Abstract
Excessive protein intake causes liver and brain damage and neurotransmitter disorders, thereby inducing cognitive dysfunction. L-theanine can regulate the neurotransmitter content and show great potential in liver and brain protection. However, it remains unclear whether l-theanine effectively regulates neurotransmitter content under high-protein diet. A 40-day feeding experiment was performed in Sprague Dawley rats to investigate the regulatory effects and mechanisms of l-theanine on neurotransmitters via liver–brain axis in high-protein diets. The results showed that a 30% protein diet increased the liver and brain neurotransmitter content while maintaining the normal structure of liver and the hippocampal CA1 of brain and improving the autonomous behavior of rats. In contrast, 40% and 50% protein diets decreased the content of neurotransmitters, affected autonomous behavior, destroyed the hippocampal CA1 of brain structure, increased hepatic inflammatory infiltration, lipid degeneration, and hepatocyte eosinophilic change in liver, increased liver AST, ALT, MDA, CRP, and blood ammonia level, and decreased liver SOD and CAT level. However, l-theanine improved liver and brain neurotransmitter content, autonomous behavior, liver and hippocampal brain structure, and liver biochemical indicators in 40% and 50% protein diets. To explore how LTA can eliminate the adverse effects of a high-protein diet, we analyzed different metabolites and proteomes and using western blotting for validate quantitatively. We found that l-theanine regulates the activity of PF4 and G protein subunit alpha i2, increases the content of brain-derived neurotrophic factor and dopamine under a 20% protein diet. In addition, l-theanine can activate the adenylate cyclase-protein kinase A pathway through the protein alpha/beta-hydrolase domain protein 12 to regulate the content of neurotransmitters under a 40% protein diet, thereby exerting a neuroprotective effect.






Similar content being viewed by others
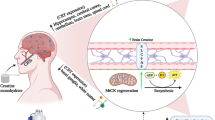
Data Availability
The datasets generated and analyzed during the current study are available from the corresponding author on reasonable request.
Abbreviations
- LTA:
-
L-theanine
- HPD:
-
High protein diet
- AST:
-
Aspartate aminotransferase
- ALT:
-
Alanine aminotransferase
- CAT:
-
Catalase
- MDA:
-
Malonic dialdehyde
- T-SOD:
-
Total superoxide dismutase
- CRP:
-
C-reactive protein
- NE:
-
Norepinephrine
- DA:
-
Dopamine
- 5-HT:
-
5-Hydroxytryptamine
- Glu:
-
Glutamic acid
- ACh:
-
Acetylcholine
- GABA:
-
γ-Aminobutyric acid
- BDNF:
-
Brain-derived neurotrophic factor
- AC:
-
Adenylate cyclase
- PKA:
-
Protein kinase A
- GNAI2:
-
G protein subunit alpha i2
- ABHD12:
-
Alpha/beta-hydrolase domain protein 12
- OFT:
-
Open-field test
- LDT:
-
Light-dark box test
- HE:
-
Hematoxylin-eosin
- NS:
-
Nissl stain
- 2-AG:
-
2-Arachidonoyl glycerol
- AEA:
-
Arachidonoyl ethanolamide
- PF4:
-
PLF4_RAT
References
French WW, Dridi S, Shouse SA, Wu H, Hawley A, Lee S-O, Gu X, Baum JI (2017) A high-protein diet reduces weight gain, decreases food intake, decreases liver fat deposition, and improves markers of muscle metabolism in obese Zucker rats. Nutrients 9(6):587. https://doi.org/10.3390/nu9060587
Ji M, Li S, Dong Q, Hu W (2018) Impact of early high-protein diet on neurofunctional recovery in rats with ischemic stroke. Med Sci Monit 24:2235–2243. https://doi.org/10.12659/msm.906533
Snelson M, Clarke RE, Nguyen TV, Penfold SA, Forbes JM, Tan SM, Coughlan MT (2021) Long term high protein diet feeding alters the microbiome and increases intestinal permeability, systemic inflammation and kidney injury in mice. Mol Nutr Food Res 65(8):e2000851. https://doi.org/10.1002/mnfr.202000851
Snelson M, Mamo JCL, Lam V, Giles C, Takechi R (2017) Differential effects of high-protein diets derived from soy and casein on blood-brain barrier integrity in wild-type mice. Front Nutr 4:35–35. https://doi.org/10.3389/fnut.2017.00035
Butterworth RF (2019) Hepatic encephalopathy in cirrhosis: pathology and pathophysiology. Drugs 79(Suppl 1):17–21. https://doi.org/10.1007/s40265-018-1017-0
Haussinger D (2010) Hepatic encephalopathy. Acta gastro-enterologica Belgica 73(4):457–464
Dugger BN, Dickson DW (2017) Pathology of neurodegenerative diseases. Cold Spring Harb Perspect Biol 9(7):a028035. https://doi.org/10.1101/cshperspect.a028035
Butterworth RF (2013) The liver-brain axis in liver failure: neuroinflammation and encephalopathy. Nat Rev Gastroenterol Hepatol 10(9):522–528. https://doi.org/10.1038/nrgastro.2013.99
Jadeja RN, Rachakonda VP, Khurana S (2018) Targeting cholinergic system to modulate liver injury. Curr Drug Targets 19(8):938–944. https://doi.org/10.2174/1389450118666170619090219
Ochoa-Sanchez R, Rose CF (2018) Pathogenesis of hepatic encephalopathy in chronic liver disease. J Clin Exp Hepatol 8(3):262–271. https://doi.org/10.1016/j.jceh.2018.08.001
Stojanovic IR, Kostic M, Ljubisavljevic S (2014) The role of glutamate and its receptors in multiple sclerosis. J Neural Transm 121(8):945–955. https://doi.org/10.1007/s00702-014-1188-0
Albrecht J, Sidoryk-Wegrzynowicz M, Zielinska M, Aschner M (2010) Roles of glutamine in neurotransmission. Neuron Glia Biol 6(4):263–276. https://doi.org/10.1017/S1740925X11000093
Yang Y, Yang S, Liu J, Feng Y, Qi F, Zhao R (2019) DNA hypomethylation of GR promoters is associated with GR activation and BDNF/AKT/ERK1/2-induced hippocampal neurogenesis in mice derived from folic-acid-supplemented dams. Mol Nutr Food Res 63(12):e1801334. https://doi.org/10.1002/mnfr.201801334
Lu CW, Lin TY, Yang HC, Hung CF, Weng JR, Chang C, Wang SJ (2020) [1-(4-chloro-3-nitrobenzenesulfonyl)-1H-indol-3-yl]-methanol, an indole-3-carbinol derivative, inhibits glutamate release in rat cerebrocortical nerve terminals by suppressing the P/Q-type Ca(2+) channels and Ca(2+)/calmodulin/protein kinase A pathway. Neurochem Int 140:104845. https://doi.org/10.1016/j.neuint.2020.104845
Peng Y, Zhang C, Su Y, Wang Z, Jiang Y (2018) Activation of the hippocampal AC-cAMP-PKA-CREB-BDNF signaling pathway using WTKYR in depression model rats. Electrophoresis. https://doi.org/10.1002/elps.201800381
Blankman JL, Long JZ, Trauger SA, Siuzdak G, Cravatt BF (2013) ABHD12 controls brain lysophosphatidylserine pathways that are deregulated in a murine model of the neurodegenerative disease PHARC. Proc Natl Acad Sci U S A 110(4):1500–1505. https://doi.org/10.1073/pnas.1217121110
Williams JL, Everett JM, D’Cunha NM, Sergi D, Georgousopoulou EN, Keegan RJ, McKune AJ, Mellor DD et al (2020) The effects of green tea amino acid L-theanine consumption on the ability to manage stress and anxiety levels: a systematic review. Plant Foods Hum Nutr 75(1):12–23. https://doi.org/10.1007/s11130-019-00771-5
Deb S, Dutta A, Phukan BC, Manivasagam T, Justin Thenmozhi A, Bhattacharya P, Paul R, Borah A (2019) Neuroprotective attributes of L-theanine, a bioactive amino acid of tea, and its potential role in Parkinson’s disease therapeutics. Neurochem Int 129:104478. https://doi.org/10.1016/j.neuint.2019.104478
Kardashev A, Ratner Y, Ritsner MS (2018) Add-on pregnenolone with L-theanine to antipsychotic therapy relieves negative and anxiety symptoms of schizophrenia: an 8-week, randomized, double-blind, placebo-controlled trial. Clin Schizophr Relat Psychoses 12(1):31–41. https://doi.org/10.3371/csrp.kara.070415
Ogawa S, Ota M, Ogura J, Kato K, Kunugi H (2018) Effects of l-theanine on anxiety-like behavior, cerebrospinal fluid amino acid profile, and hippocampal activity in Wistar Kyoto rats. Psychopharmacology 235(1):37–45. https://doi.org/10.1007/s00213-017-4743-1
Wang D, Gao Q, Zhao G, Kan Z, Wang X, Wang H, Huang J, Wang T et al (2018) Protective effect and mechanism of theanine on lipopolysaccharide-induced inflammation and acute liver injury in mice. J Agric Food Chem 66(29):7674–7683. https://doi.org/10.1021/acs.jafc.8b02293
Lin L, Zeng L, Liu A, Peng Y, Yuan D, Zhang S, Li Y, Chen J et al (2020) l-Theanine regulates glucose, lipid, and protein metabolism via insulin and AMP-activated protein kinase signaling pathways. Food Funct 11(2):1798–1809. https://doi.org/10.1039/C9FO02451D
Zhao H, Du H, Liu M, Gao S, Li N, Chao Y, Li R, Chen W et al (2018) Integrative proteomics–metabolomics strategy for pathological mechanism of vascular depression mouse model. J Proteome Res 17(1):656–669. https://doi.org/10.1021/acs.jproteome.7b00724
Liu A, Lin L, Xu W, Gong Z, Liu Z, Xiao W (2021) l-Theanine regulates glutamine metabolism and immune function by binding to cannabinoid receptor 1. Food Funct 12(13):5755–5769. https://doi.org/10.1039/D1FO00505G
Maia J, Fonseca BM, Cunha SC, Braga J, Goncalves D, Teixeira N (1865) Correia-da-Silva G (2020) Impact of tetrahydrocannabinol on the endocannabinoid 2-arachidonoylglycerol metabolism: ABHD6 and ABHD12 as novel players in human placenta. Biochim Biophys Acta Mol Cell Biol Lipids 12:158807. https://doi.org/10.1016/j.bbalip.2020.158807
Verreijen AM, Engberink MF, Memelink RG, van der Plas SE, Visser M, Weijs PJ (2017) Effect of a high protein diet and/or resistance exercise on the preservation of fat free mass during weight loss in overweight and obese older adults: a randomized controlled trial. Nutr J 16(1):10. https://doi.org/10.1186/s12937-017-0229-6
Kuniishi H, Ichisaka S, Yamamoto M, Ikubo N, Matsuda S, Futora E, Harada R, Ishihara K et al (2017) Early deprivation increases high-leaning behavior, a novel anxiety-like behavior, in the open field test in rats. Neurosci Res 123:27–35. https://doi.org/10.1016/j.neures.2017.04.012
Kulesskaya N, Voikar V (2014) Assessment of mouse anxiety-like behavior in the light–dark box and open-field arena: role of equipment and procedure. Physiology & Behavior 133:30–38. https://doi.org/10.1016/j.physbeh.2014.05.006
Wahl D, Solon-Biet SM, Wang QP, Wali JA, Pulpitel T, Clark X, Raubenheimer D, Senior AM et al (2018) Comparing the effects of low-protein and high-carbohydrate diets and caloric restriction on brain aging in mice. Cell Rep 25(8):2234–2243. https://doi.org/10.1016/j.celrep.2018.10.070
Mendez-Lopez M, Mendez M, Arias J, Arias JL (2015) Effects of a high protein diet on cognition and brain metabolism in cirrhotic rats. Physiol Behav 149:220–228. https://doi.org/10.1016/j.physbeh.2015.05.038
Lee TH, Yang JT, Kato H, Wu JH, Chen ST (2004) Expression of brain-derived neurotrophic factor immunoreactivity and mRNA in the hippocampal CA1 and cortical areas after chronic ischemia in rats. J Neurosci Res 76(5):705–712. https://doi.org/10.1002/jnr.20097
Wang T, Zhao L, Guo Y, Zhang M, Pei H (2015) Picroside II inhibits neuronal apoptosis and improves the morphology and structure of brain tissue following cerebral ischemic injury in rats. PLoS One 10(4):e0124099. https://doi.org/10.1371/journal.pone.0124099
Park S, Kim DS, Kang S, Kim HJ (2018) The combination of luteolin and l-theanine improved Alzheimer disease-like symptoms by potentiating hippocampal insulin signaling and decreasing neuroinflammation and norepinephrine degradation in amyloid-beta-infused rats. Nutr Res 60:116–131. https://doi.org/10.1016/j.nutres.2018.09.010
Liu Y, Zhao P, Cheng M, Yu L, Cheng Z, Fan L, Chen C (2018) AST to ALT ratio and arterial stiffness in non-fatty liver Japanese population:a secondary analysis based on a cross-sectional study. Lipids Health Dis 17(1):275. https://doi.org/10.1186/s12944-018-0920-4
Sproston NR, Ashworth JJ (2018) Role of C-reactive protein at sites of inflammation and infection. Front Immunol 9:754. https://doi.org/10.3389/fimmu.2018.00754
Uyanga VA, Oke EO, Amevor FK, Zhao J, Wang X, Jiao H, Onagbesan OM, Lin H (2022) Functional roles of taurine, L-theanine, L-citrulline, and betaine during heat stress in poultry. J Anim Sci Biotechnol 13(1):23. https://doi.org/10.1186/s40104-022-00675-6
Zhu G, Yang S, Xie Z, Wan X (2018) Synaptic modification by L-theanine, a natural constituent in green tea, rescues the impairment of hippocampal long-term potentiation and memory in AD mice. Neuropharmacology 138:331–340. https://doi.org/10.1016/j.neuropharm.2018.06.030
Gardiner J, Overall R, Marc J (2011) The microtubule cytoskeleton acts as a key downstream effector of neurotransmitter signaling. Synapse 65(3):249–256. https://doi.org/10.1002/syn.20841
Greco AM, Sticchi R, Boschi G, Vetrani A, Salvatore G (1985) Effects of an overload of animal protein on the rat: brain DNA alterations and tissue morphological modifications during fetal and post-natal stage. Int J Vitam Nutr Res 55(1):107–112
Zeng L, Lin L, Chen L, Xiao W, Gong Z (2021) l-Theanine ameliorates d-galactose-induced brain damage in rats via inhibiting AGE formation and regulating sirtuin1 and BDNF signaling pathways. Oxid Med Cell Longev 2021:8850112. https://doi.org/10.1155/2021/8850112
Dineley KT, Pandya AA, Yakel JL (2015) Nicotinic ACh receptors as therapeutic targets in CNS disorders. Trends Pharmacol Sci 36(2):96–108. https://doi.org/10.1016/j.tips.2014.12.002
Ota M, Wakabayashi C, Sato N, Hori H, Hattori K, Teraishi T, Ozawa H, Okubo T et al (2015) Effect of L-theanine on glutamatergic function in patients with schizophrenia. Acta Neuropsychiatr 27(5):291–296. https://doi.org/10.1017/neu.2015.22
Luscher B, Fuchs T (2015) GABAergic control of depression-related brain states. Adv Pharmacol 73:97–144. https://doi.org/10.1016/bs.apha.2014.11.003
Shen M, Yang Y, Wu Y, Zhang B, Wu H, Wang L, Tang H, Chen J (2019) L-theanine ameliorate depressive-like behavior in a chronic unpredictable mild stress rat model via modulating the monoamine levels in limbic-cortical-striatal-pallidal-thalamic-circuit related brain regions. Phytother Res 33(2):412–421. https://doi.org/10.1002/ptr.6237
Gossard TR, Trotti LM, Videnovic A, St Louis EK (2021) Restless legs syndrome: contemporary diagnosis and treatment. Neurotherapeutics 18(1):140–155. https://doi.org/10.1007/s13311-021-01019-4
Nathan PJ, Lu K, Gray M, Oliver C (2006) The neuropharmacology of L-theanine(N-ethyl-L-glutamine): a possible neuroprotective and cognitive enhancing agent. J Herb Pharmacother 6(2):21–30
Sookoian S, Pirola CJ (2012) Alanine and aspartate aminotransferase and glutamine-cycling pathway: their roles in pathogenesis of metabolic syndrome. World J Gastroenterol 18(29):3775–3781. https://doi.org/10.3748/wjg.v18.i29.3775
Tiedje KE, Stevens K, Barnes S, Weaver DF (2010) Beta-alanine as a small molecule neurotransmitter. Neurochem Int 57(3):177–188. https://doi.org/10.1016/j.neuint.2010.06.001
Faiq MA, Wollstein G, Schuman JS, Chan KC (2019) Cholinergic nervous system and glaucoma: from basic science to clinical applications. Prog Retin Eye Res 72:100767. https://doi.org/10.1016/j.preteyeres.2019.06.003
Kowalska MA, Rauova L, Poncz M (2010) Role of the platelet chemokine platelet factor 4 (PF4) in hemostasis and thrombosis. Thromb Res 125(4):292–296. https://doi.org/10.1016/j.thromres.2009.11.023
Chaudhary P, Wainford RD (2021) Neuroanatomical characterization of Galphai2-expressing neurons in the hypothalamic paraventricular nucleus of male and female Sprague-Dawley rats. Physiol Genom 53(1):12–21. https://doi.org/10.1152/physiolgenomics.00097.2020
Lan YL, Wang X, Zou YJ, Xing JS, Lou JC, Zou S, Ma BB, Ding Y et al (2019) Bazedoxifene protects cerebral autoregulation after traumatic brain injury and attenuates impairments in blood-brain barrier damage: involvement of anti-inflammatory pathways by blocking MAPK signaling. Inflamm Res 68(4):311–323. https://doi.org/10.1007/s00011-019-01217-z
Yuan Q, Jia HX, Li SQ, Xiao Z, Wu YJ, Feng L, Liu XL, Sun XC et al (2019) The role of adenosine in up-regulation of p38 MAPK and ERK during limb ischemic preconditioning-induced brain ischemic tolerance. Brain Res 1707:172–183. https://doi.org/10.1016/j.brainres.2018.11.015
Procopio DO, Saba LM, Walter H, Lesch O, Skala K, Schlaff G, Vanderlinden L, Clapp P et al (2013) Genetic markers of comorbid depression and alcoholism in women. Alcohol Clin Exp Res 37(6):896–904. https://doi.org/10.1111/acer.12060
Myeku N, Clelland CL, Emrani S, Kukushkin NV, Yu WH, Goldberg AL, Duff KE (2016) Tau-driven 26S proteasome impairment and cognitive dysfunction can be prevented early in disease by activating cAMP-PKA signaling. Nat Med 22(1):46–53. https://doi.org/10.1038/nm.4011
Persoon CM, Hoogstraaten RI, Nassal JP, van Weering JRT, Kaeser PS, Toonen RF, Verhage M (2019) The RAB3-RIM pathway is essential for the release of neuromodulators. Neuron 104(6):1065–1080. https://doi.org/10.1016/j.neuron.2019.09.015
Ichu TA, Reed A, Ogasawara D, Ulanovskaya O, Roberts A, Aguirre CA, Bar-Peled L, Gao J et al (2020) ABHD12 and LPCAT3 interplay regulates a Lyso-phosphatidylserine-C20:4 phosphatidylserine lipid network implicated in neurological disease. Biochemistry 59(19):1793–1799. https://doi.org/10.1021/acs.biochem.0c00292
Leishman E, Mackie K, Bradshaw HB (2019) Elevated levels of arachidonic acid-derived lipids including prostaglandins and endocannabinoids are present throughout ABHD12 knockout brains: novel insights into the neurodegenerative phenotype. Front Mol Neurosci 12:142. https://doi.org/10.3389/fnmol.2019.00142
Prospero-Garcia O, Ruiz Contreras AE, Ortega Gomez A, Herrera-Solis A, Mendez-Diaz M, Grupo de Neurociencias de la Universidad Nacional Autonoma de M (2019) Endocannabinoids as therapeutic targets. Arch Med Res 50(8):518–526. https://doi.org/10.1016/j.arcmed.2019.09.005
Acknowledgements
We would like to thank Editage for English language editing.
Funding
This work was supported by the Natural Science Foundation of Hunan Province, China(2020JJ4036), National Key Research and Development Program of China (2017YFD0400803), and the key R&D program of Hunan Province (2021NK2016).
Author information
Authors and Affiliations
Contributions
All authors contributed to the study conception and design. Material preparation, data collection, and analysis were performed by Wei Xu and Yuxin Song. The first draft of the manuscript was written by Wei Xu and all authors commented on previous versions of the manuscript. All authors read and approved the final manuscript.
Corresponding authors
Ethics declarations
Ethics Approval
All experimental procedures and animal care were performed in strict accordance with the NIH guidelines for the care and use of laboratory animals (NIH Publication no. 85-23 Rev. 1985). The protocols were approved by the Ethics Committee of Hunan Agriculture University (registry number: 015063506, Changsha, China).
Consent to Participate
Informed consent was obtained from all individual participants included in the study.
Consent for Publication
This manuscript does not contain individual person’s data.
Competing Interests
The authors have no relevant financial or non-financial interests to disclose
Rights and Permissions
Springer Nature or its licensor holds exclusive rights to this article under a publishing agreement with the author(s) or other rightsholder(s); author self-archiving of the accepted manuscript version of this article is solely governed by the terms of such publishing agreement and applicable law.
Additional information
Publisher’s Note
Springer Nature remains neutral with regard to jurisdictional claims in published maps and institutional affiliations.
Supplementary Information
ESM 1
(DOCX 1.11 mb)
Rights and permissions
Springer Nature or its licensor (e.g. a society or other partner) holds exclusive rights to this article under a publishing agreement with the author(s) or other rightsholder(s); author self-archiving of the accepted manuscript version of this article is solely governed by the terms of such publishing agreement and applicable law.
About this article
Cite this article
Xu, W., Song, Y., Xiao, W. et al. Regulatory Effects and Mechanisms of L-Theanine on Neurotransmitters via Liver–Brain Axis Under a High Protein Diet. Mol Neurobiol 61, 783–798 (2024). https://doi.org/10.1007/s12035-023-03608-0
Received:
Accepted:
Published:
Issue Date:
DOI: https://doi.org/10.1007/s12035-023-03608-0