Abstract
Background
Head louse, Pediculus humanus capitis, is an obligatory blood-sucking ectoparasite, distributed worldwide. Phylogenetically, it occurs in five divergent mitochondrial clades (A–E); each exhibiting a particular geographical distribution. Recent studies suggest that, as in the case of body louse, head louse could be a disease vector. We aimed to study the genetic diversity of head lice collected in the Democratic Republic of the Congo (DR Congo) and to screen for louse-borne pathogens in these lice.
Methods
A total of 181 head lice were collected from 27 individuals at the Monkole Hospital Center located in Kinshasa. All head lice were genotyped and screened for the presence of louse-borne bacteria using molecular methods. We searched for Bartonella quintana, Borrelia recurrentis, Rickettsia prowazekii, Anaplasma spp., Yersinia pestis, Coxiella burnetii and Acinetobacter spp.
Results
Among these head lice, 67.4% (122/181) belonged to clade A and 24.3% (44/181) belonged to clade D. Additionally, for the first time in this area, we found clade E in 8.3% (15/181) of tested lice, from two infested individuals. Dual infestation with clades A and D was observed for 44.4% individuals. Thirty-three of the 181 head lice were infected only by different bacterial species of the genus Acinetobacter. Overall, 16 out of 27 individuals were infested (59.3%). Six Acinetobacter species were detected including Acinetobacter baumannii (8.3%), Acinetobacter johnsonii (1.7%), Acinetobacter soli (1.7%), Acinetobacter pittii (1.7%), Acinetobacter guillouiae (1.1%), as well as a new potential species named “Candidatus Acinetobacter pediculi”.
Conclusions
To our knowledge, this study reports for the first time, the presence of clade E head lice in DR Congo. This study is also the first to report the presence of Acinetobacter species DNAs in human head lice in DR Congo.
Similar content being viewed by others
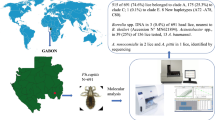
Background
Two lice species infested humans: Pediculus humanus and Pthirus pubis [1]. The first is of great public health concern and includes two ecotypes: Pediculus humanus capitis head lice, which live in the scalp area, and Pediculus humanus humanus body lice, which live in clothing [1, 2].
Studies based on mitochondrial genes appear to separate head and body lice into five divergent clades (A, B, C, D and E) exhibiting some geographical differences [3,4,5]. Head lice encompass all diversity while body lice belong only to clades A and D [3, 6]. It is well known that only clade A has a worldwide distribution [1]. However, with the globalization, clades B to E tend to disperse throughout the world. Originally, Clade B was found in Europe and in the New World [3], Clade C in Africa and Asia [7], Clade D in the Democratic Republic of Congo (DR Congo) [5], and Clade E in West Africa (Mali) [6]. Based on archeological remains, the Pediculus louse is thought to be an ancient parasite that had long association with their human hosts [8]. Because of this long association lice have become a model for studying the cophylogenetic relationships between hosts and parasites [9].
Although it is currently assumed that body lice are more potent vectors of pathogens, the potential role of head lice as a vector is not fully understood. Studies have shown that the immune responses of head lice to different pathogens are stronger than those of body lice, which obviously can carry a broad spectrum of pathogens [10, 11]. In recent decades, the DNA of several pathogenic bacteria has been increasingly detected in head lice collected around the world. This is the case of Bartonella quintana, Borrelia recurrentis, Yersinia pestis, Borrelia theileri, Coxiella burnetii, Rickettsia aeschlimannii, as well as of potential new species of the genera Anaplasma and Ehrlichia detected in head lice belonging to different mitochondrial clades [5, 6, 12,13,14,15,16,17]. Several species of Acinetobacter, including potential new species, have also been detected in human head lice [12, 18,19,20]. In addition, experimental infections with R. prowazekii have shown that head lice can be easily infected and spread these pathogens in their feces, demonstrating that these lice could have the potential to be vectors of pathogens under optimal epidemiological conditions [21]. In laboratory-reared lice, it has been shown that head lice can support a persistent load of B. quintana infection for several days following acquisition in a blood meal and disseminate viable organisms in their feces [10, 11]. This fact poses a very substantial health risk for infested persons because head lice infestations are widespread around the world and epidemics still occur regularly. Children are at increased risk, regardless of hygiene conditions and social status [22].
In this study, we aimed to investigate the genetic diversity of head lice collected in the DR Congo and to look for pathogenic bacteria in these lice.
Methods
Lice collection
Lice collection was carried out at the medical center of Monkole located at Kinshasa, the largest city and capital of the DR Congo. In total, 27 patients were enrolled and thoroughly examined for detection of both body and head lice. They came from 11 geographically very close communities. A total of 181 head lice were collected from these patients. No body lice were found during the examination. The lice collected were preserved in 70% alcohol and were then sent to the Laboratory of IHU-Méditerranée Infection, Marseille, France, stored at room temperature.
DNA extraction
To avoid bacterial contamination of lice external surface, each louse specimen was decontaminated, as described previously [23], and rinsed twice in distilled sterile water. Then, each louse was dried and cut in half lengthwise. Half was frozen at −20 °C for later use. The remaining half was crushed in sterile Eppendorf tube; total DNA was extracted using a DNA extraction kit, QIAamp Tissue Kit (Qiagen, Courtaboeuf, France) in the EZ1 apparatus following the manufacturer’s protocol. The DNA was eluted in 100 μl of TE (10/1) buffer and stored at 4 °C until used for PCR amplifications. DNA quantity and quality were assessed using a NanoDrop ND-1000 (Thermo Fisher Scientific, Waltham, MA, USA).
Genotypic status of lice
Identification of louse mitochondrial clade by qPCR assays
To identify the mitochondrial clades of the lice included in this study, all DNA samples were analyzed using clade-specific quantitative real-time PCR (qPCR) assays that targeted a portion of cytochrome b (cytb) gene specific to each of the five clades, as previously described [6]. We used lice with known clades as positive controls and master mixtures as negative control for each assay. All PCR amplifications were carried out using a CFX96 Real-Time system (Bio-Rad Laboratories, Foster City, CA, USA), as previously described [6].
Cytochrome b amplification and haplotype determination
For the phylogenetic study, DNA samples of 54 head lice randomly selected from the total number of lice, were subjected to standard PCR targeting a 347-bp fragment of the cytb gene, using the primers and conditions previously described [24]. The PCR consisted of a 50 µl volume, including 25 µl Amplitaq gold master mixes, 1 µl of each primer, 5 μl DNA template, and water. The thermal cycling profile was one incubation step at 95 °C for 15 min, 40 cycles of 1 min at 95 °C, 30 s at 56 °C and 1 min at 72 °C, followed by a final extension step for 5 min at 72 °C.
PCR amplification was performed in a Peltier PTC-200 model thermal cycler (MJ Research Inc, Watertown, MA, USA). The success of amplification was confirmed by electrophoresis on agarose gel. The purification of PCR products was performed using NucleoFast 96 PCR plates (Macherey-Nagel EURL, Hoerdt, France) according to the manufacturer’s instructions. The amplicons were sequenced using the Big Dye Terminator Cycle Sequencing Kit (Perkin Elmer Applied Biosystems, Foster City, CA) with an ABI automated sequencer (Applied Biosystems). The electrophoregrams obtained were assembled and edited using the ChromasPro software (ChromasPro 1.7, Technelysium Pty Ltd., Tewantin, Australia).
Molecular screening for the presence of bacterial DNA
The qPCRs were performed to screen all lice samples, using previously reported primers and probes for Borrelia spp., B. quintana, Acinetobacter spp., Rickettsia spp., R. prowazekii, Y. pestis, Anaplasma spp. and C. burnetii. Sequences of primers and probes are shown in Table 1 [6, 12, 13, 18, 24,25,26,27,28,29].
The qPCRs were performed using a CFX96 Real-Time system (Bio-Rad) and the Roche LightCycler 480 Probes Master Mix PCR kit (Roche Applied Science, Mannheim Germany). We included DNA extracts of the targeted bacteria as positive controls and master mixtures as negative control for each assay. We considered samples to be positive when the cycle’s threshold (Ct) was lower than 35 Ct [30].
In order to identify the species of Acinetobacter, all positive samples from qPCR were subjected to standard PCR, targeting a portion of the rpoB gene (zone 1) using primers and conditions previously described [25]. Successful amplification was confirmed via gel electrophoresis and amplicons were prepared and sequenced using similar methods as described for the cytb gene for lice above.
Data analysis
Unique haplotypes were defined by using DnaSPv5.10 to obtain head lice cytb sequences, then, compared and combined (Additional file 1: Table S1), with the cytb haplotypes previously reported [12]. In order to investigate the possible relationships between the haplotypes, the median-joining (MJ) network using the method of Bandelt was constructed with the program NETWORK4.6 (http://www.fluxus-engineering.com/sharenet.htm) using equal weights for all mutations [31]. Phylogenetic analyses and tree reconstruction were performed using MEGA software v.6.06 [32]. Phylogenetic analysis was performed using maximum likelihood (ML) approach. To generate the best ML tree, Modeltest v.3.7 [33] was used to examine model of nucleotide substitution and choose a best-fit model of sequence evolution. The model that provides the best approximation of the data using the fewest parameters was chosen for the analysis according to the Akaike information criterion [34, 35]. Tree reconstruction was conducted using MEGA software v.6.06 under HKY+I+G model with 500 bootstrap replicates. All obtained sequences of Acinetobacter spp. were analyzed using BLAST (www.ncbi.nlm.nih.gov/blast/Blast.cgi) and compared with sequences in the GenBank database. A maximum-likelihood method was used to infer the phylogenetic analyses and the best-fit model was chosen as described for cytb sequences above. The tree reconstruction was performed using the TrN+ G model for nucleotide sequences under 500 bootstrap replicates in MEGA software v.6.06 [32].
Results
Lice clade and phylogenetic analyses
Overall, 181 head lice were collected from 27 obviously healthy women. The average number of lice per individual was 6.7 ± 6.6. All collected lice were tested by qPCRs to determine their clade. Our results showed that 67.4% (122/181) of lice belonged to clade A, 24.3% (44/181) to clade D, and only 8.3% (15/181) belonged to clade E. Among the 27 persons, 15 (55.6%) were infested by only one clade. Among them, 8 (29.6%) individuals were only infested with clade A, 5 (18.5%) only with clade D, and 2 (7.4%) only with clade E. Finally, dual infestation was observed in 12 individuals (44.4%), and only with clades A and D (Table 2).
The analysis of a 347-bp fragment of 54 cytb sequences yielded 43 variable positions defining 10 different haplotypes. One haplotype belonged to the worldwide haplotype A5 within clade A. Four haplotypes also belonging to clade A, were new and were named A66-A69 (Table 3).
Within clade D, four haplotypes were identified; one of them belonged to haplotype D60. The other three haplotypes were novel and are referred to as D74-D76. The remaining haplotype belonging to clade E was novel and is referred to as E62 (Table 3). Analyses of the phylogenetic tree and the median assemblage yield similar results; all cytb sequences were distributed among five major supported clades, represented by five connected subnets and separate groups as shown by the MJ network corresponding to the known clades: A, D, B, C and E. The 10 haplotypes of our study fell into the three clades A, D and E (Figs. 1, 2). All sequences of cytb haplotypes of P. h. capitis obtained, in this study, were deposited in the GenBank database, under the accession numbers MH230921-MH230928.
Maximum-likelihood (ML) phylogram of the mitochondrial Cytb haplotypes. a Phylogenetic inference was conducted in MEGA 6 using the maximum likelihood method under HKY + I + G model with 500 bootstrap replicates. The novel haplotypes identified in this study are indicated in blue. b Bacterial DNAs detected in head lice reported in this study and the literature. The pathogenic bacteria in red are those naturally transmitted by body lice to humans
Cytb haplotype networks of human body and head lice. The five connected subnets corresponding to the known clades: A, D, B, C and E. Each circle indicates a unique haplotype, and variations in circle size are proportional to haplotype frequencies. Pie colors and sizes in circles represent the continents and the number of their sequence for a haplotype. The length of the links between nodes is proportional to the number of mutations. The types of haplotypes identified in this study are underlined
Molecular detection of bacterial DNA
All the head lice tested on qPCRs were negative for B. quintana, Y. pestis, C. burnetii, Borrelia, Anaplasma, Rickettsia spp. and R. prowazekii. The DNA of Acinetobacter spp. was detected in 33 of the 181 head lice (18.2%), infesting 16 of 27 individuals (59.3%). Sequencing of 350-bps fragment rpoB gene coupled with blast analysis revealed that 26 of 33 sequences (78.8%) match five species of Acinetobacter sharing 99–100% identity with their corresponding reference Acinetobacter spp., namely A. baumannii, A. pittii, A. soli, A. guillouiae and A. johnsonii. Acinetobacter baumannii was the most frequently identified species with a prevalence of 8.3%, followed by A. pittii, A. soli and A. johnsonii (1.7%), then A. guillouiae (1.1%). For two of the 33 sequences, BLAST analysis showed a homology score lower than 95%, meaning that these sequences are likely to correspond to new species, provisionally referred to here as “Candidatus Acinetobacter pediculii”. The most closely-related species is A. guillouiae (GenBank: FJ754439) with 94.9% similarity (337 of 355 base positions in common).
The remaining five of 33 sequences (15.1%) presented also some similarities with Acinetobacter. However, the sequences were of poor quality, which is assumed to be due to co-infection with several Acinetobacter species. The distribution of Acinetobacter species according to lice clades are presented in Table 4. The phylogenetic positions of all Acinetobacter species identified in this study are presented in Fig. 3. The partial rpoB sequences obtained in this study were deposited in the GenBank database under the accession numbers: MH230910-MH230920.
Phylogenetic tree highlighting the position of Acinetobacter spp. identified in head lice from DR Congo. The rpoB sequences were aligned using CLUSTALW, and phylogenetic inferences were conducted in MEGA 6 using the maximum likelihood method based on the TrN + G model for nucleotide sequences. Statistical support for internal branches of the trees was evaluated by bootstrapping with 500 iterations. There was a total of 345 positions in the final dataset
Discussion
In this study, we investigated the genetic diversity of head lice collected in DR Congo. The presence of lice from clades A, D and E was observed. The most prevalent clade was A, confirming its worldwide distribution, followed by clade D and clade E. Clades A and D were already reported in this area [5]. But this is the first report of clade E in Central Africa, which is more abundant in West Africa [6]. All positive lice for clade E arise from only two individuals. Several hypotheses could be suggested, such as the recent arrival of these people from West African countries, close contact with West African populations, or a previous implantation of these low-prevalence lice. However, only one haplotype for all clade E lice was observed. This clade, named here E62, had never been described before in West Africa. Overall, these data show that the current repartition of human lice clades is not definitive. Increasing the samples sizes and extending the geographical coverage are needed to better determine the intra- and interclade diversity [3].
In addition to the inter-haplogroup diversity, P. humanus also showed intra-haplogroup diversity, which is illustrated by numerous distinct A, B, D, E and C haplotypes [3, 7, 12, 36], results supported by our findings. Indeed, among the 54 head lice cytb sequences analyzed, ten different haplotypes were identified; in which eight haplotypes were novel. There are several reports stating that co-infestation by different mitochondrial DNA (mtDNA) clades of human lice in the same individual can occur, and it was found to be associated with clades A and B [37, 38], clades A and C [38, 39] and also for clades A and D [5], suggesting that these different clades can live in sympatry and interbreed [37, 39]. Indeed, several studies have shown evidence of recombination events that occur between different lice clades living in sympatric by using intergenic spacers [37, 39]. Moreover, louse females have lost their spermatheca and must mate before laying eggs; frequent mating is essential, and this process encourages outbreeding [40].
In our study, only half of the individuals were mono-infested by one clade of lice. Dual infestation was observed only with clades A and D in 12 individuals (44%). These data are consistent with the previous study conducted by Drali et al. [5], reporting a dual infestation with clades A and D among 14 of 37 (37.8%) infested people in DR Congo. It would be interesting to determine whether or not there is evidence of gene exchange and recombination between these different clades or whether lice are living in sympatry. Nevertheless, there was no dual infestation by both clades A and E, nor by clades D and E. Such double infestation may be due to the several infestation events. Multiple infestations may also facilitate the transmission of louse-borne pathogens. The dissemination of lice is also linked to globalization which is led by a significant dynamic of the world’s population [35].
So far, only body lice are considered as vectors of pathogenic bacteria [41, 42]. However, the role of head lice as vectors of infectious diseases is currently more and more discussed. Indeed, studies have reported the presence of DNA of pathogenic bacteria, such as B. quintana, B. recurrentis, Y. pestis and C. burnetii in head lice [5, 6, 12, 17, 20]. Here, we screened 181 head lice collected for several pathogenic bacteria. In our study, only Acinetobacter species was found and A. baumannii was the most prevalent. This is consistent with previous studies that showed that A. baumannii is the most abundant species found in head and body lice [20]. Another study conducted in Congo (Brazzaville) on lice of the pygmy populations found 10.4% of A. baumannii, as well as several other Acinetobacter species such as Acinetobacter junii (18.31%), Acinetobacter ursingii (14.35%), Acinetobacter johnsonii (9.40%), Acinetobacter schindleri (8.41%), Acinetobacter nosocomialis (3.18%), Acinetobacter lwoffii (4.45%), and Acinetobacter towneri (1.98%) [12]. Among Acinetobacter species, A. baumannii is the most important species, observed worldwide and involved in hospital-acquired infections, including epidemics that are a real challenge for public health. Currently, A. baumannii is considered a pathogen responsible for nosocomial infections, but also community acquired infections and infections related to war and natural disasters, such as war wounds among Iraqi and Afghan soldiers [42,43,44,45]. Our study is the first to describe A. soli, A. pittii and A. guillouiae in human lice. Unlike A. guillouiae which is an environmental species rarely associated with infections, A. soli and A. pitti have been isolated from clinical samples and are associated with carbapenem resistance [46, 47]. We also detected a potential new species, provisionally referred here as “Candidatus Acinetobacter pediculi” in human lice. In the phylogenetic tree (Fig. 3), the sequence of this potential new species forms a separate and well-supported (bootstrap value of 94) branch, that clustered together within the clade that contains A. guillouiae. However, the detection of this potential new species has its limitations, as not all previously described species of Acinetobacter are already molecularly characterized, so the identification of a new genotype variant may be the re-discovery of an old incompletely characterized species. Further studies are needed to confirm if this new genetic variant represents a new species. Furthermore, despite the fact that several studies have demonstrated widespread infection among lice with several species of Acinetobacter, suggesting that lice could be a preferential host for these bacteria, the association between Acinetobacter and Pediculus lice is still poorly understood [12, 18, 19]. For example, it is still unknown how these lice acquire and transmit Acinetobacter infections to their human hosts. Several reports have suggested that the infection could occur after the ingestion of infected blood meal from infected individuals [25, 48, 49]. Indeed, an experimental study demonstrated that the body louse, feeding on bacteremic rabbits, is able to acquire and maintain a persistent life-long infection with A. baumannii and A. lwoffii and disseminate viable organisms in their feces [49]. The transmission of these infections to humans occurs by contamination of bite sites, microlesions of the skin and mucous membranes with the feces or crushed bodies of infected lice [41]. Further studies are needed to investigate the specificities of the associations between lice and Acinetobacter infections.
Conclusions
In conclusion, we highlighted the presence of clade E head lice in Central Africa. The more prevalent head lice clades in DR Congo were clades A and D. Several Acinetobacter species were detected, including one potential new one. More importantly, these ubiquitous opportunistic bacteria reservoirs and their potential involvement in human infections should be put under surveillance.
Availability of data and materials
All data generated or analyzed during this study are included in this published article and its additional file. The newly generated sequences were submitted to the GenBank database under the accession numbers MH230910-MH230920 for Acinetobacter spp. and MH230921-MH230928 for cytochrome b sequences.
Abbreviations
- DR Congo:
-
Democratic Republic of the Congo
- Cytb:
-
Cytochrome b
- qPCR:
-
quantitative real-time PCR
- mtDNA:
-
mitochondrial DNA
References
Bonilla DL, Durden LA, Eremeeva ME, Dasch GA. The biology and taxonomy of head and body lice—implications for louse-borne disease prevention. PLoS Pathog. 2013;9:e1003724.
Veracx A, Raoult D. Biology and genetics of human head and body lice. Trends Parasitol. 2012;28:563–71.
Ashfaq M, Prosser S, Nasir S, Masood M, Ratnasingham S, Hebert PD. High diversity and rapid diversification in the head louse, Pediculus humanus (Pediculidae: Phthiraptera). Sci Rep. 2015;5:e14188.
Amanzougaghene N, Mumcuoglu KY, Fenollar F, Alfi S, Yesilyurt G, Raoult D, et al. High ancient genetic diversity of human lice, Pediculus humanus, from Israel reveals new insights into the origin of Clade B lice. PloS ONE. 2016;11:e0164659.
Drali R, Shako JC, Davoust B, Diatta G, Raoult D. A new clade of African body and head lice infected by Bartonella quintana and Yersinia pestis - Democratic Republic of the Congo. Am J Trop Med Hyg. 2015;93:990–3.
Amanzougaghene N, Fenollar F, Sangaré AK, Sissoko MS, Doumbo OK, Raoult D, et al. Detection of bacterial pathogens including potential new species in human head lice from Mali. PloS ONE. 2017;12:e0184621.
Light JE, Allen JM, Long LM, Carter TE, Barrow L, Suren G, et al. Geographic distributions and origins of human head lice (Pediculus humanus capitis) based on mitochondrial data. J Parasitol. 2008;94:1275–81.
Ascunce MS, Toups MA, Kassu G, Fane J, Scholl K, Reed DL. Nuclear genetic diversity in human lice (Pediculus humanus) reveals continental differences and high inbreeding among worldwide populations. PloS ONE. 2013;8:e57619.
Demastes JW, Spradling TA, Hafner MS, Spies GR, Hafner DJ, Light JE. Cophylogeny on a fine scale: Geomydoecus chewing lice and their pocket gopher hosts, Pappogeomys bulleri. J Parasitol. 2012;98:262–70.
Kim JH, Previte DJ, Yoon KS, Murenzi E, Koehler JE, Pittendrigh BR, et al. Comparison of the proliferation and excretion of Bartonella quintana between body and head lice following oral challenge. Insect Mol Biol. 2017;26:266–76.
Previte D, Olds BP, Yoon K, Sun W, Muir W, Paige KN, et al. Differential gene expression in laboratory strains of human head and body lice when challenged with Bartonella quintana, a pathogenic bacterium. Insect Mol Biol. 2014;23:244–54.
Amanzougaghene N, Akiana J, Mongo Ndombe G, Davoust B, Nsana NS, Parra HJ, et al. Head lice of pygmies reveal the presence of relapsing fever Borreliae in the Republic of Congo. PLoS Negl Trop Dis. 2016;10:e0005142.
Angelakis E, Diatta G, Abdissa A, Trape JF, Mediannikov O, Richet H, et al. Altitude-dependent Bartonella quintana genotype C in head lice, Ethiopia. Emerg Infect Dis. 2011;17:2357–9.
Boutellis A, Mediannikov O, Bilcha KD, Ali J, Campelo D, Barker SC, et al. Borrelia recurrentis in head lice, Ethiopia. Emerg Infect Dis. 2013;19:796–8.
Boutellis A, Veracx A, Angelakis E, Diatta G, Mediannikov O, Trape JF, et al. Bartonella quintana in head lice from Senegal. Vector Borne Zoonotic Dis. 2012;12:564–7.
Diatta G, Mediannikov O, Sokhna C, Bassene H, Socolovschi C, Ratmanov P, et al. Prevalence of Bartonella quintana in patients with fever and head lice from rural areas of Sine-Saloum, Senegal. Am J Trop Med Hyg. 2014;91:291–3.
Louni M, Amanzougaghene N, Mana N, Fenollar F, Raoult D, Bitam I, et al. Detection of bacterial pathogens in clade E head lice collected from Niger’s refugees in Algeria. Parasit Vectors. 2018;11:348.
Bouvresse S, Socolovshi C, Berdjane Z, Durand R, Izri A, Raoult D, et al. No evidence of Bartonella quintana but detection of Acinetobacter baumannii in head lice from elementary schoolchildren in Paris. Comp Immunol Microbiol Infect Dis. 2011;34:475–7.
Candy K, Amanzougaghene N, Izri A, Brun S, Durand R, Louni M, et al. Molecular survey of head and body lice, Pediculus humanus, in France. Vector-Borne Zoonotic Dis. 2018;18:243–51.
Sunantaraporn S, Sanprasert V, Pengsakul T, Phumee A, Boonserm R, Tawatsin A, et al. Molecular survey of the head louse Pediculus humanus capitis in Thailand and its potential role for transmitting Acinetobacter spp. Parasit Vectors. 2015;8:127.
Robinson D, Leo N, Prociv P, Barker SC. Potential role of head lice, Pediculus humanus capitis, as vectors of Rickettsia prowazekii. Parasitol Res. 2003;90:209–11.
Chosidow O. Scabies and pediculosis. Lancet. 2000;355:819–26.
La Scola B, Fournier PE, Brouqui P, Raoult D. Detection and culture of Bartonella quintana, Serratia marcescens, and Acinetobacter spp. from decontaminated human body lice. J Clin Microbiol. 2001;39:1707–9.
Li W, Ortiz G, Fournier PE, Gimenez G, Reed DL, Pittendrigh B, et al. Genotyping of human lice suggests multiple emergencies of body lice from local head louse populations. PLoS Negl Trop Dis. 2010;4:e641.
La Scola B, Gundi VA, Khamis A, Raoult D. Sequencing of the rpoB gene and flanking spacers for molecular identification of Acinetobacter species. J Clin Microbiol. 2006;44:827–32.
Nguyen-Hieu T, Aboudharam G, Signoli M, Rigeade C, Drancourt M, Raoult D. Evidence of a louse-borne outbreak involving typhus in Douai, 1710-1712 during the war of Spanish succession. PloS ONE. 2010;5:e15405.
Parola P, Diatta G, Socolovschi C, Mediannikov O, Tall A, Bassene H, et al. Tick-borne relapsing fever borreliosis, rural Senegal. Emerg Infect Dis. 2011;17:883–5.
Dahmani M, Davoust B, Rousseau F, Raoult D, Fenollar F, Mediannikov O. Natural Anaplasmataceae infection in Rhipicephalus bursa ticks collected from sheep in the French Basque Country. Ticks Tick-Borne Dis. 2017;8:18–24.
Mediannikov O, Diatta G, Fenollar F, Sokhna C, Trape JF, Raoult D. Tick-borne rickettsioses, neglected emerging diseases in rural Senegal. PLoS Negl Trop Dis. 2010;4:e821.
Sokhna C, Mediannikov O, Fenollar F, Bassene H, Diatta G, Tall A, et al. Point-of-care laboratory of pathogen diagnosis in Rural Senegal. PLoS Negl Trop Dis. 2013;7:e1999.
Bandelt HJ, Forster P, Röhl A. Median-joining networks for inferring intraspecific phylogenies. Mol Biol Evol. 1999;16:37–48.
Tamura K, Stecher G, Peterson D, Filipski A, Kumar S. MEGA6: Molecular Evolutionary Genetics Analysis version 6.0. Mol Biol Evol. 2013;30:2725–9.
Posada D, Crandall KA. Modeltest: testing the model of DNA substitution. Bioinformatics. 1998;14:817–8.
Posada D, Buckley TR. Model selection and model averaging in phylogenetics: advantages of Akaike information criterion and bayesian approaches over likelihood ratio tests. Syst Biol. 2004;53:793–808.
Huelsenbeck JP, Rannala B. Phylogenetic methods come of age: testing hypotheses in an evolutionary context. Science. 1997;276:227–32.
Ascunce MS, Fane J, Kassu G, Toloza AC, Picollo MI, González-Oliver A, et al. Mitochondrial diversity in human head louse populations across the Americas. Am J Phys Anthropol. 2013;152:118–29.
Boutellis A, Bitam I, Fekir K, Mana N, Raoult D. Evidence that clade A and clade B head lice live in sympatry and recombine in Algeria. Med Vet Entomol. 2015;29:94–8.
Xiong H, Campelo D, Boutellis A, Raoult D, Alem M, Ali J, et al. SNPs in entire mitochondrial genome sequences (≈15.4 kb) and cox1 sequences (≈486 bp) resolve body and head lice from doubly infected people from Ethiopia, China, Nepal, and Iran but not France. J Med Entomol. 2014;51:1199–207.
Veracx A, Boutellis A, Raoult D. Genetic recombination events between sympatric Clade A and Clade C lice in Africa. J Med Entomol. 2013;50:1165–8.
Boutellis A, Abi-Rached L, Raoult D. The origin and distribution of human lice in the world. Infect Genet Evol. 2014;23:209–17.
Raoult D, Roux V. The body louse as a vector of reemerging human diseases. Clin Infect Dis. 1999;29:888–911.
Zinsser H, Grob GN. Rats, lice and history. Abington: Taylor & Francis; 2008.
Eveillard M, Kempf M, Belmonte O, Pailhoriès H, Joly-Guillou ML. Reservoirs of Acinetobacter baumannii outside the hospital and potential involvement in emerging human community-acquired infections. Int J Infect Dis. 2013;17:e802–5.
Peleg AY, Seifert H, Paterson DL. Acinetobacter baumannii: emergence of a successful pathogen. Clin Microbiol Rev. 2008;21:538–82.
Johanson WG, Pierce AK, Sanford JP. Changing pharyngeal bacterial flora of hospitalized patients. Emergence of gram-negative bacilli. N Engl J Med. 1969;281:1137–40.
Pellegrino FL, Vieira VV, Baio PV, dos Santos RM, dos Santos AL, Santos NG, et al. Acinetobacter soli as a cause of bloodstream infection in a neonatal intensive care unit. J Clin Microbiol. 2011;49:2283–5.
Endo S, Sasano M, Yano H, Arai K, Aoyagi T, Hatta M, et al. First carbapenem-resistant isolates of Acinetobacter soli in Japan. Antimicrob Agents Chemother. 2012;56:2786–7.
Kempf M, Abdissa A, Diatta G, Trape JF, Angelakis E, Mediannikov O, et al. Detection of Acinetobacter baumannii in human head and body lice from Ethiopia and identification of new genotypes. Int J Infect Dis. 2012;16:e680–3.
Houhamdi L, Raoult D. Experimental infection of human body lice with Acinetobacter baumannii. Am J Trop Med Hyg. 2006;74:526–31.
Acknowledgements
We thank medical staff involved in this study and patients who agreed to participate. This study was supported by the Institut Hospitalo-Universitaire (IHU) Méditerranée Infection, the National Research Agency under the program « Investissements d’avenir », reference ANR-10-IAHU-03, the Région Provence Alpes Côte d’Azur and European funding FEDER PRIMI.
Funding
This study was supported by the Institut Hospitalo-Universitaire (IHU) Méditerranée Infection, the National Research Agency under the programme “Investissements d’avenir”, reference ANR-10-IAHU-03, the Région Provence Alpes Côte d’Azur and European funding FEDER IHUBIOTK.
Author information
Authors and Affiliations
Contributions
Conception or design of the work, revision: FF, OM, DR. Analysis, interpretation data and drafting: CSBK. Interpretation of data and substantial revision: NA. Sample collection: OM, LT, BD. Substantial revision of manuscript: BD, LT, JBLD. All authors read and approved the final manuscript.
Corresponding author
Ethics declarations
Ethics approval and consent to participate
This study was approved by the Central Ethic Committee (CEL) of CEFA (Centre de Formation et d’Appui sanitaire) associated with Monkole Hospital Center (N/Réf:020/CEFA-MONKOLE/CEL/2017). Lice collection was carried out at the Monkole Hospital Center located in Kinshasa, the largest city and capital of DR Congo. All required consents were obtained from the individuals involved or their legal representatives in the case of children. Informed consent was oral as participants were illiterate. The Institutional Review Board approved the use of oral consent. Dr Oleg Mediannikov participated in the collection and was a witness of the participantʼs consents. Professor Leon Tshilolo, physician director of Monkole Hospital Center, was also present.
Consent for publication
Not applicable.
Competing interests
The authors declare that they have no competing interests.
Additional information
Publisher's Note
Springer Nature remains neutral with regard to jurisdictional claims in published maps and institutional affiliations.
Additional file
Additional file 1.
Geographical occurrences and frequencies of cytb haplotypes of human head and body lice.
Rights and permissions
Open Access This article is distributed under the terms of the Creative Commons Attribution 4.0 International License (http://creativecommons.org/licenses/by/4.0/), which permits unrestricted use, distribution, and reproduction in any medium, provided you give appropriate credit to the original author(s) and the source, provide a link to the Creative Commons license, and indicate if changes were made. The Creative Commons Public Domain Dedication waiver (http://creativecommons.org/publicdomain/zero/1.0/) applies to the data made available in this article, unless otherwise stated.
About this article
Cite this article
Boumbanda Koyo, C.S., Amanzougaghene, N., Davoust, B. et al. Genetic diversity of human head lice and molecular detection of associated bacterial pathogens in Democratic Republic of Congo. Parasites Vectors 12, 290 (2019). https://doi.org/10.1186/s13071-019-3540-6
Received:
Accepted:
Published:
DOI: https://doi.org/10.1186/s13071-019-3540-6