Abstract
Disuse induces a rapid bone loss in humans and animals; hypodynamia/sedentarity is now recognized as a risk factor for osteoporosis. Hypodynamia also decreases bone mass but its effects are largely unknown and only few animal models have been described. Hypodynamic chicken is recognized as a suitable model of bone loss but the effects on the quality have not been fully explored. We have used ten chickens bred in a large enclosure (FREE group); ten others were confined in small cages with little space to move around (HYPO group). They were sacrificed at 53 days and femurs were evaluated by microcomputed tomography (microCT) and nanoindentation. Sections (4 µm thick) were analyzed by Fourier Transform InfraRed Microspectroscopy (FTIR) to see the effects on mineralization and collagen and quantitative backscattered electron imaging (qBEI) to image the mineral of the bone matrix. Trabecular bone volume and microarchitecture were significantly altered in the HYPO group. FTIR showed a significant reduction of the mineral-to-matrix ratio in the HYPO group associated with an increase in the carbonate content and an increase in crystallinity (calculated as the area ratio of subbands located at 1020 and 1030 cm−1) indicating a poor quality of the mineral. Collagen maturity (calculated as the area ratio of subbands located at 1660 and 1690 cm−1) was significantly reduced in the HYPO group. Reduced biomechanical properties were observed at the tissue level. Confined chicken represents a new model for the study of hypodynamia because bone changes are not created by a surgical lesion or a traumatic method. Animals have a reduced bone mass and present with an altered bone matrix quality which is less mineralized and whose collagen contains less crosslinks than in control chicken.





Similar content being viewed by others
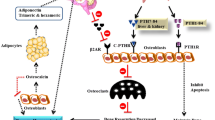
References
Klein-Nulend J, Bakker AD, Bacabac RG, Vatsa A, Weinbaum S (2013) Mechanosensation and transduction in osteocytes. Bone 54:182–190
Morey ER, Baylink DJ (1978) Inhibition of bone formation during space flight. Science 201:1138–1141
Vernikos J, Schneider VS (2010) Space, gravity and the physiology of aging: parallel or convergent disciplines? A mini-review. Gerontology 56:157–166
Iwase S, Nishimura N, Mano T (2013) Osteoporosis in Spaceflight. In: Valdés-Flores (ed) Topics in Osteoporosis. InTech. http://www.intechopen.com/books/topics-in-osteoporosis/osteoporosis-in-spaceflight
Krølner B, Toft B (1983) Vertebral bone loss: an unheeded side effect of therapeutic bed rest. Clin Sci 64:537–540
Minaire P, Neunier P, Edouard C, Bernard J, Courpron P, Bourret J (1974) Quantitative histological data on disuse osteoporosis: comparison with biological data. Calcif Tissue Res 17:57–73
Garland DE, Stewart CA, Adkins RH, Hu SS, Rosen C, Liotta FJ, Weinstein DA (1992) Osteoporosis after spinal cord injury. J Orthop Res 10:371–378
Berg H, Dudley G, Haggmark T, Ohlsen H, Tesch P (1991) Effects of lower limb unloading on skeletal muscle mass and function in humans. J Appl Physiol 70:1882–1885
Bloomfield SA (1997) Changes in musculoskeletal structure and function with prolonged bed rest. Med Sci Sports Exerc 29:197–206
Rubin C, Turner AS, Müller R, Mittra E, McLeod K, Lin W, Qin YX (2002) Quantity and quality of trabecular bone in the femur are enhanced by a strongly anabolic, noninvasive mechanical intervention. J Bone Miner Res 17:349–357
Ferretti JL, Cointry GR, Capozza RF, Frost HM (2003) Bone mass, bone strength, muscle–bone interactions, osteopenias and osteoporoses. Mech Ageing Dev 124:269–279
Turner CH, Robling AG (2003) Designing exercise regimens to increase bone strength. Exerc Sport Sci Rev 31:45–50
Sinaki M, Pfeifer M, Preisinger E, Itoi E, Rizzoli R, Boonen S, Geusens P, Minne HW (2010) The role of exercise in the treatment of osteoporosis. Curr Osteoporos Rep 8:138–144
Gómez-Cabello A, Ara I, González-Agüero A, Casajús JA, Vicente-Rodríguez G (2012) Effects of training on bone mass in older adults: A systematic review. Sports Med 42:301–325
Felsenberg D, Boonen S (2005) The bone quality framework: determinants of bone strength and their interrelationships, and implications for osteoporosis management. Clin Ther 27:1–11
Herrmann D, Buck C, Sioen I, Kouride Y, Marild S, Molnár D, Mouratidou T, Pitsiladis Y, Russo P, Veidebaum T (2015) Impact of physical activity, sedentary behaviour and muscle strength on bone stiffness in 2–10-year-old children-cross-sectional results from the IDEFICS study. Int J Behav Nutr Phys Act 12:112
Nilsson M, Ohlsson C, Mellström D, Lorentzon M (2013) Sport-specific association between exercise loading and the density, geometry, and microstructure of weight-bearing bone in young adult men. Osteoporos Int 24:1613–1622
Chappard D, Baslé MF, Legrand E, Audran M (2011) New laboratory tools in the assessment of bone quality. Osteoporos Int 22:2225–2240
Libouban H (2014) Tissu osseux et contraintes mécaniques: immobilisation et microgravité. In: Guillaume B, Audran M, Chappard D (eds) Tissu osseux et biomatériaux en chirurgie dentaire. Quintessence International, Paris, p 197–208
Jee WS, Ma Y (1999) Animal models of immobilization osteopenia. Morphologie 83:25–34
Chowdhury P, Long A, Harris G, Soulsby ME, Dobretsov M (2013) Animal model of simulated microgravity: a comparative study of hindlimb unloading via tail versus pelvic suspension. Physiol Rep 1:e00012
Chappard D, Chennebault A, Moreau M, Legrand E, Audran M, Basle MF (2001) Texture analysis of X-ray radiographs is a more reliable descriptor of bone loss than mineral content in a rat model of localized disuse induced by the Clostridium botulinum toxin. Bone 28:72–79
Marchand-Libouban H, Le Drevo MA, Chappard D (2013) Disuse induced by botulinum toxin affects the bone marrow expression profile of bone genes leading to a rapid bone loss. J Musculoskelet Neuronal Interact 13:27–36
Aguado E, Pascaretti-Grizon F, Goyenvalle E, Audran M, Chappard D (2015) Bone mass and bone quality are altered by hypoactivity in the chicken. PloS One 10:e0116763
Tassani S, Korfiatis V, Matsopoulos G (2014) Influence of segmentation on micro-CT images of trabecular bone. J Microsc 256:75–81
Dempster DW, Compston JE, Drezner MK, Kanis JA, Malluche H (2013) Standardized nomenclature, symbols, and units for bone histomorphometry: A 2012 update of the report of the ASBMR Histomorphometry Nomenclature Committee. J Bone Miner Res 28:2–17
Chappard D (2014) Technical aspects: How do we best prepare bone samples for proper histological analysis? In: Heymann D (ed) Bone cancer: progression and therapeutic approaches. Elsevier Inc., London, pp 111–120
Gamsjaeger S, Mendelsohn R, Boskey AL, Gourion-Arsiquaud S, Klaushofer K, Paschalis EP (2014) Vibrational spectroscopic imaging for the evaluation of matrix and mineral chemistry. Curr Osteoporos Rep 12:454–464
Paschalis EP (2012) Fourier transform infrared imaging of bone. Methods Mol Med 816:517–525
Strawn S, White J, Marshall G, Gee L, Goodis H, Marshall S (1996) Spectroscopic changes in human dentine exposed to various storage solutions—short term. J Dent 24:417–423
Mason JT, O’leary TJ (1991) Effects of formaldehyde fixation on protein secondary structure: a calorimetric and infrared spectroscopic investigation. J Histochem Cytochem 39:225–229
Pleshko NL, Boskey AL, Mendelsohn R (1992) An infrared study of the interaction of polymethyl methacrylate with the protein and mineral components of bone. J Histochem Cytochem 40:1413–1417
Pleshko NL, Boskey AL, Mendelsohn R (1992) An FT-IR microscopic investigation of the effects of tissue preservation on bone. Calcif Tissue Int 51:72–77
Mieczkowska A, Mansur SA, Irwin N, Flatt PR, Chappard D, Mabilleau G (2015) Alteration of the bone tissue material properties in type 1 diabetes mellitus: a Fourier transform infrared microspectroscopy study. Bone 76:31–39
Boskey AL, Pleshko N, S.B. D, Mendelsohn R (1992) Applications of Fourier transform infrared (FT-IR) microscopy to the study of mineralization in bone and cartilage. Cells Mater 2:209–220
Boskey A, DiCarlo E, Paschalis E, West P, Mendelsohn R (2005) Comparison of mineral quality and quantity in iliac crest biopsies from high-and low-turnover osteoporosis: an FT-IR microspectroscopic investigation. Osteoporos Int 16:2031–2038
Paschalis EP, Verdelis K, Doty SB, Boskey AL, Mendelsohn R, Yamauchi M (2001) Spectroscopic characterization of collagen cross-links in bone. J Bone Miner Res 16:1821–1828
Ou-Yang H, Paschalis EP, Mayo WE, Boskey AL, Mendelsohn R (2001) Infrared microscopic imaging of bone: spatial distribution of CO3(2-). J Bone Miner Res 16:893–900
Oliver WC, Pharr GM (1992) An improved technique for determining hardness and elastic modulus using load and displacement sensing indentation experiments. J Mater Res 7:1564–1583
Edwards WB, Schnitzer TJ (2015) Bone imaging and fracture risk after spinal cord injury. Curr Osteoporosis Rep 13:310–317
Kazakia GJ, Tjong W, Nirody JA, Burghardt AJ, Carballido-Gamio J, Patsch JM, Link T, Feeley BT, Ma CB (2014) The influence of disuse on bone microstructure and mechanics assessed by HR-pQCT. Bone 63:132–140
Bouvard B, Mabilleau G, Legrand E, Audran M, Chappard D (2012) Micro and macroarchitectural changes at the tibia after botulinum toxin injection in the growing rat. Bone 50:858–864
Kun-Darbois JD, Libouban H, Chappard D (2015) Botulinum toxin in masticatory muscles of the adult rat induces bone loss at the condyle and alveolar regions of the mandible associated with a bone proliferation at a muscle enthesis. Bone 77:75–82
Chappard D, Libouban H (2016) Bone loss also concerns the epiphysis in the BTX rat model of disuse osteoporosis. J Anat Soc Ind 65:3–8
Manske SL, Boyd SK, Zernicke RF (2010) Muscle and bone follow similar temporal patterns of recovery from muscle-induced disuse due to botulinum toxin injection. Bone 46:24–31
Rauch F, Hamdy R (2006) Effect of a single botulinum toxin injection on bone development in growing rabbits. J Musculoskelet Neuronal Interact 6:264–268
Thomsen JS, Christensen LL, Vegger JB, Nyengaard JR, Bruel A (2012) Loss of bone strength is dependent on skeletal site in disuse osteoporosis in rats. Calcif Tissue Int 90:294–306
Kyrou I, Tsigos C (2009) Stress hormones: physiological stress and regulation of metabolism. Curr Opin Pharmacol 9:787–793
Hartmann K, Koenen M, Schauer S, Wittig-Blaich S, Ahmad M, Baschant U, Tuckermann JP (2016) Molecular actions of glucocorticoids in cartilage and bone during health, disease, and steroid therapy. Physiol Rev 96:409–447
Paschalis E, Betts F, DiCarlo E, Mendelsohn R, Boskey A (1997) FTIR microspectroscopic analysis of human iliac crest biopsies from untreated osteoporotic bone. Calcif Tissue Int 61:487–492
Paschalis E, Gamsjaeger S, Tatakis DN, Hassler N, Robins S, Klaushofer K (2015) Fourier transform Infrared spectroscopic characterization of mineralizing type I collagen enzymatic trivalent cross-links. Calcif Tissue Int 96:18–29
Paschalis EP, Shane E, Lyritis G, Skarantavos G, Mendelsohn R, Boskey AL (2004) Bone fragility and collagen cross-links. J Bone Miner Res 19:2000–2004
Paschalis EP, Boskey AL, Kassem M, Eriksen EF (2003) Effect of hormone replacement therapy on bone quality in early postmenopausal women. J Bone Miner Res 18:955–959
Gourion-Arsiquaud S, Faibish D, Myers E, Spevak L, Compston J, Hodsman A, Shane E, Recker RR, Boskey ER, Boskey AL (2009) Use of FTIR spectroscopic imaging to identify parameters associated with fragility fracture. J Bone Miner Res 24:1565–1571
Burket JC, Brooks DJ, MacLeay JM, Baker SP, Boskey AL, van der Meulen MC (2013) Variations in nanomechanical properties and tissue composition within trabeculae from an ovine model of osteoporosis and treatment. Bone 52:326–336
Masci M, Wang M, Imbert L, Barnes AM, Spevak L, Lukashova L, Huang Y, Ma Y, Marini JC, Jacobsen CM, Warman ML, Boskey AL (2016) Bone mineral properties in growing Col1a2(+/G610C) mice, an animal model of osteogenesis imperfecta. Bone 87:120–129
Aparicio S, Doty S, Camacho N, Paschalis E, Spevak L, Mendelsohn R, Boskey A (2002) Optimal methods for processing mineralized tissues for Fourier transform infrared microspectroscopy. Calcif Tissue Int 70:422–429
Carson F, Hladik C (2009) Fixation. In: Carson F, Hladik C (eds) Histotechnology: A Self-Instructional Text. American Society for Clinical Pathology Press Chicago, IL, p 9–13
Ammann P, Badoud I, Barraud S, Dayer R, Rizzoli R (2007) Strontium ranelate treatment improves trabecular and cortical intrinsic bone tissue quality, a determinant of bone strength. J Bone Miner Res 22:1419–1425
Brennan TC, Rizzoli R, Ammann P (2009) Selective modification of bone quality by PTH, pamidronate, or raloxifene. J Bone Miner Res 24:800–808
Shipov A, Sharir A, Zelzer E, Milgram J, Monsonego-Ornan E, Shahar R (2010) The influence of severe prolonged exercise restriction on the mechanical and structural properties of bone in an avian model. Veter J 183:153–160
Chappard D, Baslé MF, Legrand E, Audran M (2008) Trabecular bone microarchitecture: a review. Morphologie 92:162–170
Acknowledgements
This work was made possible by grants from the Ministry of Research. Many thanks to Mrs. Lechat for secretarial assistance, N. Retailleau for microCT, and A. Mieczkowska for FTIR analysis.
Author contributions
This study was conceived by EA and EG, and initiated by DC. Experimental analyses were designed by DC and performed by GM and the acknowledged technicians. Analysis of the data was performed by DC. The paper was written by DC and EA, the manuscript was revised by GM, and the final manuscript was approved by all the authors. There is no potential conflict of interest for all of the authors.
Author information
Authors and Affiliations
Corresponding author
Ethics declarations
Conflict of interest
Eric Aguado, Guillaume Mabilleau, Eric Goyenvalle, and Daniel Chappard have no conflict of interest.
Human and Animal Rights and Informed Consent
This article does not contain any studies with human participants performed by any of the authors. All procedures performed in the study were reviewed and approved by the ONIRIS Council on Animal Care (National Veterinary School of Nantes) and the Regional ethical Committee and conformed to the guidelines of the French Animal Care Committee.
Rights and permissions
About this article
Cite this article
Aguado, E., Mabilleau, G., Goyenvalle, E. et al. Hypodynamia Alters Bone Quality and Trabecular Microarchitecture. Calcif Tissue Int 100, 332–340 (2017). https://doi.org/10.1007/s00223-017-0235-x
Received:
Accepted:
Published:
Issue Date:
DOI: https://doi.org/10.1007/s00223-017-0235-x