Abstract
Culture broth, cell pellet suspension and cell free supernatant of 14 Bacillus subtilis isolates obtained from different Egyptian locations were checked for their ability to repress egg hatching and juvenile (J2) activity of root-knot nematode, Meloidogyne incognita under laboratory environment. Treatments using culture broth of B. subtilis isolates B10 and B8, at a concentration of 50%, recorded lowest percentages of hatched eggs reaching 44.7 and 46.3%, respectively. Culture broth of B. subtilis isolate B10 at the same concentration showed a higher percentage of juvenile mortality reached 99.7%. Batch fermentation was completed, using B. subtilis isolate B10 (Accession No. EF583055), which gave the lowest percentage of hatched eggs and the highest percentage of juvenile mortality of M. incognita, for maximizing biomass production and suppression effects of culture broth. Batch fermentation no. 2, which started in a bioreactor with optical density of 0.5, was the best process that achieved a higher cell biomass and percentage of juvenile mortality of 4.52 g/l and 74.3%, respectively, using culture broth of 5%. Under greenhouse conditions, culture broth, cell pellet suspension, and cell-free supernatant of B. subtilis isolate B10 were used to test their potential for reducing number of galls and egg masses in the roots of tomato plants. The treatment of using culture broth at a concentration 10 ml/pot, 2 × 109 cfu/ml in a soil infested with M. incognita, was highly significant in decreasing number of galls and egg masses reaching the average of 9.3 and 6.7, with reduction percentage of 81.1 and 89.5%, respectively, compared with the control treatment of M. incognita only. In addition, B. subtilis isolate B10 was formulated and applied as bionematicide to test its efficiency in reducing number of galls and egg masses. Treatment with bioformulation at a concentration of 0.1 g/pot was more significant than the other concentrations in reducing number of galls and egg masses, reaching the average of 12 and 7 with a reduction percentage of 69.7 and 71.2%, correspondingly.
Similar content being viewed by others
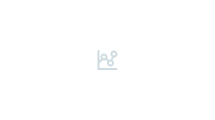
Background
The root-knot nematodes, Meloidogyne spp., are one of the highly important soil-borne pathogens that cause great economic damages to horticultural and field crops, and considered one of the most dangerous plant-parasitic nematodes, which can infect approximately all of the world’s main crop plants (Oka et al. 2000). Nematodes generally habitat the soil and usually attack the underground parts of plants; therefore, their management is a very complex (Stirling 1991). Different methods can be utilized for controlling Meloidogyne spp. such as cultural practices, resistant cultivars, and chemical nematicides which are easy for application and more effective. Concerns about public health and environmental safety are pushing for reducing chemical nematicides used in controlling of Meloidogyne spp. Thus, improvement of new alternative of management is needed, especially environmentally friendly methods. Management of Meloidogyne spp. can be achieved, using biocontrol agents (Siddiqui 2002 and Khan et al. 2008). Huang et al. (2016) reported that the highest ovicidal activity against M. incognita documented, using a combination of Syncephalastrum racemosum and Paecilomyces lilacinus at a concentration of 50%, reduced 70% of egg hatching than the control. Moreover, the treatment with S. racemosum, at a concentration of 50%, showed a best larvicidal activity, where the rate of mortality reached its highest value of 96.7%. Bacillus spp. can produce lytic enzymes, cyclic lipopeptides (Gray et al. 2006), and many secondary metabolites that play an essential role in biocontrol of Meloidogyne spp. Many lytic enzymes are produced by B. cereus, including chitinase and glucanse (Csuzi 1978). These lytic enzymes have been demonstrated to be antagonistic to M. javanica (Wepuhkhulu et al. 2011). Cyclic lipopeptides of surfactin and iturin produced by B. subtilis were more able to inhibit egg hatching of M. incognita and play an imperative role in increasing the percentage of juvenile mortality (Kavitha et al. 2012). The main goal of fermentation process is scaling-up production of cell biomass and the biological products. Matar et al. (2009) maximized cell biomass of B. subtilis isolate G-GANA7, as a bioagent against some plant pathogens, using batch fermentation strategy that achieved a cell biomass of 3.2 g/l. Scaling-up production of surfactin (lipopeptide) from B. subtilis to be used as a bioagent was performed in an innovative bioreactor, maximum concentration of surfactin achievement was 6.45 g/l (Yeh et al. 2006). Population densities and galls of root-knot nematodes, Meloidogyne spp. decreased by using the treatments with Pseudomonas aeruginosa, B. subtilis, and antagonistic fungus P. lilacinus (Prakob et al. 2009). Also, B. cereus X5 was effective in suppression of root-knot nematodes, hence number of galls of tomato roots decreased compared to the control (Tong-Jian et al. 2012). Burkett-Cadena et al. (2008) reported that formulations (contained strains of bacilli) of Equity® (multiple strains), BioYield® (two strains), and RhizoVital® (strain FZB42) reduced M. incognita eggs per gram root, juvenile nematodes per gram of soil, and galls per plant on tomato.
The main objective of this study was to evaluate the antagonistic and suppression effects of culture broth, cell pellet suspension, and cell free supernatant of B. subtilis isolates on egg hatching and juvenile (J2) activity of M. incognita under laboratory conditions. In addition, to maximize both biomass production and suppression effects of the promising B. subtilis isolate, using batch fermentation. As well, to evaluate their efficacy to reduce number of galls and egg masses in the roots of tomato plants under greenhouse conditions.
Materials and methods
Bacterial isolates and root-knot nematode, M. incognita
Fourteen B. subtilis isolates were isolated during 2002–03 from 5 locations in Egypt (El-Malaha, El-Amria, El-Nobaria, Abo-Homos, and El-Sharkia) by Dr. Abo-Zaid, G.A., Bioprocess Development Department, Genetic Engineering and Biotechnology Research Institute (GEBRI), City of Scientific Research and Technological Applications (SRTA-City), Egypt. All the isolates were identified according to the morphological, biochemical, and physiological tests recommended by Sneath et al. (1986) and Collee et al. (1996). Seven B. subtilis isolates; B1, B4, B7, B8, B10, B11, and B14 were identified based on the sequencings of 16S rRNA gene. The root-knot nematode, M. incognita used in this study was provided by Plant Pathology Department, Faculty of Agriculture, Alexandria University, Egypt.
Cultivation in shake-flask
Growth of the 14 B. subtilis cultures was conducted in shake flasks under constant controlled conditions of temperature, pH, and agitation. Bacterial colonies of B. subtilis isolates were inoculated into a nutrient liquid medium (peptone, 5 g/l and beef extract, 3 g/l) and incubated overnight (16 h) at 30 °C, with a constant shaking at 200 rpm. 1 ml of each culture was transferred into a 250 ml Erlenmeyer flask containing 49 ml of number 3 medium of which each 1 L contained 10 g peptone, 10 g glucose, 1 g KH2PO4 and 0.5 g MgSO4. 7H2O in distilled water and pH was adjusted to 7 (Asaka and Shoda 1996). Cultures were incubated overnight at 30 °C and shacked at 200 rpm until reaching 2 × 109 cfu/ml. 20 ml of each culture was centrifuged at 5590×g for 20 min, and its cell free supernatant was collected and passed through a syringe filter (0.2 μ). Also, cell pellet of each isolate was collected, washed, and centrifuged at 5590×g for 20 min. Cell pellet of each culture was suspended in 20 ml of sterile distilled water. The culture broth, cell-free supernatant, and cell pellet of these bacterial isolates were used for treatments in dilutions of their original concentrations, namely, 1:1 (50%), 1:4 (25%), and 1:10 (10%).
In vitro suppressive effect of B. subtilis isolates against M. incognita
Culture broth, cell pellet suspension, and cell-free supernatant of each B. subtilis isolate were examined for its ability to inhibit egg hatching and juvenile (J2) activity. Approximately 50 eggs and 50 juveniles (J2) were transferred into each well of a 12-well plate. Afterwards, culture broth, cell pellet suspension, and cell-free supernatant of the 14 B. subtilis isolates were added separately at a concentration of 50%. Culture broth, cell pellet suspension and cell-free supernatant of the five B. subtilis isolates; B4, B5, B7, B8 and B10 were added at the concentrations of 25 and 10%. The control treatment included medium free from B. subtilis isolates. The plates were covered and incubated at 25 °C for 72 h. Three replicates/treatment were analyzed. The percentages of hatched eggs and survival of juveniles were counted under microscope (Huang et al. 2016).
Fermentation experiment
Bioreactor (fermentor)
Batch fermentation was performed, according to Matar et al. (2009), in a working volume of 4 L in a 10 L bench-top bioreactor (Cleaver, Saratoga, USA), prepared with three 6-bladed disc-turbine impeller and four baffles, and joined to a digital control unit. The process was automated through a control unit provided with control panel of 10.4ˮ color touch-screen interface and storage program up to 59,994 programs for different kinds of conditions. Temperature and pH were adjusted at 30 °C and 7, correspondingly. pH was controlled by automatic feed of 2 N NaOH and 2 N HCl. Sterilized air passed through sterile filter was supplied, originally at 0.5 VVM (air volume per medium volume per minute). Agitation rate ranged between 200 and 400 rpm to maintain the dissolved oxygen level above 20%. METTLER TOLEDO electrodes were used to determine the dissolved oxygen level and pH values on-line.
Batch fermentation
Two batch fermentation runs of B. subtilis isolate B10 (Accession No. EF583055), which gave the lowest percentage of hatched eggs and the highest percentage of juvenile mortality of M. incognita, were started in a fermentor, with an optical density of 0.2 and 0.5 at 550 nm, using number 3 medium (Asaka and Shoda 1996). Shake flask pre-cultured seed was prepared as follows: a single colony of B. subtilis isolate B10 was inoculated into a 500 ml Erlenmeyer flask containing 100 ml of production medium of N3 medium. Bacterial isolate was cultured overnight at 30 °C and shacked at 200 rpm. During the time of the two batch fermentation, several samples of culture were taken and cell number was determined by measuring culture optical density at 550 nm. Glucose level was estimated enzymatically (Matar et al. 2009), using (GOD-PAD) colorimetric kit (Diamond Diagnostic, Egypt). Ability of culture broth of B. subtilis isolate B10 to antagonize and inhibit juvenile (J2) activity of M. incognita at a concentration of 5% (1:20) was tested (Huang et al. 2016). Dry cell weight was estimated by centrifuging 10 ml sample at 894×g for 10 min, and the pellet was re-suspended, washed, and centrifuged again as before. Pellets were then dried overnight in a dry-air oven at 80 °C (Van Dam-Mieras et al. 1992).
Suppressive effect of B. subtilis isolate B10 against M. incognita under greenhouse conditions
A pot experiment was carried out under greenhouse conditions, using tomato plants as a host plant. Twenty four pots (25 × 25 cm in diameter) were filled by 5 kg mixture of sterilized clay and sand (2:1 v/v), and the nematode eggs were applied at the rate of 1000 eggs/pot, 3 days after transferring tomato seedlings (30 days old) to the pots. Culture broth, cell pellet suspension, and cell-free supernatant of B. subtilis isolate B10 were applied at a concentration (10 ml/pot, 2 × 109 cfu/ml) twice, 3 days after nematode inoculation and 1 month after the first treatment as a soil drench. Each treatment was accomplished by three replicates. Eight treatments were performed as follows: (1) M. incognita as a check treatment; (2) untreated control; (3) culture broth; (4) cell pellet suspension (5) cell-free supernatant; (6) M. incognita + culture broth; (7) M. incognita + cell pellet suspension; and (8) M. incognita + cell-free supernatant. The plants were up-rooted after 60 days then root galls and egg masses were determined. Also, the fresh and dry weight of shoots and roots were determined. The roots were stained for 15 min in an aqueous solution of Phloxine B stain (0.15 g/l water), then washed with running tap water to remove residual stain and detect the presence of nematode egg masses (Holbrook et al. 1983).
Formulation experiment and preparation of B. subtilis isolate B10
Talc powder (TP) was used for preparing a bioformulation. The culture broth containing 2.0 × 109 cfu/m1 of B. subtilis isolate B10 was used for the preparation. To 400 ml of culture broth, 2 g of glucose and 10 g of carboxymethylcellulose (CMC) were added as additives. Glucose served as carbon source for keeping the cells viable, while CMC acted as an adhesive. The culture broth and additives were mixed uniformly on a vortex mixer. 15 g of calcium carbonate was added to 1 kg of talc powder (TP) and mixed well for adjusting the pH to 7.0. Four hundreds ml of culture broth with additives were mixed with 1 kg of the talc powder. The formulation was shade-dried to reduce the moisture content to ~ 20% and then packed in UV-sterilized polythene bags and sealed (Vidhyasekaran and Muthamilan 1995).
Effect of formulated B. subtilis isolate B10 on M. incognita under greenhouse conditions
A pot experiment was carried out under greenhouse conditions, using tomato plants as a host plant. Twenty seven pots (15 × 15 cm in diameter) were filled by 3 kg mixture of clay and sand (2:1 v/v), and the nematode eggs were applied at the rate of 1000 eggs/pot, 3 days after transferring tomato seedlings (30 days old) to pots. Formulation contained B. subtilis isolate B10 was applied at a concentration of 0.02, 0.06, and 0.1 g/pot twice, 3 days after nematode inoculation and 1 month after the first treatment, as a soil drench. Each treatment was accomplished by three replicates. Nine treatments were performed as follows: (1) M. incognita as a check treatment; (2) untreated control; (3) formulation 0.02 g/pot; (4) formulation 0.06 g/pot (5) formulation 0.1 g/pot; (6) M. incognita + formulation 0.02 g/pot; (7) M. incognita + formulation 0.06 g/pot; (8) M. incognita + formulation 0.1 g/pot; and (9) M. incognita + Vydete (2 ml/l). The plants were up-rooted after 50 days, and then root galls and egg masses were determined. The fresh and dry weight of shoots and roots were determined as mentioned before (Holbrook et al. 1983).
Statistical analysis
All data obtained from laboratory bioassay and pots experiments were analyzed using analysis of variance (ANOVA). The significant differences among treatments were determined according to the least significant differences (LSD) at P < 0.05 level of probability, using the CoStat software.
Results and discussion
Culture broth, cell pellet suspension, and cell-free supernatant of all B. subtilis isolates were tested for their ability to suppress both egg hatching and juvenile (J2) activity at a concentration of 50%. Five B. subtilis isolates, B4, B5, B7, B8, and B10, were also tested at different concentration of 25 and 10%. Not all B. subtilis isolates were capable to inhibit egg hatching of M. incognita at a concentration of 50%, except B3, B5, B8, B10, and B14. Culture broth and supernatant of B. subtilis isolates B10 and B8 had a maximum activity against egg hatching of M. incognita; hence, the percentages of hatched eggs were 44.7 and 46.3%, using a culture broth, while it reached 53.3 and 54.7%, respectively, by using the supernatant. Cell pellet suspension of B. subtilis isolate B3 recorded the lowest percentage of hatched eggs, reaching 67% compared with the treatments with cell pellet suspension of B. subtilis isolates B10 and B8, where the percentage of hatched eggs recorded 78.3 and 81.7%, respectively (Fig. 1). At a concentration of 25%, culture broth and supernatant of B. subtilis isolates B10 and B8 inhibited egg hatching of M. incognita with percentages of hatched eggs of 57.3 and 59%, using culture broth, respectively, while the percentages of hatched eggs were 67 and 68% by using supernatant, respectively. Cell pellet suspension of B. subtilis isolate B10 and B8 recorded approximately 85.7 and 86.3%, respectively, of hatched eggs (Fig. 2). Capability of B. subtilis isolates to suppress egg hatching at a concentration of 10% was lowest, the percentages of hatched eggs in the treatment, using the culture broth and supernatant of B. subtilis isolate B10, were 68 and 73.7%, respectively, while the treatment, using cell pellet suspension recorded approximately 91.3% egg hatching (Fig. 2). Culture broth and cell-free supernatant of all B. subtilis isolates had antagonistic effect against juvenile (J2) activity of M. incognita compared with those at the highest concentration of 50%, whereas cell pellet suspension of B. subtilis isolates B2 and B13 did not show any suppression effects. Culture broth of B. subtilis isolate B10 showed highly percentage of juvenile mortality, followed by B8 and B7, which reached 99.7, 89, and 88.3%, respectively. Furthermore, the treatments of using supernatant of B. subtilis isolate B10, B8, and B7 showed 90.7, 88.3, and 82.3% mortality, respectively. In the treatment of using cell pellet suspension, the percentage of juvenile mortality did not exceed 39.3% with B. subtilis isolate B10 (Fig. 3). Treatment with culture broth and supernatant of B. subtilis isolate B10, at concentrations of 25 and 10%, achieved the highest percentages of juvenile mortality (97.3 and 89%) by using culture broth, respectively, while it reached 83.3 and 77.3% by using supernatant, respectively. The percentages of juvenile mortality were the lowest in the treatment using cell pellet suspension at concentrations of 25 and 10%. Cell pellet suspension of B. subtilis isolate B10 recorded highest percentages of juvenile mortality that reached 25.3 and 18.7%, respectively, compared with the other isolates (Fig. 4). Nematicidal activity of culture broth and cell-free supernatant of B. subtilis isolates, used in this study as biocontrol agents, may be referred to the ability of these isolates to produce lytic enzymes such as chitinase, glucanase, and protease that affect nematode cuticles and eggs (Page et al. 2014 and Chen et al. 2015) and cyclic lipopeptides such as iturin and surfactin, that may influence nematode behavior (Castaneda-Alvarez and Aballay 2016). The treatment of using B. cereus achieved a lower egg hatching rate and a higher mortality of J2 of root-knot nematodes, Meloidogyne spp. than the control, which related to extracellular proteins or substances produced in filtrate (Xiao et al. 2012 and Huang et al. 2016). Also, Kavitha et al. (2012) suggested that cyclic lipopeptides of surfactin and iturin produced by B. subtilis were responsible for suppression egg hatching of M. incognita and increasing the percentage of juvenile mortality.
Effect of culture broth, cell pellet, and supernatant of five Bacillus subtilis isolates (B4, B5, B7, B8, and B10, C is a control) on egg hatching of Meloidogyne incognita at a concentration of 25% and 10%. Values with the identical letters above the bar are not significantly different at P < 0.05 level
Effect of culture broth, cell pellet, and supernatant of five Bacillus subtilis isolate (B4, B5, B7, B8, and B10, C is a control) on juvenile mortality of Meloidogyne incognita at a concentration of 25% and 10%. Values with the identical letters above the bar are not significantly different at P < 0.05 level
Batch fermentation of B. subtilis isolate B10, which gave the lowest percentage of hatched eggs and the highest percentage of juvenile mortality of M. incognita, was carried out in a 10 L bench-top bioreactor (Cleaver, Saratoga, USA) to achieve maximum biomass production and suppressive effect using culture broth. In batch fermentation no. 1 that started in a bioreactor with an optical density of 0.2 (inoculums size) at 550 nm, the maximum biomass obtained was 2.015 g/l at 8 h. Glucose concentration was decreased gradually reaching 3.2 g/l at 12 h. Percentage of juvenile mortality of M. incognita [at a concentration of 5% (1:20)] at 2 h recorded 1% and reached its highest value of 61% at 8 h (Fig. 5). The culture grew exponentially with a specific growth rate of 0.2 h−1 (Fig. 6). The culture was maintained at a higher value of dissolved oxygen which reduced step by step after 1.5 h, representing bacterial cells growth and glucose consumption from the culture broth. The dissolved oxygen reduced as a result of the rising in O2 require for growing the culture (Fig. 7). To assure a sufficient oxygen supply, oxygen was reserved at above (20%) by raising the agitation speed. In batch fermentation no. 2 that started in a bioreactor with optical density of 0.5 at 550 nm, biomass, percentage of juvenile mortality of M. incognita and glucose concentration of B. subtilis isolate B10 plotted against time, the maximum biomass achieved was 4.52 g/l at 10 h (Fig. 8). Percentage of juvenile mortality of M. incognita [at a concentration of 5% (1:20)] at 2 h recorded 13.3% and reached its highest value of 74.3% at 10.5 h. Glucose concentration decreased rapidly reaching 0 g/l at 7 h. Cell mass increased exponentially over time with a constant specific growth rate of 0.09 h−1 within the exponential phase, resulted from the logarithmic relationship (Fig. 9). In this figure, the values of the lnX (values of X were obtained by using the correlation coefficient δ, 0.5 calculated from the linear relationship among dry mass weight and optical density shown in Fig. 10) were presented by a straight line on a semi-logarithm plot against time. The behavior in this phase allowed estimating the yield coefficient YX/S (0.66 g cells/g glucose) as showed in Fig. 11. Culture was maintained at a higher value of dissolved oxygen, which decreased gradually representing cell growth and glucose consumption from the culture broth. The reason for decreasing the dissolved oxygen refers to increase in O2 demand for culture growth (Fig. 12). To guarantee a sufficient oxygen supply, oxygen was kept above 20% by raising the agitation speed from 200 to 500 rpm. After 10 h, the dissolved oxygen increased gradually. Matar et al. (2009) maximized biomass and activity of cell-free supernatant of B. subtilis isolate G-GANA7, using batch fermentation in bioreactor.
Yield coefficient for growth of Bacillus subtilis isolate B10 on glucose. X0 represents the cell mass in the broth at initial time t0 (g/l); X, the cell mass concentration in the broth at time t (g/l); S, glucose concentration in the broth at time t (g/l); S0, glucose concentration in the broth at initial time t0 (g/l)
All treatments of culture broth, cell pellet suspension, and cell-free supernatant of B. subtilis isolate B10 were able to reduce number of galls and egg masses in the roots of tomato plants. Treatment using culture broth in a soil infected with M. incognita was highly significant that decreased number of galls and egg masses to reach the average of 9.3 and 6.7 with reduction percentages of 81.1 and 89.5%, respectively, than the treatment with M. incognita only whereas number of galls and eggs masses recorded an average of 49.3 and 64, respectively. In the treatment of using cell pellet suspension, number of galls and egg masses reached the average of 18 and 10 with reduction percentages of 63.5 and 84.4%, respectively, while treatment using supernatant was less effective in decreasing number of galls and egg masses, which reached the averages of 27 and 21 with reduction percentages of 45.2 and 67.2%, respectively (Table 1). Siddiqui (2002) and Mokbel (2013) reported that bacterial cell free-filtrates of B. subtilis isolates was less effective in reducing M. javanica and M. arenaria multiplication on infected tomato plants than treatment by sporulated bacterial cells suspension that was highly significant. Treatment with culture broth of B. subtilis isolate B10 was efficient in the induction and stimulation the growth of tomato plants in comparison with the other treatments. Fresh and dry weights of both shoots and roots were determined (Table 2). The treatment of using the culture broth in a soil infected with M. incognita encouraged the growth of both shoots and roots of tomato plants, fresh weight of shoots and roots reached 65.9 g and 17.4 g with increase of 59.9% and 17.8%, respectively, whereas dry weight reached 13.03 g and 8.2 g with an increase of 53.1 and 22%, respectively. In the treatment with culture broth only, fresh weight of shoots and roots reached 74.3 and 21.6 g with increase of 64.5 and 33.8%, respectively, while dry weight reached 14.03 g and 9.5 g with increase of 56.4 and 32.6%, respectively, than the control. Similar results were obtained by Mokbel and Alharbi (2014) and Xiong et al. (2015).
B. subtilis isolate B10 was formulated and applied as bionematicide versus the treatment of Vydate (nematicide) as a standard to test its efficiency in reducing number of galls and egg masses in the roots of tomato plants caused by M. incognita under greenhouse conditions. Treatment with formulated B. subtilis isolate B10 at a concentration of 0.1 g/pot in a soil infected with M. incognita was more significant and effective in reducing number of galls and egg masses than the other concentrations of formulation that were closed to Vydate effect. Number of galls and egg masses decreased to reach the average of 12 and 7 with reduction percentages of 69.7 and 71.2%, respectively, while the treatment of using Vydate decreased number of galls and egg masses to reach the averages of 4.7 and 2.7 with reduction percentages of 88.2 and 89%, respectively, than the treatment with M. incognita only; hence, number of galls and egg masses recorded the average of 39.7 and 24.3, correspondingly (Table 3). Burkett-Cadena et al. (2008) documented that Equity®, BioYield® and RhizoVital® formulations reduced number of galls per plant on tomato.
Conclusion
In conclusion, biocontrol agents can be used as an alternative approach of the chemical nematicides for controlling various nematodes. B. subtilis is recommended for the management of root-knot nematode, M. incognita. However, further investigations are required to determine the formulation that can achieve the best results.
References
Asaka O, Shoda M (1996) Biocontrol of Rhizoctonia solani damping-off of tomato with Bacillus subtilis RB14. Appl and Envir Micro 62:4081–4408
Burkett-Cadena M, Kokalis-Burelle N, Lawrence KS, Santen EV, Kloepper JW (2008) Suppressiveness of root-knot nematodes mediated by rhizobacteria. Biol Control 47:55–59
Castaneda-Alvarez C, Aballay E (2016) Rhizobacteria with nematicide aptitude: enzymes and compounds associated. World J Microb Biotech 32:203
Chen L, Jiang H, Cheng Q et al (2015) Enhanced nematicidal potential of the chitinase pachi from Pseudomonas aeruginosa in association with Cry21Aa. Sci Rep 5:14395
Collee JG, Fraser AG, Marmion BP, Simmons A (1996) Practical medical microbiology. Tests for the identification of bacteria, 14th edn. Churchill Livingstone, New York, pp 131–149
Csuzi S (1978) The induction of a lytic enzyme in cultures of Bacillus cereus. Acta Biochim Biophys Acad Sci Hungaria 13:41–42
Gray EJ, Lee KD, Souleimanov AM, Falco MRD, Zhou X, Charles TC, Driscoll BT, Smith DL (2006) A novel bacteriocin, thuricin 17, produced by plant growth promoting rhizobacteria strain Bt NEB17: isolation and classification. J Appl Microbiol 100:545–554
Holbrook CC, Knauft DA, Dikson DW (1983) A technique for screening peanut for resistance to Meloidogyne arenaria. Plant Dis 57:957–958
Huang WK, Cui JK, Liu SM, Kong LA, Wu QS, Huan Peng H, He WT, Sun JH, Peng DL (2016) Testing various biocontrol agents against the root-knot nematode (Meloidogyne incognita) in cucumber plants identifies a combination of Syncephalastrum racemosum and Paecilomyces lilacinus as being most effective. Biol Control 92:31–37
Kavitha PG, Jonathan EI, Nakkeeran S (2012) Effects of crude antibiotic of Bacillus subtilis on hatching of eggs and mortality of juveniles of Meloidogyne incognita. Nematol Medit 40:203–206
Khan Z, Kim SG, Jeon YH, Kh HU, Son SH, Kim YH (2008) A plant growth promoting rhizobacterium, Paenibacillus polymyxa strain GBR-1, suppresses root-knot nematode. Bioresour Technol 99:3016–3023
Matar SM, El-Kazzaz SA, Wagih EE, El-diwany AI, Hafez EE, Moustafa HE, Abo-Zaid GA, Serour EA (2009) Molecular characterization and batch fermentation of Bacillus subtilis as biocontrol agent, II. Biotechnology 8:35–43
Mokbel AA (2013) Impact of some antagonistic organisms in controlling Meloidogyne arenaria infecting tomato plants. J Life Sci Tech 1:69–74
Mokbel AA, Alharbi AA (2014) Suppressive effect of some microbial agents on root-knot nematode, Meloidogyne javanica infected eggplant. Aust J Crop Sci 8:1428–1434
Oka Y, Kolta H, Bar-Eyal M, Mor M, Sharon E, Chet I, Spiegel Y (2000) New strategies for the control of plant-parasitic nematodes. Pest Manag Sci 56:983–988
Page AP, Stepek G, Winter AD, Pertab D (2014) Enzymology of the nematode cuticle: a potential drug target. Int J Parasitol Drugs Drug Resist 4:133–141
Prakob W, Nguen-Hom J, Jaimasit P, Silapapongpri S, Thanunchai J, Chaisuk P (2009) Biological control of lettuce root knot disease by use of Pseudomonas aeruginosa, Bacillus subtilis and Paecilomyces lilacinus. J Agric Tech 5:179–191
Siddiqui A (2002) Suppression of Meloidogyne javanica by Pseudomonas aeruginosa and Bacillus subtilis in tomato. Nema Mediterr 30:125–130
Sneath PHA, Mair NS, Sharpe ME, Holt JG (1986) Bergey’s manual of systematic bacteriology. In: Endospore-forming gram-positive rods and cocci, vol 2. Williams and Wilkins, Baltimore, pp 1104–1139
Stirling GR (1991) Biological control of plant parasitic nematode: progress, problems and prospects. CAB International, Wallington
Xiao TJ, Tan SY, Shen QR, Ran W (2012) Bacillus cereus X5 suppresses root-knot nematode of tomato by colonizing in roots and soil. Afr J Microbiol Res 6:2321–2327
Van Dam-Mieras MCE, Jeu WH, Vries J, Currell BR, James JW, Leach CK, Patmore RA (1992) Techniques used in bioproduct analysis. Butterworth-Heinemann Ltd., Oxford, p 16
Vidhyasekaran P, Muthamilan M (1995) Development of formulation of Pseudomonas fluorescens for control of chickpea wilt. Plant Dis 79:782–786
Wepuhkhulu M, Kimenju J, Anyango B, Wachira P, Kyallo G (2011) Effect of soil fertility management practices and Bacillus subtilis on plant parasitic nematodes associated with common bean, Phaseolus vulgaris. Trop Subtrop Agro Ecosyst 13:27–34
Yeh M, Wei Y, Chang J (2006) Bioreactor design for enhanced carrier-assisted surfactin production with Bacillus subtilis. Process Biochem 4:1799–1805
Acknowledgements
The authors thank Prof. Ibrahim, I.K.A. and Prof. El-Saeedy, A. at Plant Pathology Department, Faculty of Agriculture, Alexandria University, El-Shatby, Alexandria, Egypt. Also, Prof. Hafez, E.E. and Dr. Kenawy, A.M. at City of Scientific Research and Technological Applications, Alexandria, Egypt for their critical reading and revision.
Funding
Not applicable
Availability of data and materials
All data and material are available.
Author information
Authors and Affiliations
Contributions
All authors read and approved the final manuscript.
Corresponding author
Ethics declarations
Ethics approval and consent to participate
Not applicable.
Consent for publication
Not applicable
Competing interests
The authors declare that they have no competing interests.
Publisher’s Note
Springer Nature remains neutral with regard to jurisdictional claims in published maps and institutional affiliations.
Rights and permissions
Open Access This article is distributed under the terms of the Creative Commons Attribution 4.0 International License (http://creativecommons.org/licenses/by/4.0/), which permits unrestricted use, distribution, and reproduction in any medium, provided you give appropriate credit to the original author(s) and the source, provide a link to the Creative Commons license, and indicate if changes were made.
About this article
Cite this article
Basyony, A.G., Abo-Zaid, G.A. Biocontrol of the root-knot nematode, Meloidogyne incognita, using an eco-friendly formulation from Bacillus subtilis, lab. and greenhouse studies. Egypt J Biol Pest Control 28, 87 (2018). https://doi.org/10.1186/s41938-018-0094-4
Received:
Accepted:
Published:
DOI: https://doi.org/10.1186/s41938-018-0094-4