Abstract
The former Callahan Mine Site in Brooksville, ME, is an open-pit, hardrock mine site in an intertidal system, thus providing a unique opportunity to evaluate how metal-enriched sediments and overlying water impact estuarine food webs. Copper, zinc, cadmium, and lead concentrations in sediment, whole water, and Atlantic killifish (Fundulus heteroclitus) were evaluated at sites in Goose Pond (GP; Callahan Mine Site) and at reference sites. The metal concentrations of sediment, water, and fish were spatially distinct and significantly greater at the mine site than in the reference estuary. Sediment concentrations were particularly elevated and were above probable effects levels for all four metals adjacent to the tailings pile. Even in this well-mixed system, water metal concentrations were significantly elevated adjacent to the tailings pile, and concentrations of Cu and Zn were above ambient water-quality criteria for chronic marine exposure. Neither organic matter in the sediment nor salinity or pH of the water explained the metal concentrations. Adjacent to the tailings pile, killifish metal body burdens were elevated and were significantly related to both sediment and aqueous concentrations. In conclusion, (1) the contaminated sediment and seepage from the tailings impoundment and waste rock pile no. 3 create a continual flux of metals into the water column, (2) the metals are bioavailable and bioconcentrating as evident in the killifish tissue concentrations, and (3) Callahan Mine is directly affecting metal bioaccumulation in fauna residing in the GP estuary and, potentially, in Penobscot Bay by the way of “trophic nekton relay.”
Similar content being viewed by others
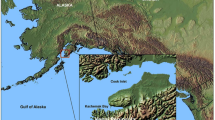
Mining activity and abandoned mine sites are a major source of metal contamination globally and in the United States. In 2008, the United States Government Accountability Office determined that there were at least 161,000 abandoned hardrock mine sites in the 12 western states as well as in Alaska, where most United States mining takes place. At least 33,000 of these sites have degraded the environment by contaminating surface water and groundwater (Mittal 2011). Waste rock and tailings from the operations are often stored in large piles, which can result in persistent acid and toxic metal drainage or dust contamination to the surrounding ecosystem (Schaider et al. 2007). The negative impacts of wet and dry deposition from mine waste piles on local human communities, as well as aquatic and terrestrial wildlife, are numerous and well-documented (Beyer et al. 2004; Hu et al. 2007; Kiser et al. 2010; Malcoe et al. 2002).
The United States Environmental Protection Agency (USEPA) currently has 120 mine sites listed on the National Priorities List or being remediated using the Superfund Alternative Approach (United States Environmental Protection Agency 2012). This includes the Tar Creek site in Oklahoma, the Clark Fork River site and associated Milltown Reservoir in Montana, Eagle Mine in Colorado, and Bunker Hill in Idaho. All of these are contaminant sources to freshwater systems. Studies have been performed on the effects of highly urbanized areas (Bryan and Langston 1992; Hall and Pulliam 1995; McKinley et al. 2011), landfills (Pope et al. 2011), and upstream mining (Rainbow et al. 2011) on estuarine environments. However, there is a paucity of research on sources of metals to estuaries or marine systems, and to our knowledge there are no studies on the effects of an open pit mine in an estuarine environment.
The state of Maine has a number of former hard-rock mines; the most well known and the second largest operation is the former Callahan Mine. The mine was in operation initially from 1881 until 1887. It was worked more intensely again from 1968 to 1972, during which 800,000 tons of copper (Cu) and zinc (Zn) ore were extracted. The remedial investigation for the Callahan Mine Site was conducted from 2004 through 2008 and found elevated levels of Cu, Zn, cadmium (Cd), arsenic (As), and lead (Pb) in surface soils throughout the site. Polychlorinated biphenyl (PCB) contamination was found in the area of the site where the ore-processing facilities were located as well as in surface soils. The specific source of the PCB contamination is not known; however, some of the PCB contamination is believed to be related to former transformers located near the south portion of the Primary and Secondary Crusher buildings (MACTEC 2009c). The concentrations of As, Pb, and PCBs were greater than levels considered acceptable for residential or recreational use. Seepage from the waste rock piles and tailing impoundment to GP was found, in cases, to exceed chronic ambient water-quality criteria (AWQC) concentrations for Cu, Zn, Cd, and Pb, anywhere from just above the AWQC to 2,123 times greater in the case of Zn and 3,581 times greater in the case of Cu for concentrations recorded in 2006 from a seep adjacent to waste rock pile no. 3 (sample SP-509) (MACTEC 2009b). Cu, Zn, Cd, and Pb were are also found to be elevated in sediments and biota tissue, particularly in hot-spot locations in GP and the salt marsh adjacent to the waste rock piles and the tailing impoundment where Cu and Zn were detected at 110 and 150 times greater than the effects-range medium (ERM) benchmark developed by the National Oceanic and Atmospheric Administration (NOAA) at location GP-24 in the salt marsh sediment (United States Environmental Protection Agency 2009; MACTEC 2009a). In light of these contamination sources, we analyzed the spatial gradient of Cu, Zn, Cd, and Pb metal concentrations in the estuary sediment and whole water and the relationship to bioaccumulation in resident forage fish, Atlantic killifish or mummichog (Fundulus heteroclitus), based on water-chemistry readings, known killifish life history, and a consideration of landscape factors.
In aquatic ecosystems, all four metals—Cu, Zn, Cd, and Pb—have the potential to bioaccumulate in local fauna with exposure to sources in sediments or water (Bryan and Langston 1992; Mason 2002) and transfer trophically. Benthic infauna living in contaminated sediments accumulate metals and can transfer contaminants to both pelagic and benthic organisms. Cu, Zn, Cd, and Pb are most bioavailable in their free ion form (Mason 2002). However, this form also most readily complexes, especially so because salinity in the water and/or organic content in sediment increases, thus making the metals less bioavailable (Fernandes and Henriques 1991; Zamuda and Sunda 1982). Simultaneously, enriched sediments are potentially a source of metals to the overlying water, creating another exposure route for pelagic species either in a dissolved, colloidal, or particulate form. Zn, with high assimilation efficiency, has been found to biomagnify in certain cases and affect higher trophic level species (Chen et al. 2000; Mathews and Fisher 2008; Reinfelder et al. 1998). Cu and Cd can either biomagnify or biodiminish depending on the position in the trophic chain (Chen et al. 2000; Rossi and Jamet 2008; Zhang and Wang 2007). Pb, in contrast, has been found to consistently biodiminish in the food web (Chen et al. 2000; Chen and Folt 2000; Mathews and Fisher 2008; Stewart et al. 2005).
Exposure of fish to high levels of either essential (e.g. Cu and Zn) or nonessential metals (e.g. Cd and Pb) retards mental and physical development and fitness, especially in early life stages (Kearns and Atchison 1979; Lefcort et al. 1998; Maes et al. 2013; Vieira et al. 2009). Furthermore, it has been found to decrease wound healing and fin regeneration of fish (Weis and Weis 1976), alter population numbers (Poulton et al. 2010), change an ecosystem’s community composition (Clements and Kiffney 1994; McKinley et al. 2011; Pascoe et al. 1994; van der Merwe et al. 2011), shift feeding patterns (Clements and Rees 1997), and decrease production of specific gene products in response to cellular overstimulation (Maes et al. 2013). In some cases, individuals can develop physiological tolerance, or populations can develop genetic resistance to the metal toxicant, and they can survive with sustainable numbers (Bryan and Langston 1992; Weis and Weis 1989). In particular, killifish populations are known to have a high degree of genetic variability and thus an enhanced ability to buffer stresses and accommodate to a wide-ranging pollution gradient (Whitehead et al. 2011a). Although killifish are known to be more tolerant of environmental and pollution gradients than other species, they still experience stress as a result of exposure and, by surviving, serve as vectors of contaminant transport to other fauna.
In this study, metal concentrations in GP, the estuary impacted by the former Callahan Mine in Brooksville, Maine, were evaluated. The site is notable for being one of the few, if not the only, documented open pit hard-rock mining site in an intertidal zone and provides an opportunity to research a metal contamination source in an estuarine environment. As mentioned previously, risk assessment evaluations conducted by the USEPA, MACTEC, Inc., and other researchers indicate that metal concentrations in the mine site are increased greater than background levels (Dwyer 1973; United States Environmental Protection Agency 2009; MACTEC 2009b; Rajakaruna et al. 2011). To better understand the fate of metals in the estuarine food web, specifically bioadvection, we evaluated the spatial distribution of Cu, Zn, Cd, and Pb within the mine site in sediments and water and the corresponding transfer of metals to fish as affected by site conditions (e.g. organic carbon, pH, salinity, flushing) and biological factors (e.g. life-history factors and predictions through time). Killifish samples were collected, and corresponding sediment and whole water samples were analyzed. Killifish were an ideal study species because their tissue residues represent bioavailable and thus biologically relevant metal concentrations. Killifish are ubiquitous across our study sites as well as being found along the Atlantic coast of the United States into the Gulf of Mexico. They maintain small home ranges, link lower trophic guilds with higher ones, and are extremely tolerant to a range of salinities as well as a range of pollutants (Kneib and Stiven 1978; Kneib et al. 2002; McMahon et al. 2005; Valiela et al. 1977; Whitehead et al. 2011a, b). Metals in whole water samples were analyzed as an indicator of total fish exposure because Cu, Zn, Cd, and Pb can be taken up by absorption over the gills or by way of ingestion (Schlekat et al. 2002; Xu and Wang 2002; Mathews and Fisher 2009).
The main objectives of this study were twofold: for the four metals in question, in a tidally dynamic system (1) to determine if spatial differences in sediment concentrations within the study site were reflected in the overlying surface water concentrations; and (2) to evaluate the relationship of sediment and/or aqueous metal concentrations on body burdens of killifish. The discussion will consider whether the mine is a source of exposure to the local estuarine food web.
Methods
Study Site
The GP estuary is a tidally flushed ecosystem located in Brooksville, Maine (Fig. 1). The estuary has freshwater inflow from Marsh Creek in the south and empties on the north side through a reversing falls into Goose Cove as well as the convergence of Bagaduce River and Penobscot Bay. The site is primarily surrounded by private residential properties, excluding the Holbrook Island Sanctuary State Park on the east border. The mine was owned and operated by the Callahan Mining Corporation between 1964 and 1972 and was located in the lower, more saline portion of the estuary (Metcalf and Eddy 2003). During the mining operation, GP was dammed both at the south end to block Marsh Creek stream water as well as at the north end at the estuary mouth. Following the completion of the dams, GP was drained to allow for the development of an open pit–mining operation. The ore deposit was a Kuroko-style volcanogenic massive sulfide body that consisted of lenses and pods of Zn, Cu, Pb, and Fe sulfide minerals embedded in a talc- and calcite-mineralized zone (MACTEC 2009b). Approximately 5 × 106 tons of rock was removed from the open pit resulting in a mine pit 125–300 m in diameter and >90 m in depth (Woods Hole Group 2007). When the mining operation ceased, an 8-foot section of the Goose Falls dam was opened allowing the system to flood and again be subject to tidal patterns (MACTEC 2009a). The legacy of the mining operations consists of three waste rock piles, a tailings impoundment, ore pad (location where excavated ore material was stored before processing), and the former mine operations area. Portions of GP were used as a settling pond resulting in the accumulation of tailing in the sediment. Dyer Cove and Goose Cove were also used to settle the sediment resulting from dewatering of the open pit (MACTEC 2009b). Analysis of acid-producing and -neutralizing minerals suggests that, within the waste rock piles, the ore pad area, and tailings pile, the minerals are net neutralizing. The net-neutralizing condition buffers the pH of the leachate, whereas sufficient acid rock drainage generation occurs to create leachate with high concentrations of metals in both the total and dissolved fractions (MACTEC 2009b). In 2009, the USEPA selected a remediation plan to alleviate both human and ecologically based impacts. Cleanup of four residential properties with As and Pb contamination was completed in 2011. Cleanup of the PCB contamination in the former mine operations area began in 2011 and is expected to be completed in September 2013. We performed this study to establish an understanding of metal fate in the estuary before any remediation of the estuary.
Sampling sites in Goose Pond (the Callahan Mine site) and our reference site, Horseshoe Cove. HC BRK Horseshoe Cove Brackish, HC EST Horseshoe Cove Estuarine. Please refer to Fig. 2 for insert details of the Callahan Mine Site
Site Selection
We selected six sites in the Goose Pond (GP) estuary of the Callahan Mine Site in Brooksville, Maine, and two control sites in the nearby Horseshoe Cove (HC) estuary (Fig. 1). Within GP (Fig. 2), we collected samples at the following points: (1) at Goose Cove just outside the reversing falls at the mouth of the estuary (GP FALLS), (2) on the north shore of GP just before the mouth (GP MOUTH), (3) along the north shore of Dyer Point (GP DYER), (4) on the western shore of the estuary along side waste rock pile 3 (GP EST), (5) on the western shore south of the former earthen dam and adjacent to the tailings impoundment (GP ADJ), and (6) in the brackish marsh creek area upstream of the mining activity (GP BRK). Reference samples were collected in the nearby HC estuary. HC is on the same peninsula, however, is a slightly larger estuary and opens up to the Penobscot Bay at the opposite end of the peninsula (Fig. 2). In HC, reference samples were collected in two locations to pair with the GP estuarine and brackish site as follows: (1) within the salt marsh area (HC BRK) and (2) further downstream in a more estuarine area (HC EST).
Sample Collection and Analysis
Samples were collected in August 2011 using nonmetallic sampling gear and using trace-metal clean techniques. Water-sampling techniques are derived from USEPA Method 1669, i.e. Sampling Ambient Water for Determinations of Metals at EPA Water Quality Criteria Levels. The biota and sediment sampling techniques are described in Chen et al. (2000) and in Chen and Folt (2000). All containers, bags, and sampling materials were sequentially washed with Citronox detergent (Alconox, Inc., White Plains, NY, USA), trace metal–grade HCL, and trace metal–grade HNO3, and rinsed with DDI water before use. In the field, all samples were immediately frozen on dry ice and later stored at −20 °C until analysis.
Sediment samples were collected during low tide using a 6 cm–diameter coring tube with the top 2 cm of three cores composited into a single sample. This was repeated three times at each site for triplicate samples. Each sample was freeze-dried, homogenized, and an aliquot was analyzed for total metals. Another aliquot of approximately 10 g was muffled at 550 °C for 8 h and allowed to cool overnight. The amount of carbon lost, as a weight, is presented as percent lost on ignition (%LOI).
Triplicate aqueous metal samples were taken at each site as whole water samples (not filtered) and were collected at high tide off of a plastic boat using trace metal–clean techniques. Whole water, rather than filtered, samples were taken because they reflect a range of exposures of metals to biota, including both dissolved as well as particulate metals. They were collected and stored in acid-cleaned Teflon containers. Each sample was acidified in the field to pH 1 with optima nitric acid (0.4 % acid volume) and was refrigerated until analyzed. Concurrent in situ measurements of pH, water temperature, and salinity were taken using a Hydrolab Minisonde 4A (Austin, TX, USA) portable system, which was calibrated daily.
We collected fish at mid or high tides using fish seines and minnow traps and stored these fish using trace metal–clean techniques. Fish total lengths (TLs) and wet weights were measured, and triplicates of fish (TL approximately 60 mm) were selected (0.4–0.8 g dry weight [dw]). Whole fish were freeze-dried and digested with 4 mL of Optima HNO3 and 4 mL of Epure ultrapurified water in a MARSxpress microwave digestion unit (CEM, Mathews, NC, USA) at 180 °C.
Trace-Metal Analysis
All metal samples were analyzed by the Dartmouth Trace Element Core facility by inductively coupled plasma-mass spectrometry (ICP-MS; Agilent 7500cx) using both He mode (Cu, Zn, Cd) and normal mode (Pb). Quality control for all metal results included blank, duplicate, metal spike detection, and certified reference material (SRM) analyses. Sediment had duplicate relative percent differences of 8.7, 2.8, 6.0, and 6.2 % and detection limits (DLs) of 0.18, 0.18, 0.005, and 0.12 ppm for Cu, Zn, Cd, and Pb, respectively, with an SRM recovery of 106 %. For water sample analyses, the SRM NIST-1640a percent recoveries were 100, 102, 98, and 92 % and DLs were 0.23, 1.17, 0.083, and 0.12 ppb for Cu, Zn, Cd, and Pb, respectively. For killifish analysis, the standard reference material DORM-2 was used, which is produced from dogfish muscle tissue and certified by the National Research Council of Canada. In the killifish run, DORM-2 had a recovery of 133, 107, 119, and 130 % of the published metal concentrations for Cu, Zn, Cd, and Pb, respectively, and method DLs of 0.20, 0.40, 0.04, and 0.04 ppm for Cu, Zn, Cd, and Pb. Two of the vessels were run as blanks and subsequently deemed contaminated, and the SRM percent recoveries were re-evaluated at 119, 107, 117, and 118 % for Cu, Zn, Cd, and Pb, respectively.
Data Analyses
Statistical analyses of the data—general linear models—were performed using JMP 5.01a (SAS Institute, Inc., Cary, North Carolina, USA). To evaluate the metal concentrations across the sites for sediment, water, and killifish, we applied one-way analyses of variance (ANOVA) models on untransformed data. All necessary assumptions of the ANOVA were met except equal variance; according to Levene’s test for equality of variances, significant p-values indicated that variances were unequal across sites. However, applying Box’s 1954 publication on theorems on quadratic forms, moderate inequality of variance should not affect the robustness of the ANOVA test when equal sample sizes are present across the sites. With the study design of triplicates for each criterion at each site, which is a balanced design, the robustness of the ANOVA test is maintained. As a check, nonparametric Welch’s method was applied to the data, and the results were compared.
All ANOVA tests were significant, so post hoc Tukey–Kramer methods were then applied with α = 0.05. Regression analyses were used to evaluate the effect of organic content in sediment and salinity and pH readings in the water on the resulting metal concentration. Regression analyses were also applied with sediment and water as predictors of water and killifish metal concentrations.
The NOAA Screening Quick Reference Tables (SQuiRTs) and published metal concentrations present in other sites were used as comparisons to the levels present in this study. The NOAA SQuiRTs provide a series of screening values of suggested toxicological benchmarks of inorganics and organics in sediments, soils, and waters of coastal hazardous waste sites (Buchman 2008). From these tables, probable effects level (PEL), ERM, and chronic AWQC were applied. PEL is a threshold effect concentration referring to the chemical level above which adverse effects are expected to occur frequently. ERM represents the concentration above which adverse effects would frequently be observed. Chronic AWQC is the USEPA AWQC as experienced as a long-term exposure of a contaminant. It is considered the preferred surface water and groundwater benchmark.
For each site and for each metal, a bioaccumulation factor (BAF) was calculated by dividing the mean tissue metal concentration by that of the mean water concentration and then log10-transforming the results. Where water or tissue concentration values were below the analysis DL (BDL) of the mass spectrometer, Dartmouth Trace Element Analysis core did not provide the lower estimate because they are not accurate. Thus, when values were BDL, the DL was applied to the calculation (0.236, 1.776, 0.083, and 0.118 ppb for aqueous Cu, Zn, Cd, and Pb, respectively, and 40 ppb for Cd tissue concentrations). Applying the aqueous DL, which is a high estimate, to the BAF calculation results in a decreased calculation of BAF. This should not matter because uptake rates should be similar at high or low metal concentrations under the assumption that there are no toxicological effects at high metal concentrations.
Ratios of the metal concentrations of GP ADJ, as compared with the mean values present at HC ([metalGP ADJ]/[metalHC mean]), were calculated for each metal in each compartment—sediment, water, and killifish. Again, where water concentration values were BDL, this cut-off was applied to the calculation. In this case, applying the DL value in all calculations is a more conservative approach than applying the estimated lower-than-DL values; the former method is preferred as it decreases intersite as well as intrasite differences.
Results
For all four metals, sediment (Fig. 3), water (Fig. 4), and fish (Fig. 5) varied significantly across all sites (Table 1). This remained true when run as a nonparametric test. By Welch’s test, (1) sediment Cu (p < 0.0002), Zn (p < 0.0002), Cd (p < 0.006), and Pb (p < 0.180), (2) water Zn and Cd (both with p < 0.0001), and (3) fish Cu, Zn, Cd, and Pb (all with p < 0.0003) varied significantly across sites. Significance could not be determined for water Cu and Pb because concentrations in HC were lower than the DLs of the analysis. The samples collected adjacent to the tailings pile (GP ADJ) showed concentrations that were significantly greater than that of the HC reference sites. The mean and SD for each bar shown in Figs. 4 and 5 are provided as supplementary materials available online. There was 92× more Cu, 107× more Zn, 98× more Cd, and 34× more Pb recorded in the sediment from GP ADJ than in HC. For all four metals, concentrations at GP ADJ and GP EST exceeded those denoted as PELs (Fig. 3) and for all metals; excepting Pb, they also exceeded the ERM value (Buchman 2008). Water samples collected at GP ADJ had Cu and Zn concentrations that were greater than chronic AWQC for unfiltered water samples (Fig. 4). GP EST and GP BRK water Cu concentrations also were greater than chronic AWQC (Buchman 2008). Similar to sediment concentrations, overlying water showed increased concentrations adjacent to the tailings pile and, in the case of Cu and Cd, just upstream also. Killifish tissue concentrations were elevated adjacent to the tailings pile as well as just upstream and just downstream (Fig. 5). Sediment and water had greater across-site than within-site variability (larger F-statistic) than did killifish samples, and the ANOVA model had greater goodness of fit (R 2) for sediment and water samples compared with that for killifish (Table 1). At the outlet of the mine site (GP MOUTH and GP FALLS), all three compartments—sediment, water, and fish tissue—showed levels that were not significantly different from those in the reference site.
Whole water metal concentrations (triplicate mean ± SD) of a Cu, b Zn, c Cd, and d Pb across six sites in GP and two sites in HC. The dashed line indicates chronic AWQC for unfiltered marine waters with the Cd and Pb criteria greater than the concentrations shown by our data. Lower-case letters indicate significantly similar multiple comparisons as determined by the Tukey–Kramer method. Bars with “bdl” indicate that concentrations were lower than the DLs of the instrument: 0.24, 1.78, 0.08, and 0.12 ppb for Cu, Zn, Cd, and Pb, respectively
Killifish (0.4–0.8 g of dw) whole-body metal concentrations (triplicate mean ± SD) of a Cu, b Zn, c Cd, and d Pb across six sites in GP and two sites in HC. Lower-case letters indicate significantly similar multiple comparisons as determined by the Tukey–Kramer method. Bars with “bdl” indicate that concentrations were lower than the DLs of the instrument: 0.04 for Cd
As possible biogeochemical factors affecting the bioavailability of the metals, we considered sediment organic matter and salinity and pH in the water. Within the GP sites, percent organic matter, as measured by %LOI, varied from just <4 % to almost 17 % (Table 2), and %LOI was not related to the sediment metal concentration or overlying water concentrations (Table 3). Salinity and pH both varied very slightly across the sites (Table 2); however, they did not predict metal concentration in the water or in the fish (Table 3). There was no evidence of salinity stratification.
The metals differed in their relationships across sites and between biotic and abiotic compartments. Across all metals, concentrations in sediments were strongly correlated to those in water and biota at the same site (Table 3). By regression analyses, the sediment-to-biota relationship was strongest in Cd and Pb. Water metal concentrations as a predictor of biota concentrations were strongest for Pb and Zn. BAFs based on water concentrations indicate that bioavailability of metals from water were greatest for Cu and Zn (Table 4). At GP ADJ, sediment concentrations of Cu, Zn, and Cd were the most increased above those recorded at local reference sites, whereas aqueous concentrations of Cu and Zn were the most elevated above those in the reference site. Cu and Zn were also the least increased in killifish tissues (Table 5).
Discussion
Sediment-Bound Metals
The results of the present study shows that GP has sediment metal concentrations increased above those present in the reference site as well as Gulf of Maine background levels; this is supported by past findings (Dwyer 1973; United States Environmental Protection Agency 2009; MACTEC 2009a, b; LeBlanc et al. 2011). Sediment concentrations within GP were significantly increased adjacent to the tailings pile (GP ADJ) where they were greater than PEL and ERM thresholds (Buchman 2008) suggesting that the concentrations present in these sediments are harmful to aquatic organisms. Colvin et al. (2011, unpublished data) found that polycheates (Neanthes arenaceodentata) experienced a complete die-off when exposed to GP ADJ and GP EST sediments in a 28-day toxicity test. In a subsequent study with a 96-h sediment–water interface exposure to sea urchin embryos (Strongylocentrotus purpuratus), all embryos showed abnormal development. In the study, only one of three sediment samples had a positive calculation of acid-volatile sulfides subtracted from simultaneously extracted metals (ΣSEM-AVS), suggesting nontoxic conditions. AVS is a measure of sulfide that has the potential to react with free metals. SEM is a measure of reactive metals as measured by the cold acid-extraction method. For divalent metals (e.g. Cu, Cd, Pb, Zn, and nickel [Ni]), the AVS present in the sediment binds with the SEM present to form metal sulfides and render the metals unavailable to biota (McGrath et al. 2002). Positive values of ΣSEM-AVS in sediment have been related to toxicity in benthic toxicity tests suggesting that the metals are bioavailable (Berry et al. 1996). According to Colvin et al. (2011, unpublished data), even with negative values, however, sediments in GP proved to be toxic. In contrast to what would be expected, ΣSEM-AVS was not found to be an accurate predictor for toxicity in these sediments.
The substrate underlying GP ADJ, as well as GP EST, is composed of mine waste, which might explain the high sediment metal concentrations in these areas. GP EST is located in an area that historically was used as an effluent-settling pond for the processing of water from the tailings impoundment. As a result, the benthic substrate in this area is primarily comprised of tailings. Similarly, the benthic substrate of GP ADJ is also comprised of tailings due to erosion and overflow directly from the tailings impoundment. Furthermore, above-ground seeps flow off of the waste rock piles and the tailings pile into the estuary, which may be contributing to the metal enrichment found in both the water and the sediments. The most visible and highest concentration seeps (Cu: 0.02–10 mg/L; Zn: 2–10 mg/L; Cd: 0.007–0.5 mg/L; and Pb: 0.002–0.2 mg/L) flow from the bank of GP between GP ADJ and GP EST (MACTEC 2009b). Precipitates from the seeps are observable with red-orange phases indicating iron hydroxides and white to blue precipitants consisting of calcium sulfate and aluminum and Cu sulfates (MACTEC 2009b).
Although lower than that of GP ADJ, GP BRK also contains levels of metals in the sediment that are enriched significantly greater than the levels present in our reference site, HC. When Callahan Mine was in operation, there was an earthen dam between GP ADJ and GP EST. Historically, Marsh Creek (the freshwater stream) pooled at this dam and was forced to drain south and thus away from the mine site. At the time of operation, GP BRK was likely not heavily exposed to mine waste or tailings. The sediment enrichment evident at GP BRK is likely, then, the result of the long-term deposition of particulates transported by tidal inflow after the release of the earthen dam.
The metals in GP are enriched on a fine spatial scale. GP ADJ had sediment concentrations that were significantly different from those found at GP BRK or at GP EST. In all metals except Pb, GP EST was significantly different than GP DYER. These sites are in close proximity to one another with only 300–500 m of separation. Sediment concentrations just north of GP EST, starting at GP DYER and including all other sites toward the outlet of GP, had metal levels not significantly different from background levels. Ayuso et al. (2013) found that beyond GP FALLS in Goose Cove, the sediment concentrations quickly diminished to background levels; however, there was a spike in sediment metal concentration just beyond GP FALLS. This is likely from historical use when there was a sump pump releasing effluent from the mine pit into this area, which is consistent with our observations of the direct presence of mine waste having the greatest observable impact on metal levels.
The sediment data from the present study suggest that historic deposition of tailing in the bottom substrate of GP ADJ and GP EST, as well as erosion from the tailings pile and waste rock pile no. 3, are the most pronounced sources of enrichment to the sediment. There are likely additional contributions from terrestrial seeps coming off of the waste rock piles. By applying Pb isotopes as means of identifying the source of metals, Ayuso et al. (2013) came to the same conclusion. The results of the present study found that the metal signal in sediment is relatively low adjacent to the other waste rock piles both in the historic settling pond (Dyers Cove) and alongside the ore pad (location where excavated ore material was stored before processing). The highest groundwater concentrations are found beneath the ore pad (MACTEC 2009b) indicating that it has high source potential as has been found in other study areas (Velleux et al. 2006). Here, however, it appears that the soil is filtering the impact before discharge into GP DYER.
Sediment organic content is known to bind metals, thus influencing their availability and rate of flux to overlying water (Doyle and Otte 1997; Hung and Chmura 2006; Otte et al. 1993). With ranges of 6–20 %LOI, Otte et al. (1993) found significant correlations with sediment metal concentrations. In GP, even with a comparatively large range of %LOI (4–17 %), %LOI did not relate to concentrations in either sediment or water. Organic matter, therefore, may not be significantly controlling the sediment metal gradient. It may help to explain the difference in concentrations between GP EST and GP ADJ. The organic content in GP EST was greater than that of GP ADJ suggesting that, over time, the tailings substrate beneath GP EST is maturing and that the metals present are slowly being sequestered by the deposits of lower-concentration particulates transported either from Marsh Creek or by the tides.
Compared with other mine sites and other anthropogenically affected sites, sediment concentrations of Zn, Cd, and Pb adjacent to the tailings site were high, equivalent to those detected in the waste rock piles of the Tar Creek Superfund Site in Oklahoma (Schaider et al. 2007) and slightly lower than the soil levels of Cu and Ni found in a Cu-smelting area in Ontario, Canada (Freedman and Hutchinson 1980). Compared globally with the sediment concentrations of mining sites, the levels found in GP and Goose Cove are not unusual; however, regionally the site represents a hot spot of Cu, Zn, and Pb for the Gulf of Maine surpassed only by levels in some areas of Boston Harbor (LeBlanc et al. 2011; MACTEC 2009b).
Aqueous Metals
Addressing our first study aim, we found that the spatial differences in sediments are reflected in the overlying water with our sampling site adjacent to the tailings pile experiencing the highest levels of metals. Although there typically is a strong correlation between sediment concentration and that in associated porewater and overlying water (Mason 2002), it is surprising to see such a strong correlation in a tidally influenced estuary where there is a natural mixing of the water column, which could result in more homogenous aqueous metal concentrations. For all sites but HC BRK, there is no indication of salinity stratification. Instead the systems appear to be relatively homogeneous with a well-mixed water column. Tidal cycles measured over a month (May 2007–June 2007) indicate that GP BRK experiences a wide range of salinities (0–26 ppt) with most daily tidal fluctuations ranging from approximately 10 to 24 ppt (MACTEC 2009b). Together these data suggest that the system experiences a relatively large exchange of freshwater to salt water and can be considered well mixed. Furthermore, our sampling stations were in relatively close proximity to one another, i.e. separated by only 300–500 m. Nonetheless, water concentrations in GP parallel those present in the sediment; water was metal-enriched adjacent to the tailings pile and farther upstream in the GP BRK site. We sampled water during high tide when the saltwater backs up into the Marsh Creek brackish area; that is likely why we saw increased metals, particularly Cu and Cd, in water upstream of the tailings pile. Cu and Zn (unlike Cd and Pb) stand out as being present in concentrations that exceed AWQC for whole waters (Buchman 2008).
Studies have found that water chemistry factors can affect aqueous metal concentrations. Metals can complex with carbon sequestering them in sediment (Amiard et al. 2007; Baumann and Fisher 2011). This is particularly true for Cu, which is almost entirely bound to organic matter in marine systems, whereas Cd is less strongly bound to carbon (Mason 2002). In this system, however, we found that %LOI was not predictive of the overlying aqueous concentrations for any of the evaluated metals and, as stated previously, that %LOI also did not predict sediment concentrations. Simultaneously, the salinity and pH of the water did not predict aqueous metal concentrations present.
Metals from sediment appear to be continuously fluxing to the overlying water, and water concentrations may also be influenced by terrestrial seeps draining from between the tailings pile and waste rock pile no. 3 (United States Environmental Protection Agency 2009). Zn concentrations in sediments were most tightly correlated to concentrations in the overlying surface water, followed by Pb and Cu, suggesting that these metals are experiencing the highest rate of fluxing from the sediments to the water and thus are likely the most available for accumulation by phytoplankton, microorganisms, and algae (Pope et al. 2011). MACTEC, Inc., evaluated the distribution coefficients of concentrations in sediment to its corresponding interstitial water (Kd) (MACTEC 2009b). Their data suggest that Cd and Pb have the highest rate of flux from sediment to porewater and, potentially, from porewater to overlying water. However, the investigation also found that porewater concentrations were relatively low suggesting that, overall, the Callahan Mine Site is experiencing low fluxing rates and that seeps are potentially contributing to the water signal. Both sediment fluxing and terrestrial seeps appear to be sources with correlating patterns and strong relationships between sediment and water concentration suggesting sediments as the dominant source of metals to the overlying water.
Abiotic to Biotic Transmission
Completing the second study aim, it was found that the study organism, killifish, had the highest body burdens at the sites with the highest sediment and aqueous concentrations. Bioaccumulation was significantly greater than background levels adjacent to the tailings pile and just downstream adjacent to waste rock pile no. 3. Killifish forage in a relatively constrained area and are likely integrating metal signals from only one site (McMahon et al. 2005); however, we had anticipated some overlap in killifish body burdens given the small distance between sampling sites. Although more variable than the sediment and water compartments, fish tissue data show distinct spatial differences in metal tissue concentrations across our sampling sites. Most nonmethylated metals have a short half-life and are depurated rapidly (Mason 2002); therefore, this strong spatial pattern of bioaccumulation suggests that killifish in certain regions of GP estuary are constantly exposed to either dietary or aqueous metals from the Callahan Mine Site.
Body burdens depend on a number of external factors including, but not limited to, water chemistry affecting the bioavailability of the metal(s) in question, routes of uptake, accumulation and elimination efficiencies, and growth dilution. Salinity and alkalinity negatively correspond with metal aqueous bioavailability (Bryan and Langston 1992; Dutton and Fisher 2011); however, neither salinity nor pH were found to be driving factors behind the concentrations present in our fish samples. To account for age and feeding differences, we used only killifish of the same species and size class. The size class of killifish that we evaluated—60-mm TL—were likely second season matures and would have predated on amphipods, tanaids, polycheates, and small crabs as well as grazed the sediment–water interface for detrital material and benthic algae (Kneib and Stiven 1978). They consume food mainly from the benthic layer where flora and fauna are exposed to increased metal concentrations in sediment.
Together, both sediment-bound as well as aqueous metals are likely to be a source to local fauna, and metals may be available to biota either through waterborne or dietary exposure or both (Folt et al. 2002; McCarty and Mackay 1993; Wang et al. 1999). In the study, metals were not only bioavailable but were also bioconcentrating within fish tissue at many times greater than were present in the water.
Biotic uptake from either water or sediment appeared to be dependent on the metal with Pb and Cd overall showing lower body burden relative to water than Cu and Zn (Table 4). This is likely because Cu and Zn are essential metals and are biologically regulated, whereas Pb and Cd are not (Bryan et al. 1983; Mason 2002; McGeer et al. 2003). This difference may explain why Pb and Cd had the highest ratio of fish tissue metal concentrations from GP ADJ against background levels (Table 5). Pb and Cd BAFs were also greater than Cu and Zn across the sites.
Possible Effects Beyond GP Proper
There is a need to consider metal concentrations in a contaminated system in a context beyond that provided by toxicity test results (Fisher and Hook 2002; Whitehead et al. 2011a). Metals can be physically transported or bioadvected from their source. The baseline ecological risk assessment for the Callahan Mine Site stated that the site poses a risk of harm to benthic and pelagic communities, insectivorous birds (e.g. spotted sandpiper), and piscivorous birds (e.g. great blue heron) but only within the sediment hot spots (MACTEC 2009a). However, through the physical transport of the metals by the tide and freshwater input as well as ecological transport mechanisms of trophic nekton relay, both nutrients and contaminants from the mine site have the potential to transfer from waste rock seeps and sediment deposits to coastal food webs. Metals may biotransfer as predatory fish and piscivorous birds that use the estuary as feeding grounds or as a nursery carry the contaminants out of the system in their gut and tissues. Thus, even with low metal concentrations in sediment, water, and fish at the estuary outlet (GP MOUTH and GP FALLS), the transport of the metals from within the site may nonetheless have effects in coastal marine food webs with GP killifish as a possible vector for ecological exposure of transient species. Ayuso et al. (2013) found that Pb concentrations of lobsters and crabs were elevated beyond GP MOUTH in the channel between Holbrook Island and Cape Rosier. This may be due to the historical pollution in this area by the effluent pipe in Goose Cove or may be indicative of metals bioadvection.
Conclusion
Data from abiotic and biotic compartments indicate that there is a significant hot spot of contamination in the GP estuary coinciding with the tailings impoundment, waste rock pile no. 3, and/or seeps coming from these two areas. Despite the tidal and dynamic nature of the system, the spatial distribution of metals is apparent on a fine scale with little variability within sites compared with across sites. It is clear that in this ecosystem, sediments and the adjacent tailings pile serve as potential sources of contaminants to the estuarine food web.
Our findings suggest that metals, continually fluxing to the overlying water from the sediments and/or seeps, are bioavailable and may be transferred through the food web resulting in metal bioadvection beyond the local scale of the GP estuary. In another former mine site, Rainbow et al. (2011) found that even after 30 years, there was no change in sediment and biota metal concentrations. With this in mind, it is unlikely that the contamination and the resulting bioaccumulation will naturally attenuate given the large source of metal in contaminated sediments, tailings, and rock piles.
References
Amiard JC, Geffard A, Amiard-Triquet C, Crouzet C (2007) Relationship between the lability of sediment-bound metals (Cd, Cu, Zn) and their bioaccumulation in benthic invertebrates. Estuar Coast Shelf Sci 72(3):511–521
Ayuso RA, Foley NK, Seal RR II, Bove M, Civitillo D, Cosenza A et al (2013) Lead isotope evidence for metal dispersal at the Callahan Cu–Zn–Pb mine: Goose Pond tidal estuary, Maine, USA. J Geochem Explor 126–127:1–22
Baumann Z, Fisher NS (2011) Relating the sediment phase speciation of arsenic, cadmium, and chromium with their bioavailability for the deposit-feeding polychaete Nereis succinea. Environ Toxicol Chem 30(3):747–756
Berry WJ, Hansen DJ, Mahony JD, Robson DL, DiToro DM, Shipley BP et al (1996) Predicting the toxicity of metal-spiked laboratory sediments using acid-volatile sulfide and interstitial water normalizations. Environ Toxicol Chem 15(12):2067–2079
Beyer WN, Dalgarn J, Dudding S, French JB, Mateo R, Miesner J et al (2004) Zinc and lead poisoning in wild birds in the Tri-State Mining District (Oklahoma, Kansas, and Missouri). Arch Environ Contam Toxicol 48(1):108–117
Box GEP (1954) Some theorems on quadratic forms applied in the study of analysis of variance problems.1. Effect of inequality of variance in the one-way classification. Ann Math Stat 25(2):290–302
Bryan GW, Langston WJ (1992) Bioavailability, accumulation and effects of heavy-metals in sediments with special reference to United Kingdom estuaries—A review. Environ Pollut 76(2):89–131
Bryan GW, Langston WJ, Hummerstone LG, Burt GR, Ho YB (1983) An assessment of the gastropod, Littorina littorea, as an indicator of heavy-metal contamination in United Kingdom estuaries. J Mar Biol Assoc U K 63(2):327–345
Buchman MF (2008) NOAA screening quick reference tables, vol 34. Office of Response and Restoration Division, National Oceanic and Atmospheric Administration, Seattle
Chen CY, Folt CL (2000) Bioaccumulation and diminution of arsenic and lead in a freshwater food web. Environ Sci Technol 34(18):3878–3884
Chen CY, Stemberger RS, Klaue B, Blum JD, Pickhardt PC, Folt CL (2000) Accumulation of heavy metals in food web components across a gradient of lakes. Limnol Oceanogr 45(7):1525–1536
Clements WH, Kiffney PM (1994) Integrated laboratory and field approach for assessing impacts of heavy metals at the Arkansas River. Colorado. Environ Toxicol Chem 13(3):397–404
Clements WH, Rees DE (1997) Effects of heavy metals on prey abundance, feeding habits, and metal uptake of brown trout in the Arkansas River, Colorado. Trans Am Fish Soc 126(5):774–785
Colvin MA, Rosen G, Rivera-Duarte I, Earley P, Swope B (2011) Evaluation of tools towards improved assessment of copper bioavailability and toxicity at contaminated sediment sites. Presented as a poster at the 32nd Annual Meeting of the Society of Environmental Toxicology and Chemistry, Boston, MA, Nov 13–17, 2011 [abstract available at boston.setac.org]
Doyle MO, Otte ML (1997) Organism-induced accumulation of iron, zinc and arsenic in wetland soils. Environ Pollut 96(1):1–11
Dutton J, Fisher NS (2011) Salinity effects on the bioavailability of aqueous metals for the estuarine killifish Fundulus heteroclitus. Environ Toxicol Chem 30(9):2107–2114
Dwyer RL (1973) The relation between heavy metal effluents and benthic infaunal community changes in a coastal embayment in Maine. Massachusetts Institute of Technology, Boston
Fernandes JC, Henriques FS (1991) Biochemical, physiological, and structural effects of excess copper in plants. Bot Rev 57(3):246–273
Fisher NS, Hook SE (2002) Toxicology tests with aquatic animals need to consider the trophic transfer of metals. Toxicology 181:531–536
Folt CL, Pickhardt PC, Chen CY (2002) Using plankton food web variables as indicators for the accumulation of toxic metals in fish. In: Wilson SH, Suk WA (eds) Biomarkers of environmental associated disease: technologies, concepts, and perspectives. CRC Press, Boca Raton, pp 287–304
Freedman B, Hutchinson TC (1980) Effects of smelter pollutants on forest leaf litter decomposition near a nickel-copper smelter at Sudbury, Ontario. Can J Bot 8(15):1722–1736
Hall WS, Pulliam GW (1995) An assessment of metals in an estuarine wetlands ecosystem. Arch Environ Contam Toxicol 29(2):164–173
Hu H, Shine J, Wright RO (2007) The challenge posed to children’s health by mixtures of toxic waste: the Tar Creek superfund site as a case-study. Pediatr Clin North Am 54(1):155–172
Hung GA, Chmura GL (2006) Mercury accumulation in surface sediments of salt marshes of the Bay of Fundy. Environ Pollut 142(3):418–431
Kearns PK, Atchison GJ (1979) Effects of trace metals on growth of yellow perch (Perca flavescens) as measured by RNA–DNA ratios. Environ Biol Fish 4(4):383–387
Kiser T, Hansen J, Kennedy B (2010) Impacts and pathways of mine contaminants to bull trout (Salvelinus confluentus) in an Idaho watershed. Arch Environ Contam Toxicol 59(2):301–311
Kneib RT, Stiven AE (1978) Growth, reproduction, and feeding of Fundulus heteroclitus (L) on a North Carolina salt marsh. J Exp Mar Biol Ecol 31(2):121–140
Kneib RT, Weinstein MP, Kreeger DA (2002) Salt marsh ecoscapes and production transfers by estuarine nekton in the southeastern United States. In: Weinstein MP, Kreeger DA (eds) Concepts and controversities in salt marsh ecology. Kluwer Academic Publishers, The Netherlands, pp 267–291
LeBlanc LA, Krahforst C, Aube J, Roach S, Harding G, Hennigar P, et al. (2011) Gulfwatch 2009 data report: Eighteenth year of the Gulf of Maine Environmental Monitoring Program. Gulf of Maine Environmental Monitoring Program, Gulf of Maine Council of the Marine Environment
Lefcort H, Meguire RA, Wilson LH, Ettinger WF (1998) Heavy metals alter the survival, growth, metamorphosis, and antipredatory behavior of Columbia spotted frog (Rana luteiventris) tadpoles. Arch Environ Contam Toxicol 35(3):447–456
MACTEC (2009a) Final baseline ecological risk assessment, Callahan Mine Superfund Site, Brooksville, Maine. Project no. 3612-06-2047-27. MACTEC Engineering and Consulting, Inc., Portland
MACTEC (2009b) Remedial investigation report. Volumes 1A, 1B, 1C, and 1D. Callahan Superfund Site, Brooksville, Maine. Project no. 3612062047. MACTEC Engineering and Consulting, Inc., Portland
MACTEC (2009c, July) Final operable unit 1 feasibility study, Callahan Mine Superfund Site, Brooksville, Maine. Project no. 3612062047. MACTEC Engineering and Consulting, Inc., Portland
Maes GE, Raeymaekers JAM, Hellemans B, Geeraerts C, Parmentier K, De Temmerman L et al (2013) Gene transcription reflects poor health status of resident European eel chronically exposed to environmental pollutants. Aquat Toxicol 126:242–255
Malcoe LH, Lynch RA, Kegler MC, Skaggs VJ (2002) Lead sources, behaviors, and socioeconomic factors in relation to blood lead of Native American and white children: a community-based assessment of a former mining area. Environ Health Perspect 110:221–231
Mason RP (2002) The Bioaccumulation of mercury, methylmercury, and other toxic elements into pelagic and benthic organisms. In: Newman MC, Roberts MH, Hale RC (eds) Coastal and estuarine risk assessment. Lewis, New York, pp 127–149
Mathews T, Fisher NS (2008) Trophic transfer of seven trace metals in a four-step marine food chain. Mar Ecol Prog Ser 367:23–33
Mathews T, Fisher NS (2009) Dominance of dietary intake of metals in marine elasmobranch and teleost fish. Sci Total Environ 407(18):5156–5161
McCarty LS, Mackay D (1993) Enhancing ecotoxicological modeling and assessment. Environ Sci Technol 27(9):1719–1728
McGeer JC, Brix KV, Skeaff JM, DeForest DK, Brigham SI, Adams WJ et al (2003) Inverse relationship between bioconcentration factor and exposure concentration for metals: implications for hazard assessment of metals in the aquatic environment. Environ Toxicol Chem 22(5):1017–1037
McGrath JA, Paquin PR, Di Toro DM (2002) Use of the SEM and AVS approach in predicting metal toxicity in sediment: A fact sheet on environmental risk assessment. International Council of Mining and Metals. Available at: www.icmm.com. Accessed 22 Feb 2013
McKinley AC, Miskiewicz A, Taylor MD, Johnston EL (2011) Strong links between metal contamination, habitat modification and estuarine larval fish distributions. Environ Pollut 159(6):1499–1509
McMahon KW, Johnson BJ, Ambrose WG (2005) Diet and movement of the killifish, Fundulus heteroclitus, in a Maine salt marsh assessed using gut contents and stable isotope analyses. Estuaries 28(6):966–973
Metcalf and Eddy, Inc. (2003) Conceptual model and RI/FS SOW, Callahan Mining Superfund Site, Brookville, Maine. United States Environmental Protection Agency. Available at: www.epa.gov/ne/superfund/sites/callahan/44290.pdf. Accessed 22 Jan 2013
Mittal AK (2011) Abandoned mines: Information on the Number of hardrock mines, cost of cleanup, and value of financial assurances statement. Natural Resources, Subcommittee on Energy and Mineral Resources. United States Government Accountability Office
Otte ML, Haarsma MS, Broekman RA, Rozema J (1993) Relation between heavy-metal concentrations in salt-marsh plants and soil. Environ Pollut 82(1):13–22
Pascoe GA, Blanchet RJ, Linder G, Palawski D, Brumbaugh WG, Canfield TJ et al (1994) Characterization of ecological risks at the Milltown Reservoir Clark-Fork River Sediments Superfund Site, Montana. Environ Toxicol Chem 13(12):2043–2058
Pope ND, O’Hara SCM, Imamura M, Hutchinson TH, Langston WJ (2011) Influence of a collapsed coastal landfill on metal levels in sediments and biota—A portent for the future? J Environ Monit 13(7):1961–1974
Poulton BC, Allert AL, Besser JM, Schmitt CJ, Brumbaugh WG, Fairchild JF (2010) A macroinvertebrate assessment of Ozark streams located in lead-zinc mining areas of the Viburnum Trend in southeastern Missouri, USA. Environ Monit Assess 163(1–4):619–641
Rainbow PS, Kriefman S, Smith BD, Luoma SN (2011) Have the bioavailabilities of trace metals to a suite of biomonitors changed over three decades in SW England estuaries historically affected by mining? Sci Total Environ 409(8):1589–1602
Rajakaruna N, Harris TB, Clayden SR, Dibble AC, Olday FC (2011) Lichens of the Callahan Mine, a copper- and zinc-enriched Superfund Site in Brooksville, Maine, USA. Rhodora 113(953):1–31
Reinfelder JR, Fisher NS, Luoma SN, Nichols JW, Wang WX (1998) Trace element trophic transfer in aquatic organisms: a critique of the kinetic model approach. Sci Total Environ 219(2–3):117–135
Rossi N, Jamet J-L (2008) In situ heavy metals (copper, lead and cadmium) in different plankton compartments and suspended particulate matter in two coupled Mediterranean coastal ecosystems (Toulon Bay, France). Mar Pollut Bull 56(11):1862–1870
Schaider LA, Senn DB, Brabander DJ, McCarthy KD, Shine JP (2007) Characterization of zinc, lead, and cadmium in mine waste: Implications for transport, exposure, and bioavailability. Environ Sci Technol 41(11):4164–4171
Schlekat CE, Lee B, Luoma SN (2002) The bioaccumulation of mercury, methylmercury, and other toxic elements into pelagic and benthic organisms. In: Newman MC, Roberts MH, Hale RC (eds) Coastal and estuarine risk assessment. Lewis, New York, pp 151–188
Stewart GM, Fowler SW, Teyssie JL, Cotret O, Cochran JK, Fisher NS (2005) Contrasting transfer of polonium-210 and lead-210 across three trophic levels in marine plankton. Mar Ecol Prog Ser 290:27–33
United States Environmental Protection Agency (2009) Callahan Mining Corporation public information meeting for proposed cleanup plan. SDMS# 452697. USEPA. Available at: http://www.epa.gov/region1/superfund/sites/callahan/452697.pdf. Accessed 22 Jan 2013
United States Environmental Protection Agency (2012) Abandoned mine lands: NPL mining sites. Available at: http://www.epa.gov/aml/amlsite/npl.htm. Accessed 21 Jan 2013
Valiela I, Wright JE, Teal JM, Volkmann SB (1977) Growth, production and energy transformations in salt-marsh killifish Fundulus heteroclitus. Mar Biol 40(2):135–144
van der Merwe D, Carpenter JW, Nietfeld JC, Miesner JF (2011) Adverse health effects in Canada geese (Branta canadensis) associated with waste from zinc and lead mines in the tri-state mining district (Kansas, Oklahoma, and Missouri, USA). J Wildl Dis 47(3):650–660
Velleux ML, Julien PY, Rojas-Sanchez R, Clements WH, England JF Jr (2006) Simulation of metals transport and toxicity at a mine-impacted watershed: California Gulch, Colorado. Environ Sci Technol 40(22):6996–7004
Vieira LR, Gravato C, Soares A, Morgado F, Guilhermino L (2009) Acute effects of copper and mercury on the estuarine fish Pomatoschistus microps: linking biomarkers to behavior. Chemosphere 76(10):1416–1427
Wang WX, Stupakoff I, Fisher NS (1999) Bioavailability of dissolved and sediment-bound metals to a marine deposit-feeding polychaete. Mar Ecol Prog Ser 178:281–293
Weis P, Weis JS (1976) Effects of heavy-metals on fin regeneration in killifish, Fundulus heteroclitus. Bull Environ Contam Toxicol 16(2):197–202
Weis JS, Weis P (1989) Tolerance and stress in a polluted environment. Bioscience 39(2):89–95
Whitehead A, Pilcher W, Champlin D, Nacci D (2011a) Common mechanism underlies repeated evolution of extreme pollution tolerance. Proc R Soc B Lond Biol Sci 279(1728):427–433
Whitehead A, Roach JL, Zhang S, Galvez F (2011b) Genomic mechanisms of evolved physiological plasticity in killifish distributed along an environmental salinity gradient. Proc Natl Acad Sci U S A 108(15):6193–6198
Woods Hole Group I (2007) Hydrodynamic observations at the former Callahan Mine property, Brooksville, ME: August 24–October 29, 2006 Woods Hole Group, Inc., East Falmouth, MA
Xu Y, Wang WX (2002) Exposure and potential food chain transfer factor of Cd, Se and Zn in marine fish Lutjanus argentimaculatus. Mar Ecol Prog Ser 238(0171–8630):173–186
Zamuda CD, Sunda WG (1982) Bioavailability of dissolved copper to the American oyster Crassostrea virginica.1. Importance of chemical speciation. Mar Biol 66(1):77–82
Zhang L, Wang W-X (2007) Size-dependence of the potential for metal biomagnification in early life stages of marine fish. Environ Toxicol Chem 26(4):787–794
Acknowledgments
The authors thank Edward Hathaway for time and expertise on this project and Bart Hoskins for comments in the early stages of analyzing the data. Metal concentrations were measured in Brian Jackson’s laboratory at the Dartmouth Trace Element Analysis core by R. Arthur Baker and Vivien Taylor. This publication was made possible by National Institutes of Health (NIH) Grant No. P42 ES007373 from the National Institute of Environmental Health Sciences. Its contents are solely the responsibility of the authors and do not necessarily represent the official views of the NIH or the USEPA. We are grateful to the Center of Environmental Health Sciences at Dartmouth College for the pilot funds, which made this project possible.
Author information
Authors and Affiliations
Corresponding author
Electronic supplementary material
Below is the link to the electronic supplementary material.
Rights and permissions
About this article
Cite this article
Broadley, H.J., Buckman, K.L., Bugge, D.M. et al. Spatial Variability of Metal Bioaccumulation in Estuarine Killifish (Fundulus heteroclitus) at the Callahan Mine Superfund Site, Brooksville, ME. Arch Environ Contam Toxicol 65, 765–778 (2013). https://doi.org/10.1007/s00244-013-9952-y
Received:
Accepted:
Published:
Issue Date:
DOI: https://doi.org/10.1007/s00244-013-9952-y