the largest sizes found were greater than those found at open ocean stations. At pack ice stations 52C and 53C, larger individuals were even more abundant in the samples (105.7–419.4 and 103.1–407.0 μm, respectively) and two peaks in size occurred, at approximately 200 and 300 μm (Fig. 6).
Discussion
Community structure of micro- and meso-zooplankton in sea ice regions
Information on micro- and meso-zooplankton community structure in sea ice regions, including those of the Southern Ocean, is sparse. The present study is one of few to try to assess variations in distribution and abundance of zooplankton under different sea ice conditions using ship-based sampling. This study indicates that micro- and meso-zooplankton community structures vary according to sea ice distribution. Two characteristic distribution patterns of zooplankton occurred in both years: (1) the ubiquitous distribution of O. similis and Oncaea spp.; and (2) the regional distribution of foraminiferans which contributed highly to the separate characterization of zooplankton communities in this sea ice region.
In addition, the pteropod Limacina spp. was the major contributor to total zooplankton abundance in the open ocean zone in 2012 (Fig. 3). Pteropods are ubiquitous components of Southern Ocean zooplankton communities and are extremely abundant regionally in the meso-zooplankton size fractions (Hunt et al. 2008). Regional and inter-annual variation in primary production is probably the major determinant of spatial and temporal variability in pteropod densities (Seibel and Dierssen 2003). In the present study, no relationship between pteropod density and Chl a concentration could be found. Thus, factors other than primary production may also play a role in determining the occurrence of pteropods in sea ice regions.
Mesh size effects on zooplankton sampling
The mesh size used for plankton sampling is a major factor affecting plankton selection and thus sample composition. In the Southern Ocean, several studies using plankton nets with 100-μm mesh size have indicated that small copepods exceed the abundance, and sometimes the biomass, of larger species (Metz 1996; Atkinson and Sinclair 2000; Dubischar et al. 2002; Schnack-Schiel et al. 2008). Makabe et al. (2012) noted that a 100-μm mesh net is suitable sampling gear to determine meso-zooplankton (200–2,000 μm) community structure in the northern region of Lützow-Holm Bay, although it was not appropriate for copepod nauplii. In their study, the average abundance of zooplankton was 2,664 ± 1,991 inds. m−3, and our data were within this range. In addition, Fukuchi and Tanimura (1981) observed a meso-zooplankton abundance of 2,495.7 ± 1,935.2 inds. m−3 at a fast ice station near St. 52A, a value also similar to our own for the fast ice stations. Therefore, the present data seem appropriate for the sea ice regions of the Southern Ocean.
Nonetheless, it is likely that the abundance of some taxa has been underestimated. Over 80 % of planktonic foraminiferans are smaller than 100 μm, and only adults are larger than 200 μm (Berger 1971; Brummer et al. 1986; Spindler and Dieckmann 1986). Similarly, for O. similis and Oncaea spp., it is difficult to assess the population structure for copepodite stages I(CI) and II (CII) or for copepod nauplius stages, as the body size of these juveniles is often <100 μm. Thus, the sampling regime used in the present study cannot fully represent micro-zooplankton and may underestimate the juvenile stages of meso-zooplankton. However, foraminiferans and copepods, including individuals smaller than 100 μm, were clearly abundant within the samples at pack ice and open ocean stations where Chl a concentrations were higher in both years (Table 3). Thus, despite their underestimate, a clear distribution pattern could be discerned.
The ubiquitous distribution of Oithona similis and Oncaea spp.
Oithona similis and Oncaea spp. are considered to be key components of the planktonic food web of the Southern Ocean (Atkinson 1998). They are the most numerically abundant copepod genera (Hunt and Hosie 2006a, b) and can form a significant proportion of the zooplankton biomass despite their small size (<1 mm). For example, they form up to 20 % of the total copepod biomass in the Weddell Sea (Schnack-Schiel et al. 1998). In the present study, they were the most abundant organisms in all cluster groups (Table 3).
Oithona spp. and Oncaea spp. are known to be omnivorous (Lampitt and Gamble 1982; Turner 1986). O. antarctica has been observed feeding on small copepods (Hopkins et al. 1993). O. similis are able to adapt to the low phytoplankton densities of the permanent open ocean zone (Takahashi et al. 2010). In the present study, Oncaea spp. occurred in high densities in the fast ice area where the Chl a concentration was the lowest. The omnivorous nature of these species may thus allow them to exploit areas of relatively low primary productivity, unavailable to purely herbivorous species. In addition to omnivory, these species have a behavioral strategy which may increase their intake of available microalgae. These copepods have been found to exhibit diel vertical migration synchronized with the sinking of microalgae from melting sea ice (Tanimura et al. 2008). The range of possible food sources and the feeding behavior of these copepods make them highly adaptable and thus lead to their ubiquitous distribution throughout the sea ice region.
Oncaea curvata is regarded as a deep-water species (Hardy and Gunther 1935; Seno et al. 1963). In ice-covered surface waters, Ainley et al. (1986) noted an abundance of crustacean species thought to occur only below 300 m. They suggested that the physical environment, in particular light intensity and quality, immediately beneath the ice was reminiscent of a mesopelagic environment. The pelagic environment in the fast ice area of our study would be similar to that of the deeper water where Oncaea spp. is generally found. The deep-water calanoid copepods, Aetideopsis antarctica and Spinocalanus sp. (Schnack-Schiel et al. 2008), were also found only at the fast ice stations in our study (Table 3).
The regional distribution of foraminiferans
Cluster analysis clearly separated groups according to the presence or absence of foraminiferans, which were in high abundance at the northern stations, in particular, the pack ice stations. Only one species of planktonic foraminiferans, Neogloboquadrina pachyderma Ehrenberg, lives in Antarctic waters, and the occurrence of this species in Lützow-Holm Bay was noted in the first JARE (Uchio 1960). Lipps and Krebs (1974) reported a high density of N. pachyderma in Antarctic floating ice, although it was not certain whether they were alive or merely preserved in the ice. Further studies by Spindler and Dieckmann (1986) revealed that only juveniles and sub-adults were found in high densities and that they were alive in the sea ice. Subsequently, Dieckmann et al. (1991) showed that foraminiferans in ice cores were mainly found in granular ice and that their numbers were much higher in the pack ice. Foraminiferans attach to frazil ice whose growth result in granular ice using their rhizopods and stay in the sea ice as part of their life cycle in the Southern Ocean (Eicken 1992). The ice community with its high density of foraminiferans is released into the water column as the sea ice melts. In the present study, the northern stations were areas where sea ice was melting or had recently melted. The high densities of foraminiferans found at these stations seem likely to reflect the release of foraminiferans from the ice.
Analysis of the size distribution of foraminiferans tests in sea ice cores has indicated that large living individuals (200–300 μm) are found in the lower part of the core (Spindler and Dieckmann 1986). Thus, it seems likely that in areas where the sea ice is in the process of melting the size of foraminiferans would be larger. Indeed, at the pack ice stations (52C and 53C), where the ice was just melting, a greater proportion of larger individuals was recorded. In addition, a trend in foraminiferans size distribution seemed apparent among stations with a greater proportion of smaller individuals at the open ocean stations (Fig. 6). If foraminiferans were released from melting sea ice, it might also be expected that their abundance would increase from the pack ice stations toward the open ocean stations. However, the densities at open ocean stations varied between years, and in 2012, the density at station 53BP was less than half of that at the pack ice station 53C. In addition, the numbers of larger individuals at open ocean stations were markedly less than those at the pack ice stations in both years. Adult specimens are mainly found below 200 m (Spindler and Dieckmann 1986); therefore, larger individuals may have sunk to deeper levels than the 150 m depth from which hauls were taken at the open ocean stations at the time of sampling. Another possibility is that the distribution of foraminiferans was related to Chl a concentration. The polar species N. pachyderma is known to be omnivorous but to exhibit a strong preference for phytoplankton (Hembleben et al. 1989; Lee and Anderson 1991). Bergami et al. (2009) and Takahashi et al. (2010) found correlations between foraminiferans abundance and Chl a concentration. In the present study, the Chl a concentration generally increased from fast ice to pack ice to open ocean stations. Thus, although melting ice and increased phytoplankton abundance may reflect the occurrence of foraminiferans at pack ice and open ocean stations compared with the fast ice stations, it does not explain variations between pack ice and open water stations. Foraminiferans did not show a clear distribution pattern in response to environmental conditions as identified by Hunt and Hosie (2006a) and Swadling et al. (2010).
The dynamics of sea ice around Antarctica is a major factor affecting the distribution and composition of zooplankton communities in these areas. This preliminary study indicates that, like macro-zooplankton, the distribution of micro- and meso-zooplankton varies between fast ice, pack ice and open ocean areas. While in general, O. similis and Oncaea spp. were ubiquitous, being able to adapt to fast ice, pack ice or open ocean, differences were observed in the distributions of copepodite stages compared with adults. Foraminiferans were only found in pack ice and open ocean stations. Foraminiferans have been found in the guts of higher trophic level organisms, such as polychaetes, fish, and tunicates (Hembleben et al. 1989), and they feed on phytoplankton, ciliates, and some copepods (Hembleben et al. 1989; Lee and Anderson 1991). The life cycle of these foraminiferans may be dependent on sea ice. Changes in sea ice dynamics and coverage because of climate change have major implications for the distribution of micro- and meso-zooplankton and for the ecology of the sea ice regions as a whole. Given this and the scarcity of studies of micro- and meso-zooplankton communities in sea ice regions, much more extensive and ongoing research is required in this field in the future.
References
Ainley DG, Fraser WR, Sullivan CW, Torres JJ, Hoplins TL, Smith WO (1986) Antarctic mesopelagic micronekton: evidence from seabirds that pack ice affects community structure. Science 232:847–849
Atkinson A (1998) Life cycle strategies of epipelagic copepods in the Southern Ocean. J Mar Syst 15:289–311
Atkinson A, Sinclair JD (2000) Zonal distribution and seasonal vertical migration of copepod assemblages in the Scotia Sea. Polar Biol 23:46–58
Bergami C, Capotondi L, Langone L, Giglio F, Ravaioli M (2009) Distribution of living planktonic foraminifera in the Ross Sea and the Pacific sector of the Southern Ocean (Antarctica). Mar Micropaleontol 73:37–48
Berger WH (1971) Sedimentation of planktonic foraminifera. Mar Geol 11:325–358
Brummer GJA, Hemleben C, Spindler M (1986) Planktonic foraminiferal ontogeny and new perspectives for micropaleontology. Nature 319:50–52
Cavalieri DJ, Parkinson CL, Gloersen P, Zwally HJ (1996) Sea ice concentrations from Nimbus-7 SMMR and DMSP SSM/I-SSMIS passive microwave data. Version 1.0. NSIDC, Colorado
Cavalieri DJ, Markus T, Comiso JC (2004) AMSR-E/aqua daily L3 12.5 km brightness, temperature, sea ice concentration, and snow depth polar grids V002. NSIDC, Colorado
Chiba S, Ishimaru T, Hosie GW, Fukuchi M (2001) Spatio-temporal variability of zooplankton community structure off east Antarctica (90 to 160°E). Mar Ecol Prog Ser 216:95–108
Clarke KR, Gorley RN (2006) PRIMER v6: user manual/tutorial. PRIMER-E, Plymouth
Dieckmann GS, Spindler M, Lange MA, Ackley SF, Eicken H (1991) Antarctic sea ice: a habitat for the foraminifer Neogloboquadrina pachyderma. J Foraminifer Res 21:182–189
Dubischar CD, Lopes RM, Bathmann UV (2002) High summer abundances of small pelagic copepods at the Antarctic polar front-implications for ecosystem dynamics. Deep-Sea Res II 49:3871–3887
Eicken H (1992) The role of sea ice in structuring Antarctic ecosystems. Polar Biol 12:3–13
Field JG, Clarke KR, Warwick RM (1982) A practical strategy for analyzing multispecies distribution patterns. Mar Ecol Prog Ser 8:37–52
Fukuchi M, Sasaki H (1981) Phytoplankton and zooplankton standing stocks and downward flux of particulate material around fast ice edge of Lützow-Holm Bay, Antarctica. Mem Natl Inst Polar Res Ser E (Biol Med Sci) 34:13–36
Fukuchi M, Tanimura A (1981) A preliminary note on the occurrence of copepods under sea ice near Syowa Station, Antarctica. Mem Natl Inst Polar Res Ser E (Biol Med Sci) 34:37–43
Fukuchi M, Tanimura A, Ohtsuka H (1985) Zooplankton community conditions under sea ice near Syowa Station, Antarctica. Bull Mar Sci 37:518–528
Hardy C, Gunther ER (1935) The plankton of the South Georgia whaling grounds and adjacent waters, 1926–1927. University Press, Cambridge
Hembleben C, Spindler M, Anderson OR (1989) Modern planktonic foraminifera. Springer, New York
Hempel G (1985a) Antarctic marine food web. In: Siegfried WR, Condy PR, Laws RM (eds) Antarctic nutrient cycles and food webs. Springer, Berlin, pp 266–270
Hempel G (1985b) On the biology of polar seas, particularly the Southern Ocean. In: Gray JS, Christiansen ME (eds) Marine biology of polar regions and effects of stress on marine organisms. Willey, Chichester, pp 3–34
Hopkins TL, Lancraft TM, Torres JJ, Donnelly J (1993) Community structure and trophic ecology of zooplankton in the Scotia Sea marginal ice zone in winter (1988). Deep-Sea Res I 40:81–105
Hosie GW (1994) The macrozooplankton communities in the Prydz Bay region, Antarctica. In: El-Sayed SZ (ed) Southern ocean ecology: the BIOMASS perspective. University Press, Cambridge, pp 93–123
Hunt BPV, Hosie GW (2006a) The seasonal succession of zooplankton in the Southern Ocean south of Australia, part I: the seasonal ice zone. Deep-Sea Res I 53:1182–1202
Hunt BPV, Hosie GW (2006b) The seasonal succession of zooplankton in the Southern Ocean south of Australia, part II: the sub-Antarctic to polar frontal zones. Deep-Sea Res I 53:1203–1223
Hunt BPV, Pakhomov EA, Hosie GW, Siegel V, Ward P, Bernard K (2008) Pteropods in Southern Ocean ecosystems. Prog Oceanogr 78:193–221
Lampitt RS, Gamble JC (1982) Diet and respiration of the small planktonic marine copepod Oithona nana. Mar Biol 66:185–190
Lee JJ, Anderson OR (1991) Biology of foraminifera. Academic Press, New York
Lipps JH, Krebs WN (1974) Planktonic foraminifera associated with Antarctic sea ice. J Foraminifer Res 4:80–85
Loeb V (2007) Environmental variability and the Antarctic marine ecosystem. In: Vasseur DA, McCann KS (eds) The impact of environmental variability on ecological systems. The Peter Yodzis fundamental ecology series, vol 2, pp 197–225
Makabe R, Tanimura A, Fukuchi M (2012) Comparison of mesh size effects on mesozooplankton collection efficiency in the Southern Ocean. J Plankton Res 34:432–436
Massom RA, Stammerjohn SE (2010) Antarctic sea ice change and variability—physical ecological implications. Polar Sci 4:149–186
Metz C (1996) Lebensstrategien dominanter antarktischer Oithonidae (Cyclopoida, Copepoda) und Oncaeidae (Poecilostomatoida, Copepoda) im Bellingshausenmeer. Ber Polarforsch 207:1–123
Motoda S (1959) Devices of simple plankton apparatus. Mem Fac Fish Hokkaido Univ 7:73–94
Schnack-Schiel SB, Hagen W, Mizdalski E (1998) Seasonal carbon dynamics of Antarctic copepods in the eastern Weddell Sea. J Mar Syst 17:305–311
Schnack-Schiel SB, Michels J, Mizdalski E, Schodlok MP, Schröder M (2008) Composition and community structure of zooplankton in the sea ice-covered western Weddell Sea in spring 2004—with emphasis on calanoid copepods. Deep-Sea Res II 55:1040–1055
Seibel BA, Dierssen HM (2003) Cascading trophic impacts of reduced biomass in the Ross Sea Antarctica: just the tip of the iceberg? Biol Bull 205:93–97
Seno J, Komaki Y, Takeda A (1963) Reports on the biology of the “Umitaka-Maru” expedition. Plankton collected by the “Umitaka-Maru” in the Antarctic and adjacent waters, with special references to copepod. J Tokyo Univ Fish 49:53–62
Spindler M, Dieckmann GS (1986) Distribution and abundance of the planktic foraminifer Neogloboquadrina pachyderma in sea ice of the Weddell Sea (Antarctica). Polar Biol 5:185–191
Suzuki K, Kishino M, Sasaoka K, Saitoh S, Saino T (1998) Chlorophyll-specific absorption coefficients and pigments of phytoplankton off Sanriku, Northwestern North Pacific. J Oceanogr 54:517–526
Swadling KM, Kawaguchi S, Hosie GW (2010) Antarctic mesozooplankton community structure during BROKE-west (30°E–80°E), January–February 2006. Deep-Sea Res II 57:887–904
Takahashi KT, Hosie GW, Kitchener JA, McLeod DJ, Odate T, Fukuchi M (2010) Comparison of zooplankton distribution patterns between four seasons in the Indian Ocean sector of the Southern Ocean. Polar Sci 4:317–331
Takahashi KT, Iida T, Hashida G, Odate T (2012) Field test of “ice-fence” for oceanographic observation in the sea-ice zone. Antarct Rec (in press)
Tanimura A, Fukuchi M, Hoshiai T (1986) Seasonal change in the abundance of zooplankton and species composition of copepods in the ice-covered sea near Syowa Station, Antarctica. Mem Natl Inst Polar Res Spec Issue 40:212–220
Tanimura A, Hattori H, Miyamoto Y, Hoshiai T, Fukuchi M (2008) Diel changes in vertical distribution of Oithona similis (Cyclopoida) and Oncaea curvata (Poecilostomatoida) under sea ice in mid-summer near Syowa Station, Antarctica. Polar Biol 31:561–567
Turner JT (1986) Zooplankton feeding ecology: contents of fecal pellets of the cyclopoid copepods Oncaea venusta, Corycaeus amazonicus, Oithona plumifera, and O. simplex from the Northern Gulf of Mexico. Mar Ecol 7:289–302
Uchio T (1960) Planktonic foraminifera of the Antarctic Ocean. Biological results of the Japanese Antarctic research expedition 11. Seto Mar Biol Lab, Wakayama
Uitz J, Claustre H, Morel A, Hooker SB (2006) Vertical distribution of phytoplankton communities in open ocean: an assessment based on surface chlorophyll. J Geophys Res C Oceans 111:C08005. doi:10.1029/2005JC003207
Acknowledgments
We would like to thank the officers and crew of the icebreaker Shirase and the expedition members of the Japanese Antarctic Research Expedition (JARE) cruises 52 and 53 for collecting data and zooplankton samples along with the CTD data.
Author information
Authors and Affiliations
Corresponding author
Additional information
Part of this research was supported by the National Institute of Polar Research (NIPR) through Project Research no. KP-4. The study is also part of the Science Program of JARE AMB-2. It was supported by the NIPR under MEXT
Rights and permissions
Open Access This article is distributed under the terms of the Creative Commons Attribution License which permits any use, distribution, and reproduction in any medium, provided the original author(s) and the source are credited.
About this article
Cite this article
Ojima, M., Takahashi, K.T., Iida, T. et al. Distribution patterns of micro- and meso-zooplankton communities in sea ice regions of Lützow-Holm Bay, East Antarctica. Polar Biol 36, 1293–1304 (2013). https://doi.org/10.1007/s00300-013-1348-y
Received:
Revised:
Accepted:
Published:
Issue Date:
DOI: https://doi.org/10.1007/s00300-013-1348-y