Abstract
The ecological impacts of urbanization and eutrophication on zooplankton communities in urban waterbodies have recently gained wide interest. Study findings vary across species and urban waterbodies. How the development of such changes affects the variation of biotic assemblages has only been explored to a limited extent in tropical urban lakes.
Spatial and temporal variations of zooplankton community structure in 11 urban waterbodies in the Klang Valley, Malaysia were investigated along trophic and urbanization gradients. Zooplankton and water quality samples were collected three times, between May and November 2017, from two different locations in each lake. All three main zooplankton groups (rotifera, copepoda, and cladocera) were recorded from the study areas throughout the sampling period. The zooplankton community structure, particularly with regard to rotifers and cladocerans, varied between lakes and seasons. Zooplankton diversity does not vary with lake size or distance from the city center but does vary with shoreline development index and urbanization impacts. The zooplankton populations were dominated by rotifers, mainly Brachionus angularis at all study sites during the study period followed by copepods and cladocerans. The total density of zooplankton was significantly highest (p < 0.05) in the hypereutrophic lakes and during the dry season. Zooplankton diversity and rotifer species richness were negatively correlated with total phosphorus (TP). Diversity increased with urbanization and shoreline development, with rotifers as a potential bioindicator of trophic state in urban tropical lakes, due to their close relationship with TP.
Similar content being viewed by others
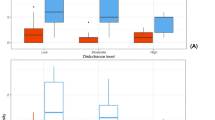
Introduction
Lake ecosystems generally have three trophic components, namely, phytoplankton as the primary producers, zooplankton, and higher trophic consumers such as fishes (Wetzel 2001). Zooplankton communities play an important role in the food web structure and in ecosystem health by feeding on phytoplankton, and they serve as food for higher trophic fishes and many carnivorous invertebrates. Their direct and rapid response to many physical, chemical, and biological changes in aquatic ecosystems means that zooplankton represents a useful indicator that could reflect the changes in aquatic ecosystems caused by pollution and various other disturbances (Marques et al. 2007). Different zooplankton groups may have different responses to eutrophication, due to differences in reproductive rates, filtering capacities (An et al. 2012), and food selection (Lodi et al. 2011). Water quality in many lakes is deteriorating due to anthropogenic effects, subsequently affecting zooplankton community structures, such as their composition and abundance, due to their sensitivity to environmental changes. Ban et al. (2013) reported that zooplankton community structure varies with chlorophyll a concentration, temperature, and different higher trophic species, such as fish communities, which prey on zooplankton. Mimouni et al. (2018) showed a stronger relationship between zooplankton species richness and macrophyte cover in urban waterbodies: a higher macrophyte cover provides a higher diversity of habitats for zooplankton communities.
The majority of lakes and ponds situated in urbanized and highly populated areas tend to receive municipal wastewater discharges, failing septic systems, and sewage overflows, inducing rapid deterioration of water quality and eutrophication (Naselli-Flores 2008). Higher nutrient loads lead to a higher trophic state in urban lakes, as their smaller and shallower morphology (Birch and McCaskie 1999) makes them more sensitive to water pollution and eutrophication. Studies show that high levels of urbanization reduce aquatic biodiversity in urban lakes due to the replacement of natural habitat by roads and buildings (Hassall and Anderson 2015). Urbanization levels decrease cladoceran abundance and zoobenthos diversity and increase dominant species in urban streams and waterbodies (Oruso 2001). In contrast to natural lakes, urban ponds had a concrete margin, a synthetic base, reduced vegetation cover, and lower connectivity to other waterbodies and are subject to run-off from residential and industrial development which can greatly increase the pollution levels and eutrophication (Hassall 2014). A cascading effect on different trophic levels, especially in zooplankton communities (Lodi et al. 2011) during eutrophication may increase the density of cyanobacteria and microalgae, which are inedible and toxic to zooplankton (Paerl and Otten 2013). Thus, the dominance of highly pollutant-tolerant species and a decrease in zooplankton diversity can be expected in highly eutrophic waters (Ger et al. 2014). The marked dominance of Brachionus forficula, Brachionus nilsoni, and Trichocerca sp. suggests that these zooplankton species are good indicators of eutrophic conditions in waterbodies Ismail and Adnan (2016).
Urban lakes and ex-mining ponds are frequently used for flood retention, and their intrinsic aesthetic value is an attractive factor for recreational purposes (Naselli-Flores 2008; Hassall 2014; Hassall and Anderson 2015), besides providing habitat for many aquatic organisms including zooplankton (Hassall 2014). To the best of our knowledge, despite the importance of urban lakes for containing water and cushioning floods, especially in a densely populated area such as the Klang Valley in Malaysia, few studies have been carried out on the habitat, and particularly on the zooplankton structure, of urban tropical waterbodies. Such waterbodies, located in urbanized settings, are severely threatened by rapid development and eutrophication. Nevertheless, these waterbodies are becoming hotspots for recreation and fishing activities. In this study, we investigate the variation of zooplankton community structures in urban lakes and ponds along trophic and urbanization gradients in Kuala Lumpur and Selangor, Malaysia. This study on the taxonomy and structure of zooplankton populations will support management efforts in developing a basis for sustainable water protection and fishery resources development in urban waterbodies.
Materials and methods
Study area
The Klang Valley is the most populated area in Malaysia (greater than six million inhabitants) and has experienced rapid urbanization over the last two centuries. This urbanization process has an impact on surrounding waterbodies created for flood retention and on ex-mining pools. A total of 11 waterbodies, ranging in size from 6.6 to 65 ha, were selected for this study (Table 1). The waterbodies were selected based on different densities of development (urbanization status), i.e., high-density, medium-density, or low-density development. The waterbodies’ distances from the city center of Kuala Lumpur ranged from 2.3 to 23.2 km. Five waterbodies were used as urban gardens and recreational sites, while the rest were used solely for flood retention.
Zooplankton sampling and enumeration
Field measurements were carried out in 2017, and samples were collected three times between May and November. Zooplankton samples were collected vertically using a zooplankton net (mesh size: 60 μm). The collected zooplankton samples were preserved in 5% buffered formalin (final concentration) and kept in 500-ml plastic bottles prior to subsequent analysis. All samples were transported to the laboratory for taxonomic identification and enumeration. A sample of 1–5 ml (depending on the zooplankton density, i.e., where no fewer than 150 individuals of the dominant species were counted) was placed in a Sedgewick Rafter counting chamber and left to settle for half an hour prior to transfer to the microscope stage (Nikon Eclipse E400, Nikon, Japan). Identification of zooplankton was based on descriptions, a taxonomic key and illustrations by Phan et al. (2015), Segers (2008), and Sa-Ardrit et al. (2013). Random non-overlapping fields were examined until at least 150 individuals of the dominant species were counted and reported as a number of individuals per liter. The density of zooplankton was calculated according to Lee and McAlice (1979).
Environmental variables
Water samples were collected at surface level (about 0.5 m) or mid-depth (for shallower sites below 1 m) using a 2.2-L Van Dorn sampler. Samples were analyzed for total phosphorus (TP) using a DR2800 spectrophotometer. The lake size, shore length, and distance to the city center were measured using Google Earth Pro. The urbanization gradient is measured using surface area, shore length, shoreline development index, pond distance from the city center (CCD), and the urban impact assessment (UIA) scale. The shoreline development index (SDI) was calculated based on the waterbody’s surface area and shoreline length following Osgood (2005) and categorized as small (< 8 ha), medium (8–17 ha), or large (> 17 ha). The urban impact assessment scale (UIA) was subjectively estimated based on the three-scale approach used by Pawlikiewicz and Jurasz (2017): 1 = completely urbanized area with high-density development, 2 = significantly urbanized area with medium-density development, and 3 = area with a low degree of urbanization or low-density development. Due to the close proximity of some ponds, we limited urbanization impacts to those originating within a 1-km radius surrounding the ponds. The distance from the city center of Kuala Lumpur was categorized into three groups: 1 (< 6 km), 2 (6–10 km), and 3 (> 10 km).
Data analysis
Biodiversity indices were calculated for each sample: the number of species, the Shannon-Wiener diversity index, Margalef species richness index, and Pielou’s evenness index. Species abundance data were transformed via square roots to balance the contributions from the few very abundant species with the many rare species (Clarke and Warwick 1994), while TP values were log-transformed to improve normality. All values were pooled into mean values for each waterbody to analyze the zooplankton structure against TP and urbanization gradient. The effects of the urbanization gradient on zooplankton density and diversity were tested using the non-parametric Kruskal-Wallis test and pairwise comparison.
Cluster analysis and non-dimensional metric scaling (NMDS) were employed to visualize multivariate patterns of the zooplankton abundance. The SIMPER (similarity percentage) analysis was used to determine the contribution of each rotifer species to the average similarity within the site defined. Non-parametric Spearman’s rank-order correlation analyses were conducted on the environmental parameters and rotifer communities, to determine whether any significant relationships existed among the parameters. Seasons were categorized into dry (May–September) and wet (October–December) for t test analysis. A Mann-Whitney U test was carried out to test the significant differences between dry and wet seasons. All multivariate analyses were performed using the PRIMER software package, v. 6.1.9 (PRIMER-E Ltd.), while correlations, non-parametric tests, and t tests were conducted using SPSS v. 22 (IBM SPSS Statistical, Chicago, USA).
Results
Trophic state conditions
Total phosphorus (TP) concentration was significantly highest (p < 0.05) in Selayang Pond throughout the study period, followed by Intan Baiduri Lake, Danau Kota Pond, and Jinjang Pond. The TP concentration was significantly lowest (p < 0.05) in Titiwangsa Lake. The TP concentrations in the remaining lakes and ponds ranged from 7.6 × 10−7 to 59 × 10−7 μM (Fig. 1). The Carlson Trophic State Index (CTSI) based on TP showed that all the studied ponds were categorized as eutrophic to hypereutrophic. Selayang Pond had the highest CTSI value (> 100) followed by Intan Baiduri Lake and Danau Kota Pond. The remaining ponds had CTSI values < 100 with the lowest value in Titiwangsa Lake (58.2).
Spatial and temporal zooplankton abundance and diversity
A total of 45 zooplankton taxa were recorded in the study areas throughout the sampling period comprising 35 species of rotifer, five species of copepoda (including all developmental stages, i.e., nauplii, copepodites, and adults), three species of cladocera, and one species of ostracoda (see supporting document for a list of species and their abundances). Rotifera were highly dominant (> 98.5%) in Jinjang Pond, Danau Kota Pond, and Intan Baiduri Lake, followed by Biru Kundang and Metropolitan Lakes (> 80%), Titiwangsa Lake (76.2%), and Selayang Pond (60.2%). Meanwhile, Desa Park Pond and Sri Johor Pond were dominated by copepoda with values of 61.4% and 60.6%, respectively. Cladocera contributed less than 10% of the total zooplankton in all study sites while ostracoda were found only in Biru Kundang Lake.
Among the study areas, the mean density of total zooplankton was significantly highest (p < 0.05) in Selayang Pond (Fig. 2), followed by Biru Kundang, Intan Baiduri, Danau Kota, Jinjang, Metropolitan, and Titiwangsa lakes. The zooplankton densities of the remaining lakes and ponds were less than 100.0 ind. L−1. In Selayang, Intan Baiduri, and Danau Kota Ponds, total zooplankton density was recorded as highest in July 2017 (dry season) and lowest in October 2017 (wet season) (Fig. 3). Meanwhile, a high total zooplankton density was recorded in Biru Kundang Lake in May 2017 with a total of 1409.7 ind. L−1. A Mann-Whitney U test analysis showed that overall zooplankton densities were significantly higher (p < 0.05) in the dry season (411.43 ± 162.04 ind. L−1) than in the wet season (86.98 ± 27.27 ind. L−1) (Fig. 4). The highest zooplankton diversity and species evenness was recorded in Titiwangsa Lake and Metropolitan Lake, while the lowest diversity and species evenness was recorded in Jinjang Pond. The NMDS plot showed five distinct clusters at 50% similarity (Fig. 5). Both hypereutrophic waterbodies, Intan Baiduri Lake, and Selayang Pond are clustered with 65% similarity. Batu Pond, a flood retention pond, had the highest zooplankton richness followed by Desa Park Pond and Permaisuri Lake.
NMDS plot of zooplankton abundance, with superimposed circles of increasing TP concentration. Numbers: 1 - Permaisuri, 2 - Intan Baiduri, 3 - Metropolitan, 4 - Biru Kundang, 5 - Titiwangsa, 6 - Batu, 7 - Desa Park, 8 - Jinjang, 9 - Sri Johor, 10 - Danau Kota, 11 - Selayang. Solid line: 45% similarity; dashed line: 50% similarity; dotted line: 65% similarity
The zooplankton populations were mostly dominated by rotifers at all study sites (Table 2). Permaisuri Lake, Batu Pond, and Desa Park Pond had high species richness. A decreasing trend of rotifer diversity was found during the wet season for Permaisuri Lake and Sri Johor Pond, while an increasing trend was observed for Intan Baiduri and Biru Kundang Lakes (Fig. 6).
Effects of urbanization and nutrient gradient on zooplankton abundance and diversity
In terms of urbanization parameters, no relationship was observed between zooplankton density or diversity and lake morphometry, i.e., surface area and shore length (p > 0.05). Only species diversity showed significant differences with SDI (p < 0.05) (Fig. 7). Batu and Jinjang ponds had the highest SDI while Permaisuri Lake and Danau Kota Pond had the lowest SDI. The cladoceran density was significantly related to UIA (p < 0.05). Higher cladoceran densities were recorded in ponds located in low-density development areas such as Biru Kundang and Permaisuri Lakes. No relationship was found between zooplankton density or diversity and distance from the city center. Zooplankton diversity and rotifer species richness were negatively correlated with TP (p < 0.05). Furthermore, rotifer species evenness and diversity indices were positively correlated with shore length, with r = 0.51 and r = 0.59, respectively (Table 3). This result is consistent with findings by Lodi et al. (2011), showing that the total density and evenness of rotifer communities were the best correlates of eutrophication and that zooplankton density increased with eutrophication level.
Bioindicator species
SIMPER analyses based on community estimates at different lakes and ponds identified the discriminator species primarily accounting for the assemblage differences between the various lakes and ponds. Table 4 shows the two species with the highest percentage contribution in all the lakes and ponds. SIMPER analysis showed that Brachionus angularis was the major contributor species, in the following waterbody order: Danau Kota > Selayang > Sri Johor > Intan Baiduri > Jinjang > Desa Park > Batu. In addition, Hexarthra mira and Polyarthra vulgaris contributed high percentages in Permaisuri Lake (65.2%) and Metropolitan Lake (31.5%), respectively. Keratella cochlearis contributed fairly high percentages in Biru Kundang Lake (24.2%) and Titiwangsa Lake (20.3%).
Discussion
Spatial and temporal variation of zooplankton communities
The present study illustrates the varying zooplankton structure in urban tropical lakes in Malaysia. Nutrient levels, and zooplankton diversity and species evenness varied between lakes. Significant differences in zooplankton densities were also found among lakes. A high density of zooplankton was observed in highly eutrophic ponds. Rotifer was dominant in Jinjang, Intan Baiduri, and Danau Kota ponds throughout the study. In contrast, copepod was more dominant in Permaisuri, Desa Park, and Sri Johor ponds. Organic and inorganic matter from the anthropogenic activities draining into the pond increased the nutrient and water turbidity levels (Dodson et al. 2007). A high turbidity level, due to elevated suspended sediments, limits the visual distance of planktivorous species, which may lead to a declining foraging rate, allowing increased survival of the zooplankton community and therefore an increase in the zooplankton density (Wetzel 2001). This study found that a higher density of zooplankton was observed during the dry season compared to the wet season. A similar finding was obtained by Shukla and Singh (2013), who studied the seasonal plankton population in Maheshara Lake, India. High zooplankton density during the dry season is probably due to the high photosynthetic activity (Shukla and Singh 2013) that provides the food for zooplankton, as well as to favorable environmental conditions (Manickam et al. 2018). High seasonal variation of zooplankton communities, including rotifers, was also observed by Umi et al. (2018) and Sharip et al. (2017): a higher density of zooplankton was found in three different reservoirs, between June and October, coinciding with the dry months.
The highest diversity of zooplankton, found in Titiwangsa Lake, maybe related to the presence of macrophytes. High submerged macrophyte cover was qualitatively observed in this waterbody during the field sampling. A strong positive relationship between zooplankton diversity and richness and macrophyte abundance has been shown in a few studies in other temporary and permanent ponds including stormwater detention structures (Mimouni et al. 2018; Sun et al. 2019). Possible macrophyte effects that could drive zooplankton species richness include ecological gradient such as food availability and low predator visibility (Mimouni et al. 2018).
Urbanization and nutrient gradient on zooplankton abundance and diversity
Among the urbanization parameters, urbanization impacts have greater influence in shaping diversity. Distance from the city center has no significant relation to the variation of zooplankton structure, probably due to localized urban effects as the waterbodies are drained by different river tributaries. Even waterbody within the same river basin, such as Jinjang Lake, Intan Baiduri Lake, Selayang Pond, and Metropolitan Lake, which are all drained by the Jinjang River, exhibit different trophic and zooplankton community variations. Lakes that are surrounded by dense housing development and heavy industry (Jinjang, Selayang, and Intan Baiduri) are more polluted than lakes surrounded by low-density development and gardens (Titiwangsa, Metropolitan ,and Permaisuri). Direct discharges of waste water containing high TP from markets and stalls, and inadequate waste water treatment facilities have been reported as significant contributors of nutrients and other pollutants in urban environments (Sharip and Mohamad 2019). These factors impact on the trophic condition of waterbodies and subsequently their ecological quality. Dodson et al. (2007) reported that zooplankton richness and composition were indirectly associated with land use, including urbanization.
Among the zooplankton group, rotifers were the richest species group compared to copepods and cladocerans. They have been used as a bioindicator of lake-water quality status (Pontin 1978; Ejsmont-Karabin 2012; Oh et al. 2017) besides being deemed the most important zooplankton group in the freshwater food web, especially in lentic water (Lokko et al. 2017; Wen et al. 2017). In this study, a total of 37 rotifer species were recorded. Rotifer species found in the studied lakes were comparable with those found in freshwater localities in Malaysia (Meor Hussain et al. 2002; Ismail and Adnan 2016; Sharip et al. 2017). These findings were comparable with those of Wen et al. (2017) who found 47 rotifer species in their study in two urban lakes in China and higher than those of Manickam et al. (2018) who found nine rotifer species in their study of Ukkadam Lake in India.
In this study, copepods, dominated by copepod nauplii, were dominant in Desa Park and Sri Johor ponds. The dominance of copepod nauplii and copepodites was typical of eutrophic waters. This is probably due to the larger size of the adult stage, which increases the predation rate Sampaio et al. 2002. Additionally, it is also probably influenced by the feeding behavior of copepods nauplii, which are considered to be herbivorous, in contrast to the adult copepods which are mostly carnivorous. High densities of phytoplankton in eutrophic waters possibly provide enough food for the nauplii. Meanwhile, cyclopoids dominated the adult copepod assemblage in the studied ponds. Kumar and Rao (2001) also mentioned that cyclopoid predators are dominant in eutrophic waterbodies as they have wide range of prey species (ciliates, rotifers, and cladocerans). In addition, Pinto-Coelho and Bezerra-Neto (2005) showed that calanoids were good indicators for oligotrophic lakes. This study also showed a low density of calanoids in the study area. Cladocerans had the lowest abundances and were sometimes absent in the studied ponds. Higher cladoceran densities were found in less urbanized ponds (Permaisuri and Desa Park) consistently with the findings of Gianuca et al. (2018). Gianuca et al. (2018) showed that the dominance of cladoceran species was related to traits and phylogenetic metrics with large-bodied species becoming more dominant in less urbanized systems. Abrantes et al. (2006) showed that the presence of Cyanobacteria in eutrophic waters decreased the densities of cladocerans, especially Daphnia. The toxicity and filamentous characteristics of cyanobacteria decrease the grazing rate of cladocera and reduce their abundance. The cause of the occurrence of ostracods in Biru Kundang Lake was not known, due to limited information about environmental parameters. Rossetti et al. (2004) showed that total alkalinity and pH were the most important parameters structuring the ostracods species assemblages.
Previous studies suggest that individual rotifer occurrences and distributions are commonly influenced by trophic state (Duggan et al. 2001; Wen et al. 2011). In this study, rotifer assemblages were dominated by Brachionus. Brachionus has been reported as the most abundant rotifer in eutrophic lakes, since it has a high tolerance to pollutants (Tasevska et al. 2012). Furthermore, Ismail and Adnan (2016) stated that Brachionus is a better trophic indicator than crustaceans as the former are less affected by algal bloom. SIMPER analysis showed that B. angularis had a high contribution in seven of the 11 studied ponds. Similarly, Mola (2011) also found B. angularis to be the most dominant species in the eutrophic Lake El-Manzalah. Kumar and Rao (2001) showed that B. angularis was more successful that B. calyciflorus in competing for the same food source, given its smaller size. Likewise, in the hypereutrophic Sembrong Reservoir, Brachionus, Keratella, and Trichocerca were the most dominant genera recorded (Sharip et al. 2017; Umi et al. 2018). In addition, Brachionus and Keratella cochlearis have also been regularly reported as dominant in eutrophic lakes (Špoljar et al. 2011; Ejsmont-Karabin 2012). In this study, Keratella cochlearis also had a high contribution in the studied ponds.
The present data displayed no significant correlation between crustacean zooplankton species richness and TP, although species composition was correlated with environmental gradients consistent with findings by Dodson et al. (2009). A study by Pawlikiewicz and Jurasz (2017) showed that the Shannon diversity index increases with the size of a water body. Mimouni et al. (2015) also identified waterbody size as one of the important local factors shaping variation in zooplankton community composition. In this study, such a linear relationship was not observed, but diversity did increase with the shoreline development index. Earlier, it was found that TP concentrations may not exhibit a linear relationship with an increase in urbanization or development in natural tropical lakes and urban ponds (Sharip et al. 2014), which may support the present data.
Urban freshwater lakes and ponds are vulnerable to anthropogenic activities including nutrient pollution. TP was used as an independent variable to show trophic state in this study. Pinto-Coelho and Bezerra-Neto (2005) showed that TP is a good descriptive variable for eutrophication in lakes and reservoirs. Carlson’s TSI was used in this study for the trophic classification of lakes, due to its simplicity, its wide usage and the fact that fewer parameters are needed (Carlson 1977). CTSI values showed that all lakes and ponds were contaminated with high TP concentrations and categorized as eutrophic. Cluster and NMDS analysis showed that the zooplankton abundances and community structures are associated with TP levels. Lake eutrophication is a worldwide phenomenon, and Malaysia is no exception. An earlier study on lake eutrophication in Malaysia showed that 62% of the 90 lakes studied were identified as eutrophic (Sharip and Yusop 2007). The earlier study, however, did not include the urban ponds studied here. In this work, all the studied urban ponds were either eutrophic or hypereutrophic, with Selayang Pond having the highest TP concentration. The pond is highly eutrophic, probably due to the direct discharge of waste water containing high nutrient concentrations from the wet market which is located close to the pond (Jais et al. 2017). Furthermore, correlation analysis showed that surface area and shore length had no influence on the TP concentration. The eutrophication is mainly a result of anthropogenic activities.
The present study showed that zooplankton density was positively correlated with TP. However, increased zooplankton density as a reaction to eutrophication only can be seen for some species. In eutrophic lakes, tolerance mechanisms towards cyanobacteria are important for zooplankton survival. Work and Havens (2003) showed that zooplankton consume heterotrophic bacteria and cyanobacteria when the density of chlorophytes and diatoms is low. However, some species have low tolerance to environmental stress. This study also showed that species richness was negatively correlated with TP. High TP concentration is a probable reason for the decreasing species richness, as some rotifer communities have been shown to respond quickly to a wide range of environmental stresses (Wen et al. 2017). Ger et al. (2014) also showed that the dominance of high pollutant-tolerant species and a decrease in zooplankton diversity are expected in highly eutrophic waters. Furthermore, a sharp decline in the biomass of the cladoceran group with an increase in TP was reported by Pinto-Coelho and Bezerra-Neto (2005). Sun et al. (2019) showed that urban ponds that function as urban drainage systems or stormwater detention structures not only act as pollutant trap but also provide suitable habitats for pollution-tolerant taxa.
The close relationship between zooplankton diversity and TP indicates that zooplankton diversity has potential as a bioindicator of lake trophic state in tropical urban lakes. This study did not assess the effects of fishes on the zooplankton community via predation or the influence of algae assemblages as food for the zooplankton, which may affect the variability of zooplankton diversity and density. Further work should be carried out to investigate the role of fishes, algal, and the overall food web in urban tropical waterbodies.
Conclusions
This study observed the spatial and temporal zooplankton structure and diversity in urban tropical lakes. Zooplankton density was positively correlated with TP, while rotifer species richness was negatively correlated with TP. Species diversity was significantly related to shoreline development index and urbanization impacts, rather than lake morphometry. Increased urbanization and shoreline development may decrease the zooplankton density and diversity and the overall ecosystem health. Rotifers, dominated by Brachionus angularis, were closely related to TP and may therefore provide a potential bioindicator of the trophic state in urban tropical lakes. Current knowledge about the impacts of urbanization and eutrophication on zooplankton communities in urban tropical waterbodies is limited. As urbanization increases in developing nations, this finding provides basic information about the zooplankton structure, diversity, and distribution in waterbodies that are created or modified as recreational sites for city dwellers, for further monitoring and assessment of urban ecosystems.
Availability of data and materials
Please contact the corresponding author for data requests.
Abbreviations
- CCD:
-
City center
- CTSI:
-
Carlson Trophic State Index
- NMDS:
-
Non-dimensional metric scaling
- SDI:
-
Shoreline development index
- TP:
-
Total phosphorus
- UIA:
-
Urban impact assessment
References
Abrantes N, Antunes SC, Pereira MJ, Gonçalves F (2006) Seasonal succession of cladocerans and phytoplankton and their interactions in a shallow eutrophic lake (Lake Vela, Portugal). Acta Oecol 29:54–64
An XP, Du ZH, Zhang JH, Li YP, Qi JW (2012) Structure of the zooplankton community in Hulun Lake, China. Procedia Environ Sci 13:1099–1109
Ban S, Makino W, Sakano H, Haruna H, Ueda H (2013) Annual variation in biomass and the community structure of crustacean zooplankton over 5 years in Lake Toya, Japan. Limnology 14:59–70. https://doi.org/10.1007/s10201-012-0387-3
Birch S, McCaskie J (1999) Shallow urban lakes: a challenge for lake management. Hydrobiologia 395(365):365–377. https://doi.org/10.1023/A:1017099030774
Carlson RE (1977) A trophic state index of lakes. Limnol Oceanogr 22:361–368
Clarke KR, Warwick RM (1994) Change in marine communities: an approach to statistical analysis and interpretation. Natural Environment Research Council, Plymouth
Dodson S, Everhart W, Jandl A, Krauskopf S (2007) Effect of watershed land use and lake age on zooplankton species richness. Hydrobiologia 579:393–399
Dodson SI, Newman AL, Will-Wolf S, Alexander ML, Woodford WP, Van Egeren S (2009) The relationship between zooplankton community structure and lake characteristics in temperate lakes (northern Wisconsin, USA). J Plankton Res 31:93–100
Duggan IC, Green JD, Shiel RJ (2001) Distribution of rotifers in North Island, New Zealand, and their potential use as bioindicators of lake trophic state. Hydrobiologia 446:155–164
Ejsmont-Karabin J (2012) The usefulness of zooplankton as lake ecosystem indicators: rotifer trophic state index. Polish J Ecol 60:339–350
Ger KA, Hansson LA, Lurling M (2014) Understanding cyanobacteria-zooplankton interactions in a more eutrophic world. Freshw Biol 59:1783–1798
Gianuca AT, Engelen J, Brans KI, Hanashiro FTT, Vanhamel M, van den Berg EM, Souffreau C, Meester LD (2018) Taxonomic, functional and phylogenetic metacommunity ecology of cladoceran zooplankton along urbanization gradients. Ecography 41:183–194. https://doi.org/10.1111/ecog.02926
Hassall C (2014) The ecology and biodiversity of urban ponds. Wiley Interdiscip Rev Water 1:187–206
Hassall C, Anderson S (2015) Storm water ponds can contain comparable biodiversity to unmanaged wetlands in urban areas. Hydrobiologia 745:137–149
Ismail AH, Adnan AAM (2016) Zooplankton composition and abundance as indicators of eutrophication in two small man-made lakes. Tropical Life Sci Res 27:31–38
Jais NM, Mohamed RMSR, Al-Gheethi AA, Hashim MA (2017) The dual roles of phycoremediation of wet market wastewater for nutrients and heavy metals removal and microalgae biomass production. Clean Tech Environ Policy 19:37–52
Kumar R, Rao TR (2001) Effect of the cyclopoid copepod Mesocyclops thermocyclopoides on the interactions between the predatory rotifer Asplanchna intermedia and its prey Brachionus calyciflorus and B. angularis. Hydrobiologia 453:261–268
Lee WY, McAlice BJ (1979) Sampling variability of marine zooplankton in a tidal estuary. Estuary Coastal Mar Sci 8:565–582
Lodi S, Vieira LCG, Velho LFM, Bonecker CC, de Carvalho P, Bini LM (2011) Zooplankton community metrics as indicators of eutrophication in urban lakes. Natureza & Conservação 9:87–92
Lokko K, Virro T, Kotta J (2017) Seasonal variability in the structure and functional diversity of psammic rotifer communities: role of environmental parameters. Hydrobiologia 796:287–307. https://doi.org/10.1007/s10750-016-2923-3
Manickam N, Bhavan PS, Santhanam P, Bhuvaneswari R, Muralisankar T, Srinivasan V, Asaikkutti A, Rajkumar G, Udayasuriyan R, Karthik M (2018) Impact of seasonal changes in zooplankton biodiversity in Ukkadam Lake, Coimbatore, Tamil Nadu, India, and potential future implications of climate change. J Basic Appl Zool 79:15
Marques SC, Pardal MA, Pereira MJ, Gonçalves F, Marques JC, Azeiteiro UM (2007) Zooplankton distribution and dynamics in a temperate shallow estuary. Hydrobiologia 587:213–223. https://doi.org/10.1007/s10750-007-0682-x
Meor Hussain MAF, Ahyaudin A, Shah AR, Shah M (2002) The structure and dynamics of net-zooplankton communities of the littoral versus limnetic zone of a typical embayment in a small flow through tropical reservoir. J Biosci 13:23–34
Mimouni E-A, Pinel-Alloul B, Beisner BE (2015) Assessing aquatic biodiversity of zooplankton communities in an urban landscape. Urban Ecosyst 18:1353–1372. https://doi.org/10.1007/s11252-015-0457-5
Mimouni E-A, Pinel-Alloul B, Beisner BE, Legendre P (2018) Summer assessment of zooplankton biodiversity and environmental control in urban waterbodies on the Island of Montreal. Ecosphere 9:e02277. https://doi.org/10.1002/ecs2.2277
Mola HR (2011) Seasonal and spatial distribution of Brachionus (Pallas, 1966; Eurotatoria: Monogonanta: Brachionidae), a bioindicator of eutrophication in lake El-Manzalah, Egypt. Egypt Biol Medi 3:60–69
Naselli-Flores L (2008) Urban lakes: ecosystems at risk, worthy of the best care. In: Proceedings of Taal 2007: The 12th World Lake Conference, p 1337
Oh HJ, Jeong HG, Nam GS, Oda Y, Dai W, Lee EH, Chang KH (2017) Comparison of taxon-based and trophi-based response patterns of rotifer community to water quality: applicability of the rotifer functional group as an indicator of water quality. Animal Cells System 21:133–140
Oruso RT (2001) Effects of urbanization on benthic macroinvertebrate communities in streams, Anchorage, Alaska. In: Survey USG (ed) Water-Resources Investigations Report 014278
Osgood RA (2005) Shoreline density. Lake Reserv Manage 21:125–126
Paerl HW, Otten TG (2013) Harmful cyanobacterial blooms: causes, consequences, and controls. Microbial Ecol 65:995–1010. https://doi.org/10.1007/s00248-012-0159-y
Pawlikiewicz P, Jurasz W (2017) Ecological drivers of Cladoceran diversity in the central European City (Lodz, Poland): effects of urbanisation and size of the waterbody. Ann Zool Fenn 54:315–333
Phan DD, Nguyen VK, Le TN, Dang NT, Ho TH (2015) Identification handbook of freshwater zooplankton of the Mekong River and its tributaries. Mekong River Commission, Vientiane
Pinto-Coelho RM, Bezerra-Neto JF (2005) Effects of eutrophication on size and biomass of crustacean zooplankton in a tropical reservoir. Brazilian J Biol 65:325–338
Pontin R (1978) A key to the freshwater planktonic and semi-planktonic Rotifera of the British Isles. Scientific Publication No. 38. Freshwater Biological Association, Ambleside
Rossetti G, Bartoli M, Martens K (2004) Limnological characteristics and recent ostracods (Crustacea, Ostracoda) of freshwater wetlands in the Parco Oglio Sud (northern Italy). Int J Limnol 40:329–341
Sa-Ardrit P, Pholpunthin P, Segers H (2013) A checklist of the freshwater rotifer fauna of Thailand (Rotifera, Monogononta, Bdelloidea). J Limnol 72:361–375
Sampaio EV, Rocha O, Matsumura-Tundisi T, Tundisi JG (2002) Composition and abundance of zooplankton in the limnetic zone of seven reservoirs of the Paranapanema River, Brazil. Brazilian J Biol 62:525–545
Segers H (2008) Global diversity of rotifers (Rotifera) in freshwater. Hydrobiologia 595:49–59
Sharip Z, Ahmad-Dini UW, Yusoff FM (2017) A plankton community study in Sembrong and Subang reservoirs. In: Sharip Z, Yusoff FM, Ismail WR, Suratman S, Mohamed A (eds) A survey on water quality and some biological communities in selected lakes of Malaysia. NAHRIM, Seri Kembangan, p 33
Sharip Z, Mohamad MF (2019) Microbial contamination in urban tropical lentic waterbodies and ponds along urbanisation gradient. J Tropical Agri Sci 42:165–184
Sharip Z, Yusop Z (2007) National overview: the status of lakes eutrophication in Malaysia. In: Anton A, Zakaria S, Yusoff FM, Jin L, Sim LK, Sharip Z (eds) Proceedings of the colloquium on lakes and reservoir management: status and issues. NAHRIM, Seri Kembangan, p 24
Sharip Z, Zaki ATA, Shapiai MAH, Suratman S, Shaaban AJ (2014) Lakes of Malaysia: water quality, eutrophication and management. Lakes Reserv Res Manage 19:130–141. https://doi.org/10.1111/lre.12059
Shukla PP, Singh A (2013) A seasonal variations of plankton population of Maheshara Lake in Gorakhpur, India. World J Zool 8:9–16
Špoljar M, Tomljanovic T, Lalic I (2011) Eutrophication impact on zooplankton community: a shallow lake approach. Holistic Appr Environ 1:131–142
Sun Z, Sokolova E, Brittain JE, Saltveit SJ, Rauch S, Meland S (2019) Impact of environmental factors on aquatic biodiversity in roadside stormwater ponds. Sci Rep 9:5994. https://doi.org/10.1038/s41598-019-42497-z
Tasevska O, Guseska D, Kostoski G (2012) Comparison of pelagic rotifer communities in three natural Macedonian lakes. Acta Zool Bulg Suppl 4:159–165
Umi WAD, Yusoff FM, Aris AZ, Sharip Z (2018) Rotifer community structure in tropical lakes with different environmental characteristics related to ecosystem health. J Environ Biol 39:795–807. https://doi.org/10.22438/jeb/39/5(SI)/30
Wen X, Zhai P, Feng R, Yang R, Xi Y (2017) Comparative analysis of the spatio-temporal dynamics of rotifer community structure based on taxonomic indices and functional groups in two subtropical lakes. Sci Rep 7:578
Wen XL, Xi YL, Qian FP, Zhang G, Xiang XL (2011) Comparative analysis of rotifer community structure in five subtropical shallow lakes in East China: role of physical and chemical conditions. Hydrobiologia 661:303–316
Wetzel RG (2001) Limnology: lake and river ecosystems. Academic Press, San Diego
Work KA, Havens KE (2003) Zooplankton grazing on bacteria and cyanobacteria in a eutrophic lake. J Plankton Res 25:1301–1306
Acknowledgements
Thanks to M. Hafiz Zulkifli for assistance with the water sample collections and M. Zaki M. Amin and Lee Hin Lee for their management support.
Funding
Financial assistance for this study was supported by the Ministry of Water, Land and Natural Resources Malaysia (Vote Number: P23170000190001).
Author information
Authors and Affiliations
Contributions
SBAR and ZS designed and conducted the field research, analyzed the data, and interpreted the results and contributed in writing the manuscript. Both authors read and approved the final manuscript.
Corresponding author
Ethics declarations
Ethics approval and consent to participate
Not applicable in this section.
Consent for publication
Not applicable in this section.
Competing interests
The authors declare that they have no competing interests.
Additional information
Publisher’s Note
Springer Nature remains neutral with regard to jurisdictional claims in published maps and institutional affiliations.
Rights and permissions
Open Access This article is distributed under the terms of the Creative Commons Attribution 4.0 International License (http://creativecommons.org/licenses/by/4.0/), which permits unrestricted use, distribution, and reproduction in any medium, provided you give appropriate credit to the original author(s) and the source, provide a link to the Creative Commons license, and indicate if changes were made.
About this article
Cite this article
Abd. Razak, S.B., Sharip, Z. Spatio-temporal variation of zooplankton community structure in tropical urban waterbodies along trophic and urban gradients. Ecol Process 8, 44 (2019). https://doi.org/10.1186/s13717-019-0196-2
Received:
Accepted:
Published:
DOI: https://doi.org/10.1186/s13717-019-0196-2