Abstract
Tautomerism in monomers/dimers and association of 2,5-dihydroxy-1,8-naphthyridine was studied at the DFT level recently recommended for studies of non-covalent interactions. Studied dimers are stabilized by double and triple hydrogen-bonding. In some associates the intermolecular proton transfer may take place. Transition state related to the double proton transfer reactions were calculated and discussed in terms of energetics, changes in atomic charges upon association, aromaticity (HOMA), properties of hydrogen bond critical point (QTAIM methodology) and geometry change during this reaction. It was found that double proton transfer is supported by third hydrogen bond or by weak secondary interaction. Some protons in transition states are shared between two basic atoms, while other are covalently bound only to one of them. The said process leads to replacement of secondary interactions of attractive character to repulsive and vice versa. Overall, results suggest that in subjected compound the triple hydrogen-bonded associate may be in equilibrium with double hydrogen-bonded dimer.






Similar content being viewed by others
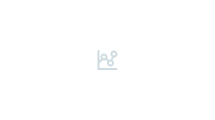
References
Desiraju GR (2011) Reflections on the hydrogen bond in crystal engineering. Cryst Growth Des 11:896–898. doi:10.1021/cg200100m
Desiraju GR (2002) Hydrogen bridges in crystal engineering: Interactions without borders. Acc Chem Res 35:565–573. doi:10.1021/ar010054t
Desiraju GR (1995) Supramolecular synthons in crystal engineering. A new organic synthesis. Angew Chem Int Ed 34:2311–2327
Moulton B, Zaworotko MJ (2001) From molecules to crystal engineering: Supramolecular isomerism and polymorphism in network solids. Chem Rev 101:1629–1658. doi:10.1021/cr9900432
Eckert J, Barthes M, Klooster WT, Albinati A, Aznar R, Koetzle TF (2000) No evidence for proton transfer along the N-H…O hydrogen bond in N-methylacetamide: Neutron single crystal structure at 250 and 276 k. J Phys Chem B 105:19–24. doi:10.1021/jp000267k
Katz JL, Post B (1960) The crystal structure and polymorphisc of n-methylacetamide. Acta Cryst 13:624–628
Adarsh NN, Sahoo P, Dastidar P (2010) Is a crystal engineering approach useful in designing metallogels? A case study. Cryst Growth Des 10:4976–4986. doi:10.1021/cg101078f
Paisner K, Zakharov LN, Doxsee KM A robust thiourea synthon for crystal engineering. Cryst Growth Des 10:3757–3762. doi:10.1021/cg100589n
Burrows AD (2004) Crystal engineering using multiple hydrogen bonds. In: Mingos DMP (ed) Supramolecular assembly via hydrogen bonds. Springer, Berlin / Heidelberg, pp 55–96
Smith G, Lynch D, Byriel K, Kennard C (1997) The utility of 4-aminobenzoic acid in promotion of hydrogen bonding in crystallization processes: The structures of the cocrystals with halo and nitro subsituted aromatic compounds, and the crystal structures of the adducts with 4-nitroaniline (1:1), 4-(4-nitrobenzyl)pyridine (1:2), and (4-nitrophenyl)acetic acid (1:1). J Chem Crystallogr 27:307–317. doi:10.1007/bf02575979
Auffinger P, Hays FA, Westhof E, Ho PS (2004) Halogen bonds in biological molecules. Proc Natl Acad Sci USA 101:16789–16794. doi:10.1073/pnas.0407607101
Aakeröy CB, Schultheiss NC, Rajbanshi A, Desper J, Moore C (2008) Supramolecular synthesis based on a combination of hydrogen and halogen bonds. Cryst Growth Des 9:432–441. doi:10.1021/cg8006712
Politzer P, Murray JS, Clark T (2010) Halogen bonding: An electrostatically-driven highly directional noncovalent interaction. Phys Chem Chem Phys 12:7748–7757
Prasang C, Nguyen HL, Horton PN, Whitwood AC, Bruce DW (2008) Trimeric liquid crystals assembled using both hydrogen and halogen bonding. Chem Comm:6164–6166
Rissanen K (2008) Halogen bonded supramolecular complexes and networks. Cryst Eng Comm 10:1107–1113
Ritter SK (2009) Halogen bonding begins to fly. Chem Eng News 87:39–42
Voth AR, Khuu P, Oishi K, Ho PS (2009) Halogen bonds as orthogonal molecular interactions to hydrogen bonds. Nature 1:74–79
Berl V, Krische MJ, Huc I, Lehn JM, Schmutz M (2000) Template-induced and molecular recognition directed hierarchical generation of supramolecular assemblies from molecular strands. Chem Eur J 6:1938–1946
van Beek DJM, Spiering AJH, Peters GWM, te Nijenhuis K, Sijbesma RP (2007) Unidirectional dimerization and stacking of ureidopyrimidinone end groups in polycaprolactone supramolecular polymers. Macromolecules 40:8464–8475. doi:10.1021/ma0712394
Richards FM, Kundrot CE (1988) Identification of structural motifs from protein coordinate data: Secondary structure and first-level supersecondary structure. Proteins Struct Funct Genet 3:71–84
Kabsch W, Sander C (1983) Dictionary of protein secondary structure: Pattern recognition of hydrogen-bonded and geometrical features. Biopolymers 22:2577–2637
Watson JD, Crick FHC (1953) Molecular structure of nucleic acids: A structure for deoxyribose nucleic acid. Nature 171:737–738
Watson JD, Crick FHC (1953) Genetical implications of the structure of deoxyribonucleic acid. Nature 171:964–967
Jeffery GA, Saenger W (1994) Hydrogen bonding in proteins. In: Hydrogen bonding in biological structures. Springer-Verlag, Berlin, pp 351–394
de Groot BL, Daura X, Mark AE, Grubmüller H (2001) Essential dynamics of reversible peptide folding: Memory-free conformational dynamics governed by internal hydrogen bonds. J Mol Biol 309:299–313
Daura X, van Gunsteren WF, Rigo D, Jaun B, Seebach D (1997) Studying the stability of a helical β-heptapeptide by molecular dynamics simulations. Chem Eur J 3:1410–1417. doi:10.1002/chem.19970030907
Daura X, Jaun B, Seebach D, van Gunsteren WF, Mark AE (1998) Reversible peptide folding in solution by molecular dynamics simulation. J Mol Biol 280:925–932
Suárez M, Lehn J-M, Zimmerman SC, Skoulios A, Heinrich B (1998) Supramolecular liquid crystals. Self-assembly of a trimeric supramolecular disk and its self-organization into a columnar discotic mesophase. J Am Chem Soc 120:9526–9532
Ośmiałowski B, Kolehmainen E, Dobosz R, Gawinecki R, Kauppinen R, Valkonen A, Koivukorpi J, Rissanen K (2010) Self-organization of 2-acylaminopyridines in the solid state and in solution. J Phys Chem A 114:10421–10426
Lehn J-M (2002) Toward self-organization and complex matter. Science 295:2400–2403. doi:10.1126/science.1071063
Kuykendall DW, Anderson CA, Zimmerman SC (2009) Hydrogen-bonded DeUG–DAN heterocomplex: Structure and stability and a scalable synthesis of deug with reactive functionality. Org Lett 11:61–64. doi:10.1021/ol802344w
Hisamatsu Y, Fukumi Y, Shirai N, S-i I, Odashima K (2008) Five-membered heterocyclic ureas suitable for the donor-donor-acceptor hydrogen-bonding modules. Tetrahedron Lett 49:2005–2009
Hisamatsu Y, Shirai N, Ikeda S-I, Odashima K (2010) A new quadruple hydrogen-bonding module based on five-membered heterocyclic urea structure. Org Lett 12:1776–1779. doi:10.1021/ol100385b
Ong HC, Zimmerman SC (2006) Higher affinity quadruply hydrogen-bonded complexation with 7-deazaguanine urea. Org Lett 8:1589–1592. doi:10.1021/ol0601803
Mayer MF, Nakashima S, Zimmerman SC (2005) Synthesis of a soluble ureido-naphthyridine oligomer that self-associates via eight contiguous hydrogen bonds. Org Lett 7:3005–3008
Jorgensen WL, Pranata J (1990) Importance of secondary interactions in triply hydrogen bonded complexes: Guanine-cytosine vs uracil-2,6-diaminopyridine. J Am Chem Soc 112:2008–2010
Ośmiałowski B, Dobosz R The influence of secondary interactions on complex stability and double proton transfer reaction in 2-[1H]-pyridone/2-hydroxypyridine dimers. J Mol Model. doi:10.1007/s00894-010-0934-y
Ośmiałowski B (2009) Systematic investigation of 2,7-dihydroxy-1,8-naphthyridine dimerization - secondary interactions and tautomeric preferences calculations. J Mol Struct THEOCHEM 908:92–101
Lüning U, Kühl C, Uphoff A (2002) Four hydrogen bonds - DDAA, DADA, DAAD and ADDA hydrogen bond motifs. Eur J Org Chem 2002:4063–4070
Szyc Ł, Guo J, Yang M, Dreyer J, Tolstoy PM, Nibbering ETJ, Czarnik-Matusewicz B, Elsaesser T, Limbach H-H (2010) The hydrogen-bonded 2-pyridone dimer model system. 1. Combined NMR and FT-IR spectroscopy study. J Phys Chem A 114:7749–7760. doi:10.1021/jp103630w
Yang M, Szyc Ł, Dreyer J, Nibbering ETJ, Elsaesser T (2010) The hydrogen-bonded 2-pyridone dimer model system. 2. Femtosecond mid-infrared pump-probe study. J Phys Chem A 114:12195–12201. doi:10.1021/jp108096y
Müller A, Losada M, Leutwyler S (2004) Ab initio benchmark study of (2-pyridone)2, a strongly bound doubly hydrogen-bonded dimer. J Phys Chem A 108:157–165
Alvares-Rua C, Garcia-Granda S, Goswami S, Mukherjee R, Dey S, Claramunt RM, Santa Maria MD, Rozas I, Jagerovic N, Alkorta I, Elguero J (2004) Multiple hydrogen bonds and tautomerism in naphthyridine derivatives. New J Chem 28:700–707
Newman SG, Taylor A, Boyd RJ (2008) Factors controlling extremely strong AAA-DDD triply hydrogen-bonded complexes. Chem Phys Lett 450:210–213
Blight BA, Camara-Campos A, Djurdjevic S, Kaller M, Leigh DA, McMillan FM, McNab H, Slawin AMZ (2009) AAA-DDD triple hydrogen bond complexes. J Am Chem Soc 131:14116–14122. doi:10.1021/ja906061v
Ośmiałowski B, Kolehmainen E, Gawinecki R, Dobosz R, Kauppinen R (2010) Complexation of 2,6-bis(acylamino)pyridines with dipyridin-2-ylamine and 4,4-dimethylpiperidine-2,6-dione. J Phys Chem A 114:12881–12887
Ośmiałowski B, Kolehmainen E, Gawinecki R, Kauppinen R, Koivukorpi J, Valkonen A (2010) Nmr and quantum chemical studies on association of 2,6-bis(acylamino)pyridines with selected imides and 2,2'-dipyridylamine. Struct Chem 21:1061–1067. doi:10.1007/s11224-010-9646-2
Chien C-H, Leung M-k SuJ-K, Li G-H, Liu Y-H, Wang Y (2004) Substituent effects on pyrid-2-yl ureas toward intramolecular hydrogen bonding and cytosine complexation. J Org Chem 69:1866–1871. doi:10.1021/jo0355808
Sartorius J, Schneider H-J (1996) A general scheme based on empirical increments for the prediction of hydrogen-bond associations of nucleobases and of synthetic host-guest complexes. Chem Eur J 2:1446–1452
Frisch MJ, Trucks GW, Schlegel HB, Scuseria GE, Robb MA, Cheeseman JR, Montgomery JA, Vreven T, Kudin KN, Burant JC, Millam JM, Iyengar SS, Tomasi J, Barone V, Mennucci B, Cossi M, Scalmani G, Rega N, Petersson GA, Nakatsuji H, Hada M, Ehara M, Toyota K, Fukuda R, Hasegawa J, Ishida M, Nakajima T, Honda Y, Kitao O, Nakai H, Klene M, Li X, Knox JE, Hratchian HP, Cross JB, Adamo C, Jaramillo J, Gomperts R, Stratmann RE, Yazyev O, Austin AJ, Cammi R, Pomelli C, Ochterski JW, Ayala PY, Morokuma K, Voth GA, Salvador P, Dannenberg JJ, Zakrzewski VG, Dapprich S, Daniels AD, Strain MC, Farkas O, Malick DK, Rabuck AD, Raghavachari K, Foresman JB, Ortiz JV, Cui Q, Baboul AG, Clifford S, Cioslowski J, Stefanov BB, Liu G, Liashenko A, Piskorz P, Komaromi I, Martin RL, Fox DJ, Keith T, Al-Laham MA, Peng CY, Nanayakkara A, Challacombe M, Gill PMW, Johnson B, Chen W, Wong MW, Gonzalez C, Pople JA (2004) Gaussian 03, Revision E.01. Gaussian Inc, Pittsburgh PA
Zhao Y, Schultz NE, Truhlar DG (2005) Exchange-correlation functional with broad accuracy for metallic and nonmetallic compounds, kinetics, and noncovalent interactions. J Chem Phys 123:161103–161104
Zhao Y, Truhlar DG (2006) Assessment of model chemistries for noncovalent interactions. J Chem Theor Comput 2:1009–1018
Zhao Y, Truhlar DG (2008) Exploring the limit of accuracy of the global hybrid meta density functional for main-group thermochemistry, kinetics, and noncovalent interactions. J Chem Theor Comput 4:1849–1868. doi:10.1021/ct800246v
Zhao Y, Truhlar DG (2008) The m06 suite of density functionals for main group thermochemistry, thermochemical kinetics, noncovalent interactions, excited states, and transition elements: Two new functionals and systematic testing of four m06-class functionals and 12 other functionals. Theor Chem Acc 120:215–241
Ditchfield R, Hehre WJ, Pople JA (1971) Self-consistent molecular-orbital methods. Ix. An extended gaussian-type basis for molecular-orbital studies of organic molecules. J Chem Phys 54:724–728
Hehre WJ, Ditchfield R, Pople JA (1972) Self-consistent molecular orbital methods. XII. Further extensions of gaussian-type basis sets for use in molecular orbital studies of organic molecules. J Chem Phys 56:2257–2261
Francl MM, Pietro WJ, Hehre WJ, Binkley JS, Gordon MS, DeFrees DJ, Pople JA (1982) Self-consistent molecular orbital methods. XXIII. A polarization-type basis set for second-row elements. J Chem Phys 77:3654–3665
Peng C, Schlegel HB (1993) Combining synchronous transit and quasi-newton methods to find transition states. Isr J Chem 33:449–454
Boys SF, Bernardi F (1970) The calculation of small molecular interactions by the differences of separate total energies. Some procedures with reduced errors. Mol Phys 19:553–566. doi:10.1080/00268977000101561
Simon S, Duran M, Dannenberg JJ (1996) How does basis set superposition error change the potential surfaces for hydrogen-bonded dimers? J Chem Phys 105:11024–11031
Foster JP, Weinhold F (1980) Natural hybrid orbitals. J Am Chem Soc 102:7211–7218. doi:10.1021/ja00544a007
Reed AE, Weinhold F (1983) Natural bond orbital analysis of near-hartree–fock water dimer. J Chem Phys 78:4066–4073
Biegler-König F, Schönbohm J, Bayles D (2001) AIM2000. J Comp Chem 22:545–559. doi:10.1002/1096-987x(20010415)22:5<545::aid-jcc1027>3.0.co;2-y
Kruszewski J, Krygowski TM (1972) Definition of aromaticity basing on the harmonic oscillator model. Tetrahedron Lett 13:3839–3842
Krygowski TM (1993) Crystallographic studies of inter- and intramolecular interactions reflected in aromatic character of π-electron systems. J Chem Inf Comput Sci 33:70–78
Kolehmainen E, Ośmiałowski B, Krygowski TM, Kauppinen R, Nissinen M, Gawinecki R (2000) J Chem Soc Perkin Trans 2:1259–1266
Kolehmainen E, Ośmiałowski B, Nissinen M, Kauppinen R, Gawinecki R (2000) J Chem Soc Perkin Trans 2:2185–2191
Gawinecki R, Kolehmainen E, Loghmani-Khouzani H, Ośmiałowski B, Lovasz T, Rosa P (2006) Effect of π-electron delocalization on tautomeric equilibria - benzoannulated 2-phenacylpyridines. Eur J Org Chem 2006:2817–2824
Ośmiałowski B, Krygowski TM, Dominikowska J, Palusiak M (2011) The effect of benzoannulation on the transition state and the proton transfer equilibrium in di(2-pyridyl)methane derivatives. New J Chem. doi:10.1039/C1NJ20108E
Gawinecki R, Kuczek A, Kolehmainen E, Ośmiałowski B, Krygowski TM, Kauppinen R (2007) Influence of bond fixation in benzo-annulated n-salicylideneanilines and their ortho-C(O)X derivatives (x = CH3, NH2, OCH3) on tautomeric equilibria in solution. J Org Chem 72:5598–5607. doi:10.1021/jo070454f
Krygowski TM, Zachara JE, Ośmiałowski B, Gawinecki R (2006) Topology-driven physicochemical properties of π-electron systems. 1. Does the clar rule work in cyclic pi-electron systems with the intramolecular hydrogen or lithium bond? J Org Chem 71:7678–7682
Ośmiałowski B, Kolehmainen E, Nissinen M, Krygowski TM, Gawinecki R (2002) (1Z,3Z)-1,4-di(pyridin-2-yl)buta-1,3-diene-2,3-diol: The planar highly conjugated symmetrical enediol with multiple intramolecular hydrogen bonds. J Org Chem 67:3339–3345
Ośmiałowski B, Kolehmainen E, Gawinecki R (2003) Identity double-proton transfer in (3Z)-3-hydroxy-1,4-di(quinolin-2-yl)-but-3-en-2-one. Chem Eur J 9:2710–2716
Koch U, Popelier PLA (1995) Characterization of c-h-o hydrogen bonds on the basis of the charge density. J Phys Chem 99:9747–9754. doi:10.1021/j100024a016
Sugiyama T, Meng J, Matsuura T (2002) Intermolecular interactions in the formation of two-component molecular crystals composed of chloronitrobenzoic acids and 4-benzoylpyridine. J Mol Struct 611:53–64
Majerz I, Olovsson I (2010) Influence of proton transfer on the geometry of the donor and acceptor in NHN+ hydrogen bonds. J Mol Struct 976:11–18
Marx D (2006) Proton transfer 200 years after von Grotthuss: Insights from ab initio simulations. Chem Phys Chem 7:1848–1870. doi:10.1002/cphc.200600128
Leffler JE (1953) Parameters for the description of transition states. Science 117:340–341
Acknowledgments
Financial support from the Polish Ministry of Science and Higher Education (grant no. N N204 174138) is gratefully acknowledged. The authors are very much indebted to the ICM in Warsaw for providing computer time and programs.
Author information
Authors and Affiliations
Corresponding author
Supplementary material available
Below is the link to the electronic supplementary material.
ESM 1
(DOC 2210 kb)
Rights and permissions
About this article
Cite this article
Ośmiałowski, B. Proton transfer reaction and intermolecular interactions in associates of 2,5-dihydroxy-1,8-naphthyridine. J Mol Model 18, 1633–1644 (2012). https://doi.org/10.1007/s00894-011-1178-1
Received:
Accepted:
Published:
Issue Date:
DOI: https://doi.org/10.1007/s00894-011-1178-1