Abstract
During summer drought, Mediterranean fluvial networks are transformed into highly heterogeneous landscapes characterized by different environments (i.e., running and impounded waters, isolated river pools and dry beds). This hydrological setting defines novel biogeochemically active areas that could potentially increase the rates of carbon emissions from the fluvial network to the atmosphere. Using chamber methods, we aimed to identify hot spots for carbon dioxide (CO2) and methane (CH4) emissions from two typical Mediterranean fluvial networks during summer drought. The CO2 efflux from dry beds (mean ± SE = 209 ± 10 mmol CO2 m−2 d−1) was comparable to that from running waters (120 ± 33 mmol m−2 d−1) and significantly higher than from impounded waters (36.6 ± 8.5 mmol m−2 d−1) and isolated pools (17.2 ± 0.9 mmol m−2 d−1). In contrast, the CH4 efflux did not significantly differ among environments, although the CH4 efflux was notable in some impounded waters (13.9 ± 10.1 mmol CH4 m−2 d−1) and almost negligible in the remaining environments (mean <0.3 mmol m−2 d−1). Diffusion was the only mechanism driving CO2 efflux in all environments and was most likely responsible for CH4 efflux in running waters, isolated pools and dry beds. In contrast, the CH4 efflux in impounded waters was primarily ebullition-based. Using a simple heuristic approach to simulate potential changes in carbon emissions from Mediterranean fluvial networks under future hydrological scenarios, we show that an extreme drying out (i.e., a four-fold increase of the surface area of dry beds) would double the CO2 efflux from the fluvial network. Correspondingly, an extreme transformation of running waters into impounded waters (i.e., a twofold increase of the surface area of impounded waters) would triple the CH4 efflux. Thus, carbon emissions from dry beds and impounded waters should be explicitly considered in carbon assessments of fluvial networks, particularly under predicted global change scenarios, which are expected to increase the spatial and temporal extent of these environments.





Similar content being viewed by others
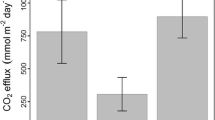
References
Abril G (2005) Carbon dioxide and methane emissions and the carbon budget of a 10-year old tropical reservoir (Petit Saut, French Guiana). Global Biogeochem Cycles 19:1–16. doi:10.1029/2005GB002457
Acuña V, Tockner K (2010) The effects of alterations in temperature and flow regime on organic carbon dynamics in Mediterranean river networks. Global Chang Biol 16:2638–2650. doi:10.1111/j.1365-2486.2010.02170.x
Acuña V, Datry T, Marshall J et al (2014) Why should we care about temporary waterways? Science 343:1080–1082. doi:10.1126/science.1246666
Alin SR, Rasera MDFFL, Salimon CI et al (2011) Physical controls on carbon dioxide transfer velocity and flux in low-gradient river systems and implications for regional carbon budgets. J Geophys Res 116:G01009. doi:10.1029/2010JG001398
Angert A, Yakir D, Rodeghiero M et al (2014) Using O2 to study the relationships between soil CO2 efflux and soil respiration. Biogeosciences Discuss 11:12039–12068. doi:10.5194/bgd-11-12039-2014
Austin AT, Vivanco L (2006) Plant litter decomposition in a semi-arid ecosystem controlled by photodegradation. Nature 442:555–558. doi:10.1038/nature05038
Bade DL (2009) Gas exchange across the air-water interface. In: Gene EL (ed) Encyclopedia of inland waters. Academic Press, Oxford, pp 70–78
Bastien J, Demarty M (2013) Spatio-temporal variation of gross CO2 and CH4 diffusive emissions from Australian reservoirs and natural aquatic ecosystems, and estimation of net reservoir emissions. Lakes Reserv Res Manag 18:115–127. doi:10.1111/lre.12028
Bastviken D, Cole J, Pace M, Tranvik LJ (2004) Methane emissions from lakes: dependence of lake characteristics, two regional assessments, and a global estimate. Global Biogeochem Cycles 18:1–12. doi:10.1029/2004GB002238
Bastviken D, Tranvik LJ, Downing JA et al (2011) Freshwater methane emissions offset the continental carbon sink. Science 331:50–57. doi:10.1126/science.1196808
Battin TJ, Luyssaert S, Kaplan LA et al (2009a) The boundless carbon cycle. Nat Geosci 2:598–600. doi:10.1038/ngeo618
Battin TJ, Kaplan LA, Findlay S et al (2009b) Biophysical controls on organic carbon fluxes in fluvial networks. Nat Geosci 2:95–100. doi:10.1038/ngeo602
Baulch HM, Dillon PJ, Maranger R, Schiff SL (2011) Diffusive and ebullitive transport of methane and nitrous oxide from streams: are bubble-mediated fluxes important? J Geophys Res Biogeosciences 116:1–15. doi:10.1029/2011JG001656
Beaulieu JJ, Shuster WD, Rebholz JA (2012) Controls on gas transfer velocities in a large river. J Geophys Res Biogeosciences 117:1–13. doi:10.1029/2011JG001794
Belger L, Forsberg BR, Melack JM (2010) Carbon dioxide and methane emissions from interfluvial wetlands in the upper Negro River basin, Brazil. Biogeochemistry 105:171–183. doi:10.1007/s10533-010-9536-0
Benstead JP, Leigh DS (2012) An expanded role for river networks. Nat Geosci 5:678–679. doi:10.1038/ngeo1593
Bernal S, von Schiller D, Sabater F, Martí E (2013) Hydrological extremes modulate nutrient dynamics in mediterranean climate streams across different spatial scales. Hydrobiologia 719:31–42. doi:10.1007/s10750-012-1246-2
Bonada N, Resh VH (2013) Mediterranean-climate streams and rivers: geographically separated but ecologically comparable freshwater systems. Hydrobiologia 719:1–29. doi:10.1007/s10750-013-1634-2
Bond-Lamberty B, Thomson A (2010) A global database of soil respiration data. Biogeosciences 7:1915–1926. doi:10.5194/bg-7-1915-2010
Campbell C, Chapman S (2003) A rapid microtiter plate method to measure carbon dioxide evolved from carbon substrate amendments so as to determine the physiological profiles of soil microbial. Appl Environ Microbiol 69:3593–3599. doi:10.1128/AEM.69.6.3593
Campeau A, Del Giorgio PA (2014) Patterns in CH4 and CO2 concentrations across boreal rivers: major drivers and implications for fluvial greenhouse emissions under climate change scenarios. Glob Chang Biol 20:1–14. doi:10.1111/gcb.12479
Campeau A, Lapierre J (2014) Regional contribution of CO2 and CH4 fluxes from the fluvial network in a lowland boreal landscape of Québec. Glob Biogeochem Cycles 28:1–13. doi:10.1002/2013GB004685
Cole JJ, Prairie YT, Caraco NF et al (2007) Plumbing the global carbon cycle: integrating inland waters into the terrestrial carbon budget. Ecosystems 10:172–185. doi:10.1007/s10021-006-9013-8
Cole JJ, Bade DL, Bastviken D et al (2010) Multiple approaches to estimating air-water gas exchange in small lakes. Limnol Oceanogr Methods 8:285–293. doi:10.4319/lom.2010.8.285
Crawford JT, Stanley EH, Spawn SA et al (2014) Ebullitive methane emissions from oxygenated wetland streams. Global Chang Biol 20:3408–3422. doi:10.1111/gcb.12614
Crusius J, Wanninkhof R (2003) Gas transfer velocities measured at low wind speed over a lake. Limnol Oceanogr 48:1010–1017. doi:10.4319/lo.2003.48.3.1010
Dahm CN, Baker MA, Moore DI, Thibault JR (2003) Coupled biogeochemical and hydrological responses of streams and rivers to drought. Freshw Biol 48:1219–1231. doi:10.1046/j.1365-2427.2003.01082.x
Datry T, Larned ST, Tockner K (2014) Intermittent rivers: a challenge for freshwater ecology. Bioscience 64:229–235. doi:10.1093/biosci/bit027
Dean WE (1974) Determination of carbonate and organic matter in calcareous sediments and sedimentary rocks by loss on ignition: comparison with other method. J Sediment Petrol 44:242–248. doi:10.1306/74D729D2-2B21-11D7-8648000102C1865D
Del Sontro T (2011) Quantifying methane emissions from reservoirs: from Basin-scale to discrete analyses with a focus on ebullition dynamics. PhD dissertation, Eidgenössische Technische Hochschule ETH Zürich, Zürich. doi: http://dx.doi.org/10.3929/ethz-a-006725547
Del Sontro T, McGinnis DF, Sobek S et al (2010) Extreme methane emissions from a Swiss hydropower reservoir: contribution from bubbling sediments. Environ Sci Technol 44:2419–2425. doi:10.1021/es9031369
Demars BOL, Manson JR (2013) Temperature dependence of stream aeration coefficients and the effect of water turbulence: a critical review. Water Res 47:1–15. doi:10.1016/j.watres.2012.09.054
Donelan MA (1990) Air–sea interaction. In: LeMehaute B, Hanes D (eds) The sea: ocean engineering science. Wiley, New York, pp 239–292
Downing JA, Cole JJ, Middelburg JJ et al (2008) Sediment organic carbon burial in agriculturally eutrophic impoundments over the last century. Global Biogeochem Cycles 22:GB1018. doi:10.1029/2006GB002854
Fearnside PM, Pueyo S (2012) Greenhouse-gas emissions from tropical dams. Nat Clim Chang 2:382–384. doi:10.1038/nclimate1540
Fellman JB, Hood E, Spencer RGM (2010) Fluorescence spectroscopy opens new windows into dissolved organic matter dynamics in freshwater ecosystems: a review. Limnol Oceanogr 55:2452–2462. doi:10.4319/lo.2010.55.6.2452
Forzieri G, Feyen L, Rojas R et al (2014) Ensemble projections of future streamflow droughts in Europe. Hydrol Earth Syst Sci 18:85–108. doi:10.5194/hess-18-85-2014
Frankignoulle M (1988) Field measurements of air-sea CO2 exchange. Limnol Ocean 33:313–322
Fujikawa T, Miyazaki T (2005) Effects of bulk density and soil type on the gas diffusion coefficient in repacked and undisturbed soils. Soil Sci 170:892–901. doi:10.1097/01.ss.0000196771.53574.79
Gallo EL, Lohse KA, Ferlin CM et al (2014) Physical and biological controls on trace gas fluxes in semi-arid urban ephemeral waterways. Biogeochemistry 121:189–207. doi:10.1007/s10533-013-9927-0
García-Ruiz JM, López-Moreno JI, Vicente-Serrano SM et al (2011) Mediterranean water resources in a global change scenario. Earth-Sci Rev 105:121–139. doi:10.1016/j.earscirev.2011.01.006
Gasith A, Resh VH (1999) Streams in mediterranean climate regions: abiotic influences and biotic responses to predictable seasonal events. Annu Rev Ecol Syst 30:51–81. doi:10.1146/annurev.ecolsys.30.1.51
Guérin F, Abril G, Serça D et al (2007) Gas transfer velocities of CO2 and CH4 in a tropical reservoir and its river downstream. J Mar Syst 66:161–172. doi:10.1016/j.jmarsys.2006.03.019
Halbedel S, Koschorreck M (2013) Regulation of CO2 emissions from temperate streams and reservoirs. Biogeosciences 10:7539–7551. doi:10.5194/bg-10-7539-2013
Hope D, Palmer SM, Billett MF, Dawson JJC (2001) Carbon dioxide and methane evasion from a temperate peatland stream. Limnol Oceanogr 46:847–857. doi:10.4319/lo.2001.46.4.0847
Hornberger GM, Kelly MG (1975) Atmospheric reaeration in a river using productivity analysis. J Environ Eng Div ASCE 101:729–739
Howard D, Howard P (1993) Relationships between CO2 evolution, moisture content and temperature for a range of soil types. Soil Biol Biochem 25:1537–1546. doi:10.1016/0038-0717(93)90008-Y
IPCC 2013: Climate Change 2013: The physical science basis. Contribution of Working Group I to the fifth assessment report of the Intergovernmental Panel on climate change. Stocker TF, Qin D, Plattner G-K, Tignor M, Allen SK, Boschung J, Nauels A, Xia Y, Bex V, Midgley PM (eds). Cambridge University Press, Cambridge and New York, 1535 pp
Jähne B, Münnich K (1987) On the parameters influencing air-water gas exchange. J Geophys Res Ocean 92:1937–1942. doi:10.1029/JC092iC02p01937
Jonsson A, Algesten G, Bergström AK et al (2007) Integrating aquatic carbon fluxes in a boreal catchment carbon budget. J Hydrol 334:141–150. doi:10.1016/j.jhydrol.2006.10.003
Kling GW, Kipphut GW, Miller MC (1991) Arctic lakes and streams as gas conduits to the atmosphere: implications for tundra carbon budgets. Science 251(4991):298–301. doi:10.1126/science.251.4991.298
Koschorreck M, Darwich A (2003) Nitrogen dynamics in seasonally flooded soils in the Amazon floodplain. Wetl Ecol Manag 11:317–330. doi:10.1023/B:WETL.0000005536.39074.72
Lake PS (2011) Drought and aquatic ecosystems: effects and responses. Wiley-Blackwell, Chichester
Larned ST, Datry T, Arscott DB, Tockner K (2010) Emerging concepts in temporary-river ecology. Freshw Biol 55:717–738. doi:10.1111/j.1365-2427.2009.02322.x
Laurion I, Vincent W, MacIntyre S (2010) Variability in greenhouse gas emissions from permafrost thaw ponds. Limnol Oceanogr 55:115–133. doi:10.4319/lo.2010.55.1.0115
López P, Marcé R, Armengol J (2011) Net heterotrophy and CO2 evasion from a productive calcareous reservoir: adding complexity to the metabolism-CO2 evasion issue. J Geophys Res Biogeosciences 116:G02021. doi:10.1029/2010JG001614
Lundin EJ, Giesler R, Persson A et al (2013) Integrating carbon emissions from lakes and streams in a subarctic catchment. J Geophys Res Biogeosciences 118:1–8. doi:10.1002/jgrg.20092
Luo Y, Zhou X (2010) Soil respiration and the environment. Elsevier Academy Press, Amsterdam
Maeck A, Del Sontro T, McGinnis DF et al (2013) Sediment trapping by dams creates methane emission hot spots. Environ Sci Technol 47:8130–8137. doi:10.1021/es4003907
McGinnis DF, Kirillin G, Tang KW et al (2015) Enhancing surface methane fluxes from an Oligotrophic lake: exploring the microbubble hypothesis. Environ Sci Technol 49:873–880. doi:10.1021/es503385d
McIntyre RES, Adams MA, Ford DJ, Grierson PF (2009) Rewetting and litter addition influence mineralisation and microbial communities in soils from a semi-arid intermittent stream. Soil Biol Biochem 41:92–101. doi:10.1016/j.soilbio.2008.09.021
Meier JA, Jewell JS, Brennen CE, Imberger J (2011) Bubbles emerging from a submerged granular bed. J Fluid Mech 666:189–203. doi:10.1017/S002211201000443X
Meybeck M, Dürr H, Vörösmarty C (2006) Global coastal segmentation and its river catchment contributors: A new look at land-ocean linkage. Global Biogeochem Cycles 20:GB1S90. doi:10.1029/2005GB002540
Millero F (1995) Thermodynamics of the carbon dioxide system in the oceans. Geochim Cosmochim Acta 59:661–677. doi:10.1016/0016-7037(94)00354-O
Milliman JD, Farnsworth KL, Jones PD et al (2008) Climatic and anthropogenic factors affecting river discharge to the global ocean, 1951–2000. Glob Planet Chang 62:187–194. doi:10.1016/j.gloplacha.2008.03.001
Mitchell AM, Baldwin DS (1999) The effects of sediment desiccation on the potential for nitrification, denitrification, and methanogenesis in an Australian reservoir. Hydrobiologia 392:3–11. doi:10.1023/A:1003589805914
Morales-Pineda M, Cózar A, Laiz I et al (2014) Daily, biweekly, and seasonal temporal scales of pCO2 variability in two stratified Mediterranean reservoirs. J Geophys Res Biogeosciences 119:1–12. doi:10.1002/2013JG002317
Mulholland PJ, Elwood JW (1982) The role of lake and reservoir sediments as sinks in the perturbed global carbon cycle. Tellus A 34:490–499. doi:10.3402/tellusa.v34i5.10834
Nilsson C, Reidy C, Dynesius M, Revenga C (2005) Fragmentation and flow regulation of the world’s large river systems. Science 308:405–408. doi:10.1126/science.1107887
Obrador B, Pretus JL (2012) Budgets of organic and inorganic carbon in a Mediterranean coastal lagoon dominated by submerged vegetation. Hydrobiologia 699:35–57. doi:10.1007/s10750-012-1152-7
Oksanen, J, Blanchet FG, Kindt R et al. (2013). Vegan: community ecology package. R package version 2.0-10. http://CRAN.R-project.org/package=vegan
Pavón D (2010) Desarrollo y decadencia hidroeléctrica en los pequeños ríos del litoral mediterráneo catalán. El caso de las cuencas del Fluvià y de la Muga. Revista de Historia Industrial 42:43–87
Pohlon E, Ochoa Fandino A, Marxsen J (2013) Bacterial community composition and extracellular enzyme activity in temperate streambed sediment during drying and rewetting. PLoS One 8:e83365. doi:10.1371/journal.pone.0083365
Prairie Y, Del Giorgio PA (2013) A new pathway of freshwater methane emissions and the putative importance of microbubbles. Inl Waters 3:311–320. doi:10.5268/IW-3.3.542
R Core Team (2013). R: a language and environment for statistical computing. R Foundation for Statistical Computing, Vienna. ISBN:3-900051-07-0, http://www.R-project.org/
Raich J, Schlesinger W (1992) The global carbon dioxide flux in soil respiration and its relationship to vegetation and climate. Tellus B 44:81–99. doi:10.1034/j.1600-0889.1992.t01-1-00001.x
Raich J, Potter C, Bhagawati D (2002) Interannual variability in global soil respiration, 1980–94. Global Chang Biol 8:800–812. doi:10.3334/CDIAC/lue.ndp081
Raymond PA, Hartmann J, Lauerwald R et al (2013) Global carbon dioxide emissions from inland waters. Nature 503:355–359. doi:10.1038/nature12760
Rey A (2015) Mind the gap: non-biological processes contributing to soil CO2 efflux. Global Chang Biol 21:1752–1761. doi:10.1111/gcb.12821
Sabater S (2008) Alterations of the global water cycle and their effects on river structure, function and services. Freshw Rev 1:75–88. doi:10.1608/FRJ-1.1.5
Sobek S, Algesten G (2003) The catchment and climate regulation of pCO2 in boreal lakes. Global Chang Biol 9:630–641. doi:10.1046/j.1365-2486.2003.00619.x
Sobek S, Zurbrügg R, Ostrovsky I (2011) The burial efficiency of organic carbon in the sediments of Lake Kinneret. Aquat Sci 73:355–364. doi:10.1007/s00027-011-0183-x
Sobek S, Del Sontro T, Wongfun N, Wehrli B (2012) Extreme organic carbon burial fuels intense methane bubbling in a temperate reservoir. Geophys Res Lett 39:2–5. doi:10.1029/2011GL050144
Steward AL, von Schiller D, Tockner K et al (2012) When the river runs dry: human and ecological values of dry riverbeds. Front Ecol Environ 10:202–209. doi:10.1890/110136
Striegl RG, Dornblaser MM, McDonald CP et al (2012) Carbon dioxide and methane emissions from the Yukon River system. Global Biogeochem Cycles 26:GB0E05. doi:10.1029/2012GB004306
Stumm W, Morgan JJ (1996) Aquatic chemistry: chemical equilibria and rates in natural waters. Wiley, Hoboken
Tang KW, McGinnis DG, Frindte K et al (2014) Paradox reconsidered: methane oversaturation in well-oxygenated lake waters. Limnol Oceanogr 59:275–284. doi:10.4319/lo.2014.59.1.0275
Teodoru CR, Prairie YT, Del Giorgio PA (2010) Spatial Heterogeneity of Surface CO2 Fluxes in a newly created eastmain-1 reservoir in Northern Quebec, Canada. Ecosystems 14:28–46. doi:10.1007/s10021-010-9393-7
Timoner X, Acuña V, Frampton L et al (2014) Biofilm functional responses to the rehydration of a dry intermittent stream. Hydrobiologia 727:185–195. doi:10.1007/s10750-013-1802-4
Tockner K, Uehlinger U, Robinson CT (2009) Rivers of Europe. Academic Press, San Diego
Tooth S (2000) Process, form and change in dry land rivers: a review of recent research. Earth Sci Rev 51:67–107. doi:10.1016/S0012-8252(00)00014-3
Vachon D, Prairie YT, Cole JJ (2010) The relationship between near-surface turbulence and gas transfer velocity in freshwater systems and its implications for floating chamber measurements of gas exchange. Limnol Oceanogr 55:1723–1732. doi:10.4319/lo.2010.55.4.1723
Vazquez E, Amalfitano S, Fazi S, Butturini A (2010) Dissolved organic matter composition in a fragmented Mediterranean fluvial system under severe drought conditions. Biogeochemistry 102:59–72. doi:10.1007/s10533-010-9421-x
Von Schiller D, Acuña V, Graeber D et al (2011) Contraction, fragmentation and expansion dynamics determine nutrient availability in a Mediterranean forest stream. Aquat Sci 73:485–497. doi:10.1007/s00027-011-0195-6
Von Schiller D, Marcé R, Obrador B et al (2014) Carbon dioxide emissions from dry watercourses. Inland waters 4:377–382. doi:10.5268/IW-4.4.746
Vörösmarty CJ, McIntyre PB, Gessner MO et al (2010) Global threats to human water security and river biodiversity. Nature 467:555–561. doi:10.1038/nature09440
Wanninkhof R (1992) Relationship between wind speed and gas exchange over the ocean. J Geophys Res Ocean 97:7373–7382. doi:10.1029/92JC00188
Weiss R (1974) Carbon dioxide in water and seawater: the solubility of a non-ideal gas. Mar Chem 2:203–215. doi:10.1016/0304-4203(74)90015-2
Williams DD (2006) The biology of temporary waters. Oxford University Press, Oxford
Xiao S, Liu D, Wang Y et al (2013) Temporal variation of methane flux from Xiangxi Bay of the three gorges reservoir. Sci Rep 3:2500. doi:10.1038/srep02500
Xu L, Baldocchi DD, Tang J (2004) How soil moisture, rain pulses, and growth alter the response of ecosystem respiration to temperature. Global Biogeochem Cycles 18:GB4002. doi:10.1029/2004GB002281
Zoppini A, Marxsen J (2011) Importance of extracellular enzymes for biogeochemical processes in temporary river sediments during fluctuating dry-wet Conditions. In: Shukla G, Varma A (eds) Soil enzymology. Springer, Berlin, pp 103–117
Acknowledgments
All the data used for the results of this paper is available upon request to the corresponding author. We thank P. A. Raymond for providing data of the effective area occupied by dry rivers in our COSCAT region. This research was funded by the Spanish Ministry of Economy and Competitiveness through the projects CGL2011-30474-C02-01 and CGL2014-58760-C3-1-R. Ll. Gómez-Gener and J. P. Casas-Ruiz were additionally supported by FPI predoctoral grants (BES-2012-059743 and BES-2012-059655) and D. von Schiller by a “Juan de la Cierva” postdoctoral grant (JCI-2010-06397). We thank Carmen Gutiérrez, Mertixell Abril and Susanne Halbedel, for the lab and field assistance. We also thank the Scientific and Technical Service Department of the Catalan Institute for Water research (ICRA) for the lab assistance.
Author information
Authors and Affiliations
Corresponding author
Additional information
Responsible Editor: Jacques C Finlay.
Electronic supplementary material
Below is the link to the electronic supplementary material.
Rights and permissions
About this article
Cite this article
Gómez-Gener, L., Obrador, B., von Schiller, D. et al. Hot spots for carbon emissions from Mediterranean fluvial networks during summer drought. Biogeochemistry 125, 409–426 (2015). https://doi.org/10.1007/s10533-015-0139-7
Received:
Accepted:
Published:
Issue Date:
DOI: https://doi.org/10.1007/s10533-015-0139-7