Abstract
The efficiency of power dissipation (η) in a dynamic material model has been conventionally used for qualitative predictions to estimate the hot working conditions at which dynamic recrystallization (DRX) is dominant. However, predicting the quantitative value of the DRX fraction (XDRX) from η remains a challenge. In this paper, a constitutive equation is proposed to quantitatively predict XDRX using η. The proposed equation for describing XDRX is derived from the reaction rate equation using the assumption that the DRX rate depends on η. The proposed equation is verified via hot compression tests of equiaxed Ti–6Al–4V ELI alloys (Ti-64) in the (α + β) region. The predicted and experimental XDRX values are found to be generally consistent with one another, exhibiting an average absolute error of 0.05. Furthermore, the proposed equation provides the same level of prediction accuracy as the conventional Johnson–Mehl–Avrami–Kolmogorov (JMAK) equation. Therefore, the proposed equation can be used to quantitatively predict XDRX following hot compression tests of equiaxed Ti-64. Moreover, compared with the JMAK equation, the proposed equation is expressed in fewer parameters and constant terms. It is, thus, expected to facilitate the quantitative prediction of XDRX.











Similar content being viewed by others
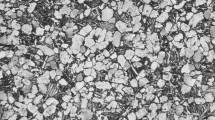
Abbreviations
- T :
-
Temperature
- \(\dot{\varepsilon }\) :
-
Strain rate
- ε :
-
Strain
- σ :
-
Stress
- d DRX :
-
DRX grain size
- D 1 :
-
Material constant
- D 2 :
-
Material constant
- Z :
-
Zener–Hollomon parameter
- R :
-
Gas constant
- Q :
-
Apparent activation energy for hot deformation
- X DRX :
-
Fraction of dynamic recrystallization
- β d :
-
Rate coefficient in the JMAK equation
- k d :
-
Avrami exponent
- ε c :
-
Critical strain at which DRX initiates
- ε p :
-
Peak strain in σ–ε curves
- n 1 :
-
Material constant
- n 2 :
-
Material constant
- ε 0 .5 :
-
ε when XDRX = 0.5
- n 3 :
-
Material constant
- n 4 :
-
Material constant
- n 5 :
-
Material constant
- Q 1 :
-
Material constant
- d 0 :
-
Initial grain size before deformation
- η :
-
Efficiency of power dissipation
- P :
-
Power dissipated during hot working
- G :
-
Power dissipated for the temperature increase due to plastic deformation
- J :
-
Power dissipated during microstructural evolution
- m :
-
Strain rate sensitivity exponent
- K :
-
Material constant
- J max :
-
Maximum value of J
- η′:
-
Modified efficiency of power dissipation
- X Precipitation :
-
Fraction of precipitation
- t :
-
Time
- R 1 :
-
Radius of a particle
- D :
-
Diffusion coefficient of the solute atoms
- N :
-
Number of participating particles per unit volume
- C :
-
Concentration of a chemical substance
- k :
-
Rate coefficient
- μ s :
-
Shear modulus
- b :
-
Magnitude of the Burgers vector
- ρ recrystallized :
-
Average dislocation density of recrystallized grains
- ρ un -recrystallized :
-
Average dislocation density of un-recrystallized grains
- ε com :
-
True compressive strain
- ΔT :
-
Temperature variation due to the work performed on the sample
- η e :
-
Fraction of the mechanical work that is transformed into heat
- c p :
-
Heat capacity of the material
- ρ :
-
Density of the sample
- W :
-
Energy per unit volume that is input into the sample
- A :
-
Constant
- ε e ff :
-
Effective strain
- \({\dot{\varepsilon }}_{\mathrm{eff}}\) :
-
Effective strain rate
- θ :
-
Misorientation angle
- c 1 :
-
Constant
- c 2 :
-
Constant
- X DRX_PRE :
-
XDRX predicted using constitutive equation
- X DRX_EXP :
-
XDRX obtained via experimentation
- AAE :
-
Average absolute error
- i :
-
ith data obtained by varying the compression conditions and observation locations
- I :
-
Total number of data points
- μ :
-
Chemical potential
References
J. Jeswiet, M. Geiger, U. Engel, M. Kleiner, M. Schikorra, J. Duflou, R. Neugebauer, P. Bariani, and S. Bruschi: CIRP JMST., 2008, vol. 1, pp. 2–17.
F. Chen, Z. Cui, and J. Chen: Manuf. Rev., 2014, vol. 1(6), pp. 1–21.
K. Huang and R.E. Loge: Mater. Des., 2016, vol. 111, pp. 548–74.
C.M. Sellars: Mater. Sci. Technol., 1990, vol. 6, pp. 1072–81.
C. Zener and J.H. Hollomon: J. Appl. Phys., 1994, vol. 15, pp. 22–32.
C.M. Sellars and J.A. Whiteman: Met. Sci., 1979, vol. 13, pp. 187–94.
F.J. Humphreys and M. Hatherly: Recrystallization and Related Annealing Phenomena, 1st ed. Pergamon Press, Oxford, 1995, pp. 188–95.
H. Matsumoto and V. Velay: J. Alloys Compd., 2017, vol. 708, pp. 404–13.
A. Laasraoui and J.J. Jonas: Metall. Trans. A., 1991, vol. 22A, pp. 151–60.
S.I. Kim, Y. Lee, D.L. Lee, and Y.C. Yoo: Mater. Sci. Eng. A, 2003, vol. 355, pp. 384–93.
H.S. Jeong, J.R. Cho, and H.C. Park: J. Mater. Process. Technol., 2005, vol. 162–163, pp. 504–11.
D. Xu, M. Zhu, Z. Tang, and C. Sun: J. Wuhan Univ. Technol. Mat. Sci., 2013, vol. 28, pp. 819–24.
G.Z. Quan, G.C. Luo, J.T. Liang, D.S. Wu, A. Mao, and Q. Liu: Comput. Mater. Sci., 2015, vol. 97, pp. 136–47.
G.Z. Quan, D.S. Wu, G.C. Luo, Y.F. Xia, J. Zhou, Q. Liu, and L. Gao: Mater. Sci. Eng. A., 2014, vol. 589, pp. 23–33.
S.M. Abbasi, A. Momeni, Y.C. Lin, and H.R. Jafarian: Mater. Sci. Eng. A, 2016, vol. 665, pp. 154–60.
Y.V.R.K. Prasad, H.L. Gegel, S.M. Doraivelu, J.C. Malas, J.T. Morgan, K.A. Lark, and D.R. Barker: Metall. Trans. A, 1984, vol. 15A, pp. 1883–92.
Y.V.R.K. Prasad and T. Seshacharyulu: Int. Mater. Rev., 1998, vol. 43, pp. 243–58.
A. Lukaszek-Solek, J. Krawczyk, T. Sleboda, and J. Grelowski: J. Mater. Res. Technol., 2019, vol. 8, pp. 3281–90.
S. Venugopal, M. Vasudevan, S. Venugopal, P.V. Sivaprasad, S.K. Jha, P. Pandey, S.L. Mannan, and Y.V.R.K. Prasad: Mater. Sci. Technol., 1996, vol. 12, pp. 955–62.
F.L. Sui, L.X. Xu, L.Q. Chen, and X.H. Liu: J. Mater. Process. Technol., 2011, vol. 211, pp. 433–40.
Y. Yamashita, Y. Li, H. Matsumoto, Y. Koizumi, and A. Chiba: Mater. Trans., 2011, vol. 52, pp. 780–86.
T. Seshacharyulu, S.C. Medeiros, W.G. Frazier, and Y.V.R.K. Prasad: Mater. Sci. Eng. A, 2000, vol. 284, pp. 184–94.
T. Seshacharyulu, S.C. Medeiros, W.G. Frazier, and Y.V.R.K. Prasad: Mater. Sci. Eng. A, 2002, vol. 325, pp. 112–25.
Y.V.R.K. Prasad, T. Seshacharyulu, S.C. Medeiros, and W.G. Frazier: J. Mater. Process. Technol., 2001, vol. 108, pp. 320–27.
N.K. Park, J.T. Yeom, and Y.S. Na: J. Mater. Process. Technol., 2002, vol. 130–131, pp. 540–45.
J. Luo, P. Ye, M.Q. Li, and L.Y. Liu: Mater. Des., 2015, vol. 88, pp. 32–40.
C. Wert and C. Zener: J. Appl. Phys., 1950, vol. 21, pp. 5–8.
P. Atkins, J. de Paula, and J. Keeler: Atkins’ Physical chemistry, 11th ed. Oxford University Press, Oxford, 2018, pp. 731–33, 787–89.
Y.P. Li, E. Onodera, H. Matsumoto, Y. Koizumi, S. Yu, and A. Chiba: ISIJ Int., 2011, vol. 51, pp. 782–87.
Y.P. Li, E. Onodera, H. Matsumoto, and A. Chiba: Metall. Mater. Trans. A, 2009, vol. 40A, pp. 982–90.
M.C. Mataya and V.E. Sackschewsky: Metall. Mater. Trans. A, 1994, vol. 25A, pp. 2737–52.
Y.P. Li, H. Matsumoto, and A. Chiba: Metall. Mater. Trans. A, 2009, vol. 40A, pp. 1203–09.
H. Matsumoto, L. Bin, S.H. Lee, Y. Li, Y. Ono, and A. Chiba: Metall. Mater. Trans. A, 2013, vol. 44A, pp. 3245–60.
T. Furu, K. Marthinsen, and E. Nes: Mater. Sci. Technol., 1990, vol. 6, pp. 1093–1102.
H. Rottenberg: Biophys. J., 1973, vol. 13, pp. 503–11.
I. Weiss, F.H. Froes, D. Eylon, and G.E. Welsch: Metall. Trans. A, 1986, vol. 17A, pp. 1935–47.
Q. Chao, P.D. Hodgson, and H. Beladi: Metall. Mater. Trans. A, 2014, vol. 45A, pp. 2659–71.
S. Gourdet and F. Montheillet: Acta Mater., 2003, vol. 51, pp. 2685–99.
L.S. Toth, Y. Estrin, R. Lapovok, and C. Gu: Acta Mater., 2010, vol. 58, pp. 1782–94.
Author information
Authors and Affiliations
Corresponding author
Ethics declarations
Conflict of interest
The authors declare that they have no conflict of interest.
Additional information
Publisher's Note
Springer Nature remains neutral with regard to jurisdictional claims in published maps and institutional affiliations.
Rights and permissions
About this article
Cite this article
Korenaga, S., Honda, M., Yamanaka, K. et al. Novel Constitutive Equation for Predicting Dynamic Recrystallization During Hot Working Considering the Efficiency of Power Dissipation. Metall Mater Trans A 53, 2163–2173 (2022). https://doi.org/10.1007/s11661-022-06658-6
Received:
Accepted:
Published:
Issue Date:
DOI: https://doi.org/10.1007/s11661-022-06658-6