Abstract
Sulfate-reducing bioreactors, also called biochemical reactors, represent a promising option for passive treatment of mining-influenced water (MIW) based on similar technology to aerobic/anaerobic-constructed wetlands and vertical-flow wetlands. MIW from each mine site has a variety of site-specific properties related to its treatment; therefore, design factors, including the organic substrates and inorganic materials packed into the bioreactor, must be tested and evaluated before installation of full-scale sulfate-reducing bioreactors. Several full-scale sulfate-reducing bioreactors operating at mine sites provide examples, but holistic understanding of the complex treatment processes occurring inside the bioreactors is lacking. With the recent introduction of high-throughput DNA sequencing technologies, microbial processes within bioreactors may be clarified based on the relationships between operational parameters and key microorganisms identified using high-resolution microbiome data. In this review, the test design procedures and precedents of full-scale bioreactor application for MIW treatment are briefly summarized, and recent knowledge on the sulfate-reducing microbial communities of field-based bioreactors from fine-scale monitoring is presented.Key points
• Sulfate-reducing bioreactors are promising for treatment of mining-influenced water.
• Various design factors should be tested for application of full-scale bioreactors.
• Introduction of several full-scale passive bioreactor systems at mine sites.
• Desulfosporosinus spp. can be one of the key bacteria within field-based bioreactors.



Similar content being viewed by others
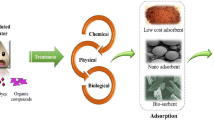
References
Aoyagi T, Hamai T, Hori T, Sato Y, Kobayashi M, Sato Y, Inaba T, Ogata A, Habe H, Sakata T (2017) Hydraulic retention time and pH affect the performance and microbial communities of passive bioreactor for treatment of acid mine drainage. AMB Express 7(1):142. https://doi.org/10.1186/s13568-017-0440-z
Aoyagi T, Hamai T, Hori T, Sato Y, Kobayashi M, Sato Y, Inaba T, Ogata A, Habe H, Sakata T (2018) Microbial community analysis of sulfate-reducing passive bioreactor for treating acid mine drainage under failure conditions after long-term continuous operation. J Environ Chem Eng 6:5795–5800. https://doi.org/10.1016/j.jece.2018.09.003
Bailey MT, Gandy CJ, Jarvis AP (2016) Reducing life-cycle costs of passive mine water treatment by recovery of metals from treatment wastes. Proceedings of the International Mine Water Association Symposium, p 1255–1262
Baldwin SA, Khoshnoodi M, Rezadehbashi M, Taupp M, Hallam S, Mattes A, Sanei H (2015) The microbial community of a passive biochemical reactor treating arsenic, zinc, and sulfate-rich seepage. Front Bioeng Biotechnol 3:27. https://doi.org/10.3389/fbioe.2015.00027
Baldwin SA, Mattes A, Rezadehbashi M, Taylor J (2016) Seasonal microbial population shifts in a bioremediation system treating metal and sulfate-rich seepage. Minerals 6:36. https://doi.org/10.3390/min6020036
Bomberg M, Mäkinen J, Salo M, Arnold M (2017) Microbial community structure and functions in ethanol-fed sulfate removal bioreactors for treatment of mine water. Microorganisms 5:61. https://doi.org/10.3390/microorganisms5030061
Briones AM, Daugherty BJ, Angenent LT, Rausch KD, Tumbleson ME, Raskin L (2007) Microbial diversity and dynamics in multi- and single-compartment anaerobic bioreactors processing sulfate-rich waste streams. Environ Microbiol 9:93–106. https://doi.org/10.1111/j.1462-2920.2006.01119.x
British Standards Institution (2011) PAS 100: 2011 Specification for composted materials. ISBN 978 0 580 653070
Chang IS, Shin PK, Kim BH (2000) Biological treatment of acid mine drainage under sulfate-reducing conditions with solid waste materials as substrate. Water Res 34:1269–1277. https://doi.org/10.1016/S0043-1354(99)00268-7
Colmer AR, Hinkle ME (1947) The role of microorganisms in acid mine drainage: a preliminary report. Science 106(2751):253–256. https://doi.org/10.1126/science.106.2751.253
Dar SA, Kleerebezem R, Stams AJM, Kuenen JG, Muyzer G (2008) Competition and coexistence of sulfate-reducing bacteria, acetogens and methanogens in a lab-scale anaerobic bioreactor as affected by changing substrate to sulfate ratio. Appl Microbiol Biotechnol 78:1045–1055. https://doi.org/10.1007/s00253-008-1391-8
Drennan DM, Almstrand R, Lee I, Landkamer L, Figueroa L, Sharp JO (2016) Organoheterotroph bacterial abundance associates with zinc removal in lignocellulose-based sulfate-reducing systems. Environ Sci Technol 50:378–387. https://doi.org/10.1021/acs.est.5b04268
Drennan DM, Almstrand R, Ladderud J, Lee I, Landkamer L, Figueroa L, Sharp JO (2017) Spatial impacts of inorganic ligand availability and localized microbial community structure on mitigation of zinc laden mine water in sulfate-reducing bioreactors. Water Res 115:50–59. https://doi.org/10.1016/j.watres.2017.02.037
Duncan WFA, Mattes AG, Gould WG, Goodazi F (2004) Multi-stage biological treatment system for removal of heavy metal contaminants. Waste Processing and Recycling in Mineral and Metallurgical Industries V, Proceedings of the 5th International Symposium; 43rd Annual Conference of Metallurgists of CIM. Hamilton, p 469–483
Ettner DC (1999) Pilot scale constructed wetland for the removal of nickel from tailings drainage, Sourthern Norway. Proceedings of the International Mine Water Association Symposium, Sevilla, pp 207–211
Ettner DC (2007) Passive mine water treatment in Norway. Proceedings of the International Mine Water Association Symposium, Cagliari, pp 187–191
Figueroa L, Miller A, Zaluski M, Bless D (2007) Evaluation of a two stage passive treatment approach for mining influenced water. Proceedings of the 24th National Meeting of the American Society of Mining and Reclamation, Gillette, pp 238–247. https://doi.org/10.21000/JASMR07010238
Fu F, Wang Q (2011) Removal of heavy metal ions from wastewaters: a review. J Environ Manag 92:407–418. https://doi.org/10.1016/j.jenvman.2010.11.011
Gibert O, de Pablo J, Cortina JL, Ayora C (2004) Chemical characterization of natural organic substrates for biological mitigation of acid mine drainage. Water Res 38:4186–4196. https://doi.org/10.1016/j.waters.2004.06.023
Gusek J, Wildeman T, Miller A, Fricke J (1998) The challenges of designing, permitting and building a 1,200 gpm passive bioreactor for metal mine drainage West Fork mine, Missouri. Proceedings of the 15th National Meeting of the American Society for Surface Mining and Reclamation, St. Louis, p 203–212. https://doi.org/10.21000/JASMR98010203
Gusek J, Mann C, Wildeman TR, Murphy D (2000) Operational results of a 1,200 gpm passive bioreactor for metal mine drainage, West Fork, Missouri. Proceedings from the 5th International Conference on Acid Rock Drainage, Littleton
Gusek J, Shipley B, Lindsay D (2005) Overcoming access issues at a remote passive treatment site near Lake Shasta, CA. Proceedings of the 22nd National Meeting of the America Society of Mining and Reclamation, Breckenridge, pp 443–453. https://doi.org/10.21000/JASMR05010443
Gusek J, Buchanan R, Sorells D (2013) Infiltration-diverting cap and full-scale biochemical reactor operation at the Iron King/Copper Chief Mine, Arizona. Proceedings of the International Mine Water Association Symposium, Golden, pp 581–586
Hiibel SR, Pereyra LP, Inman LY, Tischer A, Reisman DJ, Reardon KF, Pruden A (2008) Microbial community analysis of two field-scale sulfate-reducing bioreactors treating mine drainage. Environ Microbiol 10:2087–2097. https://doi.org/10.1111/j.1462-2920.2008.01630.x
Hiibel SR, Pereyra LP, Riquelme Breazeal MV, Reisman DJ, Reardon KF, Pruden A (2011) Effect of organic substrate on the microbial community structure in pilot-scale sulfate-reducing biochemical reactors treating mine drainage. Environ Eng Sci 28:563–571. https://doi.org/10.1089/ess2010.0237
Hori T, Kimura M, Aoyagi T, Navarro RR, Ogata A, Sakoda A, Katayama Y, Takasaki M (2014) Biodegradation potential of organically enriched sediments under sulfate- and iron-reducing conditions as revealed by 16S rRNA deep sequencing. J Water Environ Technol 12:357–366. https://doi.org/10.2965/jwet.2014.357
Hori T, Aoyagi T, Itoh H, Narihiro T, Oikawa A, Suzuki K, Ogata A, Friedrich MW, Conrad R, Kamagata Y (2015) Isolation of microorganisms involved in reduction of crystalline iron(III) oxides in natural environments. Front Microbiol 6:386. https://doi.org/10.3389/fmicb.2015.00386
Jarvis A, Gandy C, Bailey M, Davis J, Orme P, Malley J, Potter H, Moorhouse A (2015) Metal removal and secondary contamination in a passive metal mine drainage treatment system. Proceedings of the International Mine Water Association Symposium
Kimura S, Hallberg KB, Johnson DB (2006) Sulfidogenesis in low pH (3.8–4.2) media by a mixed population of acidophilic bacteria. Biodegradation. 17:159–167. https://doi.org/10.1007/s10532-005-3050-4
Kolmert Å, Johnson DB (2001) Remediation of acidic waste waters using immobilised, acidophilic sulfate-reducing bacteria. J Chem Technol Biotechnol 76:836–843. https://doi.org/10.1002/jctb.453
Kurniawan TA, Chan GYS, Lo W-H, Babel S (2006) Physico-chemical treatment technologies for wastewater laden with heavy metals. Chem Eng J 118:83–98. https://doi.org/10.1016/j.cej.2006.01.015
Kuyucak N, Chabot F, Martschuk J (2006) Successful implementation and operation of a passive treatment system in an extremely cold climate, northern Quebec, Canada. Proceedings of the 7th International Conference on Acid Rock Drainage, St. Louis, p 980–922. https://doi.org/10.21000/JASMR06020980
Lewis AE (2010) Review of metal sulfide precipitation. Hydrometallurgy 104:222–234. https://doi.org/10.1016/j.hydromet.2010.06.010
Liu A, Garcia-Dominguez E, Rhine ED, Young LY (2004) A novel arsenate respiring isolate that can utilize aromatic substrates. FEMS Microbiol Ecol 48:323–332. https://doi.org/10.1016/j.femsec.2004.02.008
Mardanov AV, Panova IA, Beletsky AV, Avakyan MR, Kadnikov VV, Antsiferov DV, Banks D, Frank YA, Pimenov NV, Ravin NV, Karnachuk OV (2016) Genomic insights into a new acidophilic, copper-resistant Desulfosporosinus isolate from the oxidized tailings area of an abandoned gold mine. FEMS Microbiol Ecol 92:fiw11. https://doi.org/10.1093/femsec/fiw111
McCullough CD, Lund MA (2011) Bioremediation of acidic and metalliferous drainage (ADM) through organic carbon amendment by municipal sewage and green waste. J Environ Manag 92:2419–2426. https://doi.org/10.1016/j.jenvman.2011.04.011
Mine Safety and Explosives Control Division, Commerce, Distribution and Industrial Safety Policy Group (2013) Act on special measures for pollution caused by the metal mining industry, etc. Policy index of Ministry of Economy, Trade and Industry, Japan
Nairn RW (2013) Carbon dioxide impacts both passive treatment system effectiveness and carbon footprint. Proceedings of the International Mine Water Association Symposium, p 673–678
Nairn RW, LaBar JA, Strevett KA, Strosnider WH, Morris D, Neely CA, Garrido A, Santamaria B, Oxenford L Kauk K, Carter S, Furneaux B (2010) A large, multi-cell, ecologically engineered passive treatment system for ferruginous lead-zinc mine waters. Proceedings of the International Mine Water Association Symposium, p 255–259
Neculita CM, Zagury GJ, Bussiere B (2007) Passive treatment of acid mine drainage in bioreactors using sulfate-reducing bacteria: critical review and research needs. J Environ Qual 36:1–16. https://doi.org/10.2134/jeq2006.0066
Neculita CM, Yim GJ, Lee G, Ji SW, Jung JW, Park HS (2011) Comparative effectiveness of mixed organic substrates to mushroom compost for treatment of mine drainage in passive bioreactors. Chemosphere 83:76–82. https://doi.org/10.1016/j.chemosphere.2010.11.082
Pereyra LP, Hiibel SR, Pruden A, Reardon FK (2008) Comparison of microbial community composition and activity in sulfate-reducing batch systems remediating mine drainage. Biotechnol Bioeng 101:702–713. https://doi.org/10.1002/bit.21930
Price WA, Errington JC (1998) Guidelines for metal leaching and acid rock drainage at mine sites in British Columbia. Ministry of Energy and Mines, British Columbia
Rakotonimaro TV, Neculita CM, Bussière B, Benzaazoua M, Zagury GJ (2017) Recovery and reuse of sludge from active and passive treatment of mine drainage-impacted waters: a review. Environ Sci Pollut Res 24:73–91. https://doi.org/10.1007/s11356-016-7733-7
Raskin L, Rittmann BE, Stahl DA (1996) Competition and coexistence of sulfate-reducing and methanogenic populations in anaerobic biofilms. Appl Environ Microbiol 62:3847–3857
Rezadehbashi M, Baldwin SA (2018) Core sulphate-reducing microorganisms in metal-removing semi-passive biochemical reactors and the co-occurrence of methanogens. Microorganisms 6:16. https://doi.org/10.3390/microorganisms6010016
Sánchez-Andrea I, Rodríguez N, Amils R, Sanz JL (2011) Microbial diversity in anaerobic sediments at Rio Tinto, a naturally acidic environment with a high heavy metal content. Appl Environ Microbiol 77:6085–9603. https://doi.org/10.1128/AEM.00654-11
Sánchez-Andrea I, Sanz JL, Bijmans MFM, Stams AJM (2014) Sulfate reduction at low pH to remediate acid mine drainage. J Hazard Mater 269:98–109. https://doi.org/10.1016/j.jhazmat.2013.12.032
Sato Y, Hori T, Navarro RR, Habe H, Ogata A (2016) Functional maintenance and structural flexibility of microbial communities perturbed by simulated intense rainfall in a pilot-scale membrane bioreactor. Appl Microbiol Biotechnol 100(14):6477–6456. https://doi.org/10.1007/s00253-016-7466-z
Sato Y, Hamai T, Hori T, Habe H, Kobayashi M, Sakata T (2018) Year-round performance of the passive sulfate-reducing bioreactor using rice bran as an organic substrate for treating acid mine drainage. Mine Water Environ 37:586–594. https://doi.org/10.1007/s10230-017-0489-6
Sato Y, Hamai T, Hori T, Aoyagi T, Inaba T, Kobayashi M, Habe H, Sakata T (2019a) Desulfosporosinus spp. were the most predominated sulfate-reducing bacteria in pilot- and laboratory-scale passive bioreactors for acid mine drainage treatment. Appl Microbiol Biotechnol 103:7783–7793
Sato Y, Hori T, Koike H, Navarro RR, Ogata A, Habe H (2019b) Transcriptome analysis of activated sludge microbiomes reveals an unexpected role of minority nitrifiers in carbon metabolism. Commun Biol 2:179. https://doi.org/10.1038/s42003-019-0418-2
Skousen J, Zipper CE, Rose A, Ziemkiewicz PF, Nairn R, McDonald LM, Kleinmann RL (2017) Review of passive systems for acid mine drainage treatment. Mine Water Environ 36:133–153. https://doi.org/10.1007/s10230-016-0417-1
The Interstate Technology & Regulatory Council Biochemical Reactors for Mining-Influenced Waste Team (2013) Biochemical reactors for mining-influenced water
United States Environmental Protection Agency (2000) Liquid assets 2000: America’s water resources at a turning point EPA-840-b-00-001
Vasquez Y, Escobar MC, Neculita CM, Arbeli Z, Roldan F (2016) Biochemical passive reactors for treatment of acid mine drainage: effect of hydraulic retention time on changes in efficiency, composition of reactive mixture, and microbial activity. Chemosphere 153:244–253. https://doi.org/10.1016/j.chemosphere.2016.03.052
Wang LK, Vaccari DA, Li Y, Shammas NK (2004) Chemical precipitation. In: Wang LK, Hung YT, Li Y, Shammas NK (eds) Physiological treatment process, vol 3. Humana Press, New Jersey, pp 141–198
Widdel F (1992) The genus Desulfotomaculum. In: Balows A, Triiper HG, Dworkin M, Harder W, Schleifer KH (eds) The prokaryotes. Springer-Verlag, New York, pp 1792–1799. https://doi.org/10.1007/0-387-30744-3_25
Zagury GJ, Kulnieks VJ, Neculia CM (2006) Characterization and reactivity assessment of organic substrates for sulfate-reducing bacteria in acid mine drainage treatment. Chemosphere 64:944–954. https://doi.org/10.1016/j.chemosphere.2006.01.001
Zhang M, Wang H (2014) Organic wastes as carbon sources to promote sulfate reducing bacterial activity for biological remediation of acid mine drainage. Miner Eng 69:81–90. https://doi.org/10.1016/j.mineng.2014.07.010
Zhao J, Fang D, Zhang P, Zhou L (2017) Long-term effects of increasing acidity on low-pH sulfate-reducing bioprocess and bacterial community. Environ Sci Pollut Res 24:4067–4076. https://doi.org/10.1007/s11356-016-8147-2
Author information
Authors and Affiliations
Contributions
All authors conceived the manuscript scope and contents. HH and YS prepared the first draft of the manuscript. TA, TI, and TH performed the literature search for microbiome. TH, KH, and MK performed the literature search for precedents of full-scale bioreactors. TS and NS performed the literature search for design factors. All authors read and approved the manuscript.
Corresponding authors
Ethics declarations
Conflict of interest
The authors declare that they have no conflict of interest.
Ethical approval
This article does not contain any studies with human participants or animals performed by any of the authors.
Additional information
Publisher’s note
Springer Nature remains neutral with regard to jurisdictional claims in published maps and institutional affiliations.
Rights and permissions
About this article
Cite this article
Habe, H., Sato, Y., Aoyagi, T. et al. Design, application, and microbiome of sulfate-reducing bioreactors for treatment of mining-influenced water. Appl Microbiol Biotechnol 104, 6893–6903 (2020). https://doi.org/10.1007/s00253-020-10737-2
Received:
Revised:
Accepted:
Published:
Issue Date:
DOI: https://doi.org/10.1007/s00253-020-10737-2