Abstract
Lipids have numerous important functions in the human body, as they form the cells’ plasma membranes and play a key role in many disease states, presumably also in osteoporosis. Here, the fatty acid composition of the outer plasma membranes of cells differentiated into the osteogenic and adipogenic direction is studied with surface-sensitive time-of-flight secondary ion mass spectrometry (ToF-SIMS). For data evaluation, principal component analysis (PCA) is applied. Human (bone-derived) mesenchymal stromal cells (hMSCs) from an osteoporotic donor and a control donor are compared to reveal differences in the fatty acid composition of the membranes. The chemical information is correlated to staining and real-time quantitative polymerase chain reaction (rt-qPCR) results to provide insight into the gene expression of several differentiation markers on the RNA level. Adipogenic differentiation of hMSCs from a non-osteoporotic donor correlates with increased relative intensities of all fatty acids under investigation. After osteogenic differentiation of non-osteoporotic cells, the relative mass signal intensities of unsaturated fatty acids such as oleic and linoleic acids are increased. However, the osteoporotic cells show increased levels of palmitic acid in the plasma membrane after exposure to osteogenic differentiation conditions, which correlates to an immature differentiation state relative to non-osteoporotic osteogenic cells. This immature differentiation state is confirmed by increased early osteogenic differentiation factor Runx2 on RNA level and by less calcium mineralization spots seen in von Kossa staining and ToF-SIMS images.

Time-of-flight secondary ion mass spectrometry is applied to analyze the fatty acid composition of the outer plasma membranes of cells differentiated into the adipogenic and osteogenic direction. Cells from an osteoporotic and a control donor are compared to reveal differences due to differentiation and disease stage of the cells.






Similar content being viewed by others
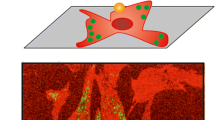
References
Murphy SA, Nicolaou A. Lipidomics applications in health, disease and nutrition research. Mol Nutr Food Res. 2013;57(8):1336–46.
Lee C-H, Olson P, Evans RM. Minireview: lipid metabolism, metabolic diseases, and peroxisome proliferator-activated receptors. Endocrinology. 2003;144(6):2201–7.
Maxfield FR, Tabas I. Role of cholesterol and lipid organization in disease. Nature. 2005;438(7068):612–21.
Wenk MR. The emerging field of lipidomics. Nat Rev Drug Discov. 2005;4(7):594–610.
Wenk MR. Lipidomics: new tools and applications. Cell. 2010;143(6):888–95.
Passarelli MK, Ewing AG. Single-cell imaging mass spectrometry. Curr Opin Chem Biol. 2013;17(5):854–9.
Fernández JA, Ochoa B, Fresnedo O, Giralt MT, Rodríguez-Puertas R. Matrix-assisted laser desorption ionization imaging mass spectrometry in lipidomics. Anal Bioanal Chem. 2011;401(1):29–51.
Santos CR, Schulze A. Lipid metabolism in cancer. FEBS J. 2012;279(15):2610–23.
Stubbs CD, Smith AD. The modification of mammalian membrane polyunsaturated fatty acid composition in relation to membrane fluidity and function. Biochim Biophys Acta Rev Biomembr. 1984;779(1):89–137.
Anitschkow N, Chalatow S. Ueber experimentelle Cholesterinsteatose und ihre Bedeutung für die Entstehung einiger pathologischer Prozesse. bl allg Path path Anat. 1913;24(24):1–9.
Steinberg D. Atherogenesis in perspective: hypercholesterolemia and inflammation as partners in crime. Nat Med. 2002;8(11):1211–7.
Pilon M. Revisiting the membrane-centric view of diabetes. Lipids Health Dis. 2016;15(1):167.
Greco EA, Lenzi A, Migliaccio S. The obesity of bone. Ther Adv Endocrinol Metab. 2015;6(6):273–86.
Bermeo S, Gunaratnam K, Duque G. Fat and bone interactions. Curr Osteoporos Rep. 2014;12(2):235–42.
Devlin MJ, Rosen CJ. The bone–fat interface. Basic and clinical implications of marrow adiposity. Lancet Diabetes Endocrinol. 2015;3(2):141–7.
Lecka-Czernik B, Stechschulte LA. Bone and fat. A relationship of different shades. Arch Biochem Biophys. 2014;561:124–9.
Schwartz AV. Marrow fat and bone. Review of clinical findings. Front Endocrinol. 2015;6(107):14.
Scheller EL, Rosen CJ. What’s the matter with MAT? Marrow adipose tissue, metabolism, and skeletal health. Ann N Y Acad Sci. 2014;1311(1):14–30.
Hardouin P, Pansini V, Cortet B. Bone marrow fat. Joint Bone Spine. 2014;81(4):313–9.
Duque G. Bone and fat connection in aging bone. Curr Opin Rheumatol. 2008;20(4):429–34.
Zhao L-J, Jiang H, Papasian CJ, Maulik D, Drees B, Hamilton J, Deng H-W. Correlation of obesity and osteoporosis: effect of fat mass on the determination of osteoporosis. J Bone Miner Res. 2008;23(1):17–29.
Rosen CJ, Bouxsein ML. Mechanisms of disease: is osteoporosis the obesity of bone? Nat Clin Pract Rheumatol. 2006;2(1):35–43.
Duque G. As a matter of fat. New perspectives on the understanding of age-related bone loss. IBMS BoneKEy. 2007;4(4):129–40.
Johansson B. ToF-SIMS imaging of lipids in cell membranes. Surf Interface Anal. 2006;38(11):1401–12.
Frisz JF, Choi JS, Wilson RL, Harley BAC, Kraft ML. Identifying differentiation stage of individual primary hematopoietic cells from mouse bone marrow by multivariate analysis of TOF-secondary ion mass spectrometry data. Anal Chem. 2012;84(10):4307–13.
Wenisch S, Trinkaus K, Hild A, Hose D, Herde K, Heiss C, Kilian O, Alt V, Schnettler R. Human reaming debris: a source of multipotent stem cells. Bone. 2005;36(1):74–83.
Bruedigam C, van Driel M, Koedam M, van de Peppel J, van der Eerden BCJ, Eijken M, et al. Basic techniques in human mesenchymal stem cell cultures: differentiation into osteogenic and adipogenic lineages, genetic perturbations, and phenotypic analyses. Curr Protoc Stem Cell Biol. 2011 Chapter 1:Unit1H.3.
Wenisch S, Trinkaus K, Hild A, Hose D, Heiss C, Alt V, Klisch C, Meissl H, Schnettler R. Immunochemical, ultrastructural and electrophysiological investigations of bone-derived stem cells in the course of neuronal differentiation. Bone. 2006;38(6):911–21.
Glenske K, Wagner A-S, Hanke T, Cavalcanti-Adam EA, Heinemann S, Heinemann C, Kruppke B, Arnhold S, Moritz A, Schwab EH, Worch H, Wenisch S. Bioactivity of xerogels as modulators of osteoclastogenesis mediated by connexin 43. Biomaterials. 2014;35(5):1487–95.
Goergen J, Wenisch S, Raabe O, Moritz A, Schlewitz G, Schnettler R, Hempel U, Heiss C, Arnhold S. Characterization of bone-marrow-derived stem cells in osteoporotic models of the rat. ISRN Stem Cells. 2013;2013(5):1–9.
Schaepe K, Kokesch-Himmelreich J, Rohnke M, Wagner A-S, Schaaf T, Wenisch S, Janek J. Assessment of different sample preparation routes for mass spectrometric monitoring and imaging of lipids in bone cells via ToF-SIMS. Biointerphases. 2015;10(1):19016.
Schaepe K, Kokesch-Himmelreich J, Rohnke M, Wagner A-S, Schaaf T, Henss A, Wenisch S, Janek J. Storage of cell samples for ToF-SIMS experiments—how to maintain sample integrity. Biointerphases. 2016;11(2):02A313.
Todd JFJ. Recommendations for nomenclature and symbolism for mass spectroscopy (including an appendix of terms used in vacuum technology). (Recommendations 1991). Pure Appl Chem. 1991;63(10):1541–66.
Graham DJ, Castner DG. Multivariate analysis of ToF-SIMS data from multicomponent systems: the why, when, and how. Biointerphases. 2012;7(1–4):1–12.
Graham DJ. NESAC/BIO toolbox. Spectragui. http://www.nb.uw.edu/mvsa/multivariate-surface-analysis-homepage. Accessed 17 Jan 2017.
Robinson MA, Graham DJ, Morrish F, Hockenbery D, Gamble LJ. Lipid analysis of eight human breast cancer cell lines with ToF-SIMS. Biointerphases. 2015;11(2):2.
Cizinauskas V, Elie N, Brunelle A, Briedis V. Fatty acids penetration into human skin ex vivo: a TOF-SIMS analysis approach. Biointerphases. 2017;12(1):11003.
Amaya KR, Sweedler JV, Clayton DF. Small molecule analysis and imaging of fatty acids in the zebra finch song system using time-of-flight-secondary ion mass spectrometry. J Neurochem. 2011;118(4):499–511.
Sjövall P, Johansson B, Belazi D, Stenvinkel P, Lindholm B, Lausmaa J, Schalling M. TOF-SIMS analysis of adipose tissue from patients with chronic kidney disease. Appl Surf Sci. 2008;255(4):1177–80.
Magnusson Y, Friberg P, Malmberg P, Chen Y. Application of multivariate analysis of TOF-SIMS spectra for studying the effect of high glucose intake on aortic lipid profile. Appl Surf Sci. 2008;254(20):6580–5.
Sousa MJ, Balcão VM, Malcata FX. Evolution of free fatty acid profile during ripening in cheeses manufactured from bovine, ovine and caprine milks with extracts of Cynara cardunculus as coagulant. Zeitschrift für Lebensmitteluntersuchung und -Forschung A. 1997;205(2):104–7.
Leefmann T, Heim C, Kryvenda A, Siljeström S, Sjövall P, Thiel V. Biomarker imaging of single diatom cells in a microbial mat using time-of-flight secondary ion mass spectrometry (ToF-SIMS). Org Geochem. 2013;57:23–33.
Stoll LL, Spector AA. Changes in serum influence the fatty acid composition of established cell lines. In Vitro. 1984;20(9):732–8.
Lagarde M, Sicard B, Guichardant M, Felisi O, Dechavanne M. Fatty acid composition in native and cultured human endothelial cells. In Vitro. 1984;20(1):33–7.
Mansson HL. Fatty acids in bovine milk fat. Food Nutr Res. 2008;52(1):1821.
Riminucci M, Bianco P. Building bone tissue. Matrices and scaffolds in physiology and biotechnology. Braz J Med Biol Res. 2003;36(8):1027–36.
Anselme K. Osteoblast adhesion on biomaterials. Biomaterials. 2000;21(7):667–81.
Boonrungsiman S, Gentleman E, Carzaniga R, Evans ND, McComb DW, Porter AE, Stevens MM. The role of intracellular calcium phosphate in osteoblast-mediated bone apatite formation. Proc Natl Acad Sci U S A. 2012;109(35):14170–5.
Wagner MS, Castner DG. Characterization of adsorbed protein films by time-of-flight secondary ion mass spectrometry with principal component analysis. Langmuir. 2001;17(15):4649–60.
Kokesch-Himmelreich J, Schumacher M, Rohnke M, Gelinsky M, Janek J. ToF-SIMS analysis of osteoblast-like cells and their mineralized extracellular matrix on strontium enriched bone cements. Biointerphases. 2013;8(1):17.
Wagner A-S, Glenske K, Wolf V, Fietz D, Mazurek S, Hanke T, Moritz A, Arnhold S, Wenisch S. Osteogenic differentiation capacity of human mesenchymal stromal cells in response to extracellular calcium with special regard to connexin 43. Ann Anat = Anatomischer Anzeiger: official organ of the Anatomische Gesellschaft. 2017;209:18–24.
Tontonoz P, Hu E, Spiegelman BM. Stimulation of adipogenesis in fibroblasts by PPARγ2, a lipid-activated transcription factor. Cell. 1994;79(7):1147–56.
Xu J, Li Z, Hou Y, Fang W. Potential mechanisms underlying the Runx2 induced osteogenesis of bone marrow mesenchymal stem cells. Am J Transl Res. 2015;7(12):2527–35.
Carlred L, Gunnarsson A, Solé-Domènech S, Johansson B, Vukojević V, Terenius L, Codita A, Winblad B, Schalling M, Höök F, Sjövall P. Simultaneous imaging of amyloid-β and lipids in brain tissue using antibody-coupled liposomes and time-of-flight secondary ion mass spectrometry. J Am Chem Soc. 2014;136(28):9973–81.
Carlred L, Vukojević V, Johansson B, Schalling M, Höök F, Sjövall P. Imaging of amyloid-β in Alzheimer’s disease transgenic mouse brains with ToF-SIMS using immunoliposomes. Biointerphases. 2016;11(2):02A312.
Acknowledgments
This study was funded by the Deutsche Forschungsgemeinschaft (DFG) as part of the Collaborative Research Centre Transregio 79 (SFB/TRR 79—subproject M5 in collaboration with subproject B11). Kaija Schaepe gratefully acknowledges the financial support of the Friedrich Naumann Foundation for Freedom. We thank Svenja Otto for the help with the data analysis and Alena-Svenja Wagner and Anne Hild for many helpful discussions. The authors thank Dan Graham, Ph.D., for developing the NESAC/BIO Toolbox used in this study and NIH grant EB-002027 for supporting the toolbox development.
Author information
Authors and Affiliations
Corresponding author
Ethics declarations
Conflict of interest
The authors declare that they have no conflict of interest.
Research involving human participants and/or animals
The experiments were approved by the local ethical committee of the Medical Faculty of the Justus Liebig University of Giessen (decision 05/06 and 106/06).
Informed consent
The femoral heads from patients, undergoing total hip arthroplasty due to primary osteoarthritis, were provided from Asklepios Clinic Lich GmbH (Lich, Germany). All patients gave their informed consent to provide the retrieved biological material for the purpose of scientific research.
Electronic supplementary material
ESM 1
(PDF 244 kb)
Rights and permissions
About this article
Cite this article
Schaepe, K., Werner, J., Glenske, K. et al. ToF-SIMS study of differentiation of human bone-derived stromal cells: new insights into osteoporosis. Anal Bioanal Chem 409, 4425–4435 (2017). https://doi.org/10.1007/s00216-017-0386-7
Received:
Revised:
Accepted:
Published:
Issue Date:
DOI: https://doi.org/10.1007/s00216-017-0386-7