Abstract
This work described the development of a cationic polylactic acid (PLA)-based nanoparticles (NPs) as an antigen delivery system using dimethyldioctadecylammonium bromide (DDA) to facilitate the engulfment of BSA-FITC by porcine alveolar macrophages (3D4/2 cells) and heat-labile enterotoxin subunit B (LTB) to enhance the transport of BSA-FITC across M cells. The experimental design methodology was employed to study the influence of PLA, polyvinyl alcohol (PVA), DDA, and LTB on the physical properties of the PLA-based NPs. The size of selected cationic PLA NPs comprising 5% PLA, 5% PVA, and 0.6% DDA with or without LTB absorption was range from 367 to 390 nm with a polydispersity index of 0.26, a zeta potential of + 26.00 to + 30.55 mV, and entrapment efficiency of 41.43%. Electron micrographs revealed NPs with spherical shape. The release kinetic of BSA from the NPs followed the Korsmeyer-Peppas kinetics. The cationic PLA NPs with LTB surface absorption showed 3-fold increase in BSA-FITC transported across M cells compared with the NPs without LTB absorption. The uptake studies demonstrated 2-fold increase in BSA-FITC intensity in 3D4/2 cells with cationic NPs as compared with anionic NPs. Overall, the results suggested that LTB decreased the retention time of BSA-FITC loaded in the cationic PLA NPs within the M cells, thus promoting the transport of BSA-FITC across the M cells, and cationic NPs composed of DDA help facilitate the uptake of BSA-FITC in the 3D4/2 cells. Further studies in pigs with respiratory antigens will provide information on the efficacy of cationic PLA NPs as a nasal antigen carrier system.









Similar content being viewed by others
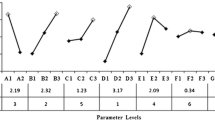
References
Neutra MR, Kozlowski PA. Mucosal vaccines: the promise and the challenge. Nat Rev Immunol. 2006;6(2):148–58.
Woodrow KA, Bennett KM, Lo DD. Mucosal vaccine design and delivery. Annu Rev Biomed Eng. 2012;14:17–46.
Illum L. Nasal drug delivery-recent developments and future prospects. J Control Release. 2012;161(2):254–63.
Vajdy M, O’Hagan DT. Microparticles for intranasal immunization. Adv Drug Deliv Rev. 2001;51(1):127–41.
Wu HY, Nguyen HH, Russell MW. Nasal lymphoid tissue (NALT) as a mucosal immune inductive site. S Scand J of immunol. 1997;46(5):506–13.
Zuercher AW, Coffin SE, Thurnheer MC, Fundova P, Cebra JJ. Nasal-associated lymphoid tissue is a mucosal inductive site for virus-specific humoral and cellular immune responses. J Immunol. 2002;168(4):1796–803.
Gullberg E, Leonard M, Karlsson J, Hopkins AM, Brayden D, Baird AW, et al. Expression of specific markers and particle transport in a new human intestinal M-cell model. Biochem Biophys Res Commun. 2000;279(3):808–13.
Jani P, Halbert GW, Langride J, Florence AT. The uptake and translocation of latex nanospheres and microspheres after oral administration to rats. J Pharm Pharmacol. 1989;41:809–12.
Mabbott NA, Donaldson DS, Ohno H, Williams IR, Mahajan A. Microfold (M) cells: important immunosurveillance posts in the intestinal epithelium. Mucosal Immunol. 2013;6(4):666–77.
Irvine DJ, Hanson MC, Rakhra K, Tokatlian T. Synthetic nanoparticles for vaccines and immunotherapy. Chem Rev. 2015;115(19):11109–46.
Pati R, Shevtsov M, Sonawane A. Nanoparticle vaccines against infectious diseases. Front Immunol. 2018;9.
McGhee JR, Mestecky J, Dertzbaugh MT, Eldridge JH, Hirasawa M, Kiyono H. The mucosal immune system: from fundamental concepts to vaccine development. Vaccine. 1992;10(2):75–88.
Mahapatro A, Singh DK. Biodegradable nanoparticles are excellent vehicle for site directed in-vivo delivery of drugs and vaccines. J Nanobiotechnology. 2011;9:55.
Gregory AE, Titball R, Williamson D. Vaccine delivery using nanoparticles. Front Cell Infect Microbiol. 2013;3:13.
Vila A, sánchez A, Évora C, Soriana I, Vila Jato JL, Alonso ML. PEG-PLA nanoparticles as carriers for nasal vaccine delivery. J Aerosol Med 2004;17:174–185.
Davis SS. Nasal vaccines. Adv Drug Deliv Rev. 2001;51(1–3):21–42.
Partidos CD. Intranasal vaccines: forthcoming challenges. Pharmaceut Sci Tech Today. 2000;3(8):273–81.
Slutter B, Bal S, Keijzer C, Mallants R, Hagenaars N, Que I, et al. Nasal vaccination with N-trimethyl chitosan and PLGA based nanoparticles: nanoparticle characteristics determine quality and strength of the antibody response in mice against the encapsulated antigen. Vaccine. 2010;28(38):6282–91.
Hofland HE, Shephard L, Sullivan SM. Formation of stable cationic lipid/DNA complexes for gene transfer. Proc Natl Acad Sci U S A. 1996;93(14):7305–9.
Qu W, Li N, Yu R, Zuo W, Fu T, Fei W, et al. Cationic DDA/TDB liposome as a mucosal vaccine adjuvant for uptake by dendritic cells in vitro induces potent humoural immunity. Artif Cells Nanomed Biotechnol. 2018;46(sup1):852–60.
Khademi F, Derakhshan M, Yousefi-Avarvand A, Najafi A, Tafaghodi M. A novel antigen of Mycobacterium tuberculosis and MPLA adjuvant co-entrapped into PLGA:DDA hybrid nanoparticles stimulates mucosal and systemic immunity. Microb Pathog. 2018;125:507–13.
Christensen D, Foged C, Rosenkrands I, Nielsen HM, Andersen P, Agger EM. Trehalose preserves DDA/TDB liposomes and their adjuvant effect during freeze-drying. Biochim Biophys Acta. 2007;1768(9):2120–9.
Katz D, Lehrer S, Galan O, Lachmi B, Cohen S, Inbar I, et al. Unique Immunomodulating properties of dimethyl dioctadecyl ammonium bromide (DDA) in experimental viral vaccines. In: Cohen S, Shafferman A, editors. Novel strategies in the design and production of vaccines. Boston, MA: Springer US; 1996. p. 115–25.
Kirby DJ, Rosenkrands I, Agger EM, Andersen P, Coombes AG, Perrie Y. PLGA microspheres for the delivery of a novel subunit TB vaccine. J Drug Target. 2008;16(4):282–93.
Korsholm KS, Agger EM, Foged C, Christensen D, Dietrich J, Andersen CS, et al. The adjuvant mechanism of cationic dimethyldioctadecylammonium liposomes. Immunology. 2007;121(2):216–26.
Bannunah AM, Vllasaliu D, Lord J, Stolnik S. Mechanisms of nanoparticle internalization and transport across an intestinal epithelial cell model: effect of size and surface charge. Mol Pharm. 2014;11(12):4363–73.
Dillon A, Lo DD. M cells: intelligent engineering of mucosal immune surveillance. Front Immunol. 2019;10:1499.
Jaganathan K, Vyas S. Strong systemic and mucosal immune responses to surface-modified PLGA microspheres containing recombinant hepatitis B antigen administered intranasally. Vaccine. 2006;24(19):4201–11.
Paulus GL, Xavier RJ. Autophagy and checkpoints for intracellular pathogen defense. Curr Opin Gastroenterol. 2015;31(1):14–23.
Sipos A, Kim K-J, Chow RH, Flodby P, Borok Z, Crandall ED. Alveolar epithelial cell processing of nanoparticles activates autophagy and lysosomal exocytosis. Am J Phys Lung Cell Mol Phys. 2018;315(2):L286–300.
Schulz O, Pabst O. Antigen sampling in the small intestine. Trends Immunol. 2013;34(4):155–61.
Pridgen EM, Alexis F, Farokhzad OC. Polymeric nanoparticle drug delivery technologies for oral delivery applications. Expert Opin Drug Deliv. 2015;12(9):1459–73.
Cervin J, Wands AM, Casselbrant A, Wu H, Krishnamurthy S, Cvjetkovic A, et al. GM1 ganglioside-independent intoxication by cholera toxin. PLoS Pathog. 2018;14(2):e1006862.
Frey A, Giannasca KT, Weltzin R, Giannasca PJ, Reggio H, Lencer WI, et al. Role of the glycocalyx in regulating access of microparticles to apical plasma membranes of intestinal epithelial cells: implications for microbial attachment and oral vaccine targeting. J Exp Med. 1996;184(3):1045–59.
World Health Organization (WHO), guideline on stability evaluation of vaccines, (2006).
USP32-NF27. <71> Sterility Tests. Rockville, Md: United States Pharmacopeial Convention, Inc; 2009.
Chaikhumwang P, Nilubol D, Tantituvanont A, Chanvorachote P. A new cell-to-cell interaction model for epithelial microfold cell formation and the enhancing effect of epidermal growth factor. Eur J Pharm Sci. 2017;106:49–61.
Rose F, Wern JE, Ingvarsson PT, van de Weert M, Andersen P, Follmann F, et al. Engineering of a novel adjuvant based on lipid-polymer hybrid nanoparticles: a quality-by-design approach. J Control Release. 2015;210:48–57.
Yusuf H, Nugraheni R, Setyawan D. Effect of cellulose derivative matrix and oligosaccharide on the solid state and physical characteristics of dimethyldioctadecylammoniumliposomes for vaccine. Res Pharm Sci. 2019;14(1):11.
Zhai W, Ko Y, Zhu W, Wong A, Park CB. A study of the crystallization, melting, and foaming behaviors of polylactic acid in compressed CO(2). Int J Mol Sci. 2009;10(12):5381–97.
Nikolic L, Ristic I, Adnadjevic B, Nikolic V, Jovanovic J, Stankovic M. Novel microwave-assisted synthesis of poly(D,L-lactide): the influence of monomer/initiator molar ratio on the product properties. Sensors (Basel). 2010;10(5):5063–73.
Rachmawati H, Curcumin-Loaded PLA. Nanoparticles: formulation and physical evaluation. Sci Pharm. 2016;84(1):191–202.
Yang Y-Y, Chia H-H, Chung T-S. Effect of preparation temperature on the characteristics and release profiles of PLGA microspheres containing protein fabricated by double-emulsion solvent extraction/evaporation method. J Control Release. 2000;69(1):81–96.
Leo E, Forni F, Bernabei MT. Surface drug removal from ibuprofen-loaded PLA microspheres. Int J Pharm. 2000;196(1):1–9.
Kirby DJ, Kaur R, Agger EM, Andersen P, Bramwell VW, Perrie Y. Developing solid particulate vaccine adjuvants: surface bound antigen favours a humoural response, whereas entrapped antigen shows a tendency for cell mediated immunity. Curr Drug Deliv. 2013;10:268–78.
Costa P, Lobo JMS. Modeling and comparison of dissolution profiles. Eur J Pharm Sci. 2001;13:11.
Niebergall PJ, Milosovich G, Goyan JE. Dissolution rate studies II. J Pharm Sci. 1963;52(3):236–41.
Talmage DW, Dixon FJ. The influence of adjuvants on the elimination of soluble protein antigens and the associated antibody responses. J Infect Dis. 1953;93(2):176–80.
Delputte PL, Vanderheijden N, Nauwynck HJ, Pensaert MB. Involvement of the matrix protein in attachment of porcine reproductive and respiratory syndrome virus to a heparinlike receptor on porcine alveolar macrophages. J Virol. 2002;76(9):4312–20.
Pang H-B, Braun GB, Ruoslahti E. Neuropilin-1 and heparan sulfate proteoglycans cooperate in cellular uptake of nanoparticles functionalized by cationic cell-penetrating peptides. Sci Adv. 2015;1(10):e1500821.
Rojanasakul Y, Wang LY, Bhat M, Glover DD, Malanga CJ, Ma JKH. The transport barrier of epithelia-a comparative study on membrane permeability and charge selectivity in the rabbit. Pharm Res. 1992;9(8):6.
Funding
This study was supported by Research and Researchers for Industries (RRI, grant number PHD58I0037), and Agricultural Research Development Agency (Public Organization) (ARDA, grant number CRP5705010990). Furthermore, the partial funding was supported by Chulalongkorn University Special Task Force for Activation Research (CU-STAR): swine virus evolution and vaccine research (SVEVR).
Author information
Authors and Affiliations
Corresponding author
Ethics declarations
Conflict of Interest
The authors declare that they have no conflict of interest.
Additional information
Publisher’s Note
Springer Nature remains neutral with regard to jurisdictional claims in published maps and institutional affiliations.
Rights and permissions
About this article
Cite this article
Chaikhumwang, P., Kitsongsermthon, J., Manopakdee, K. et al. Cationic Polylactic Acid-Based Nanoparticles Improve BSA-FITC Transport Across M Cells and Engulfment by Porcine Alveolar Macrophages. AAPS PharmSciTech 21, 134 (2020). https://doi.org/10.1208/s12249-020-01689-x
Received:
Accepted:
Published:
DOI: https://doi.org/10.1208/s12249-020-01689-x