Abstract
B7-H3 is a member of the B7 superfamily and a putative inhibitory immune checkpoint molecule. Several early-phase clinical trials have reported promising anti-tumor activity and safety of anti-cancer drugs targeting B7-H3, suggesting that it may be a promising target for a potential next-generation immune checkpoint inhibitor. Despite ongoing clinical studies, most B7-H3-targeted drugs being currently investigated rely on direct cytotoxicity as their mechanisms of action rather than modulating its function as an immune checkpoint, at least in part due to its incompletely understood immune regulatory function. Recent studies have begun to elucidate the role of B7-H3 in regulating the tumor microenvironment (TME). Emerging evidence suggests that B7-H3 may regulate the interferon-STAT1 axis in the TME and promote immune suppression. Similarly, increasing evidence shows B7-H3 may be implicated in promoting M1 to M2 polarization of tumor-associated macrophages (TAMs). There is also accumulating evidence suggesting that B7-H3 may play a role in the heterotypic fusion of cancer stem cells and macrophages, thereby promoting tumor invasion and metastasis. Here, we review the recent advances in the understanding of B7-H3 cancer immunobiology with a focus on highlighting its potential role in the interferon priming of TAMs and the heterotypic fusion of TAMs with cancer stem cells and suggest future direction in elucidating its immune checkpoint function.


Similar content being viewed by others
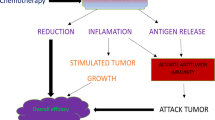
Change history
22 February 2024
A Correction to this paper has been published: https://doi.org/10.1007/s12026-024-09467-8
References
Havel JJ, Chowell D, Chan TA. The evolving landscape of biomarkers for checkpoint inhibitor immunotherapy. Nat Rev Cancer. 2019;19(3):133–50.
Hanahan D. Hallmarks of cancer: new dimensions. Cancer Discov. 2022;12(1):31–46.
Kraehenbuehl L, Weng CH, Eghbali S, Wolchok JD, Merghoub T. Enhancing immunotherapy in cancer by targeting emerging immunomodulatory pathways. Nat Rev Clin Oncol. 2022;19(1):37–50.
Korman AJ, Garrett-Thomson SC, Lonberg N. The foundations of immune checkpoint blockade and the ipilimumab approval decennial. Nat Rev Drug Discovery. 2022;21(7):509–28.
Wang Y, Zhang H, Liu C, Wang Z, Wu W, Zhang N, et al. Immune checkpoint modulators in cancer immunotherapy: recent advances and emerging concepts. J Hematol Oncol. 2022;15(1):111.
Zou W, Chen L. Inhibitory B7-family molecules in the tumour microenvironment. Nat Rev Immunol. 2008;8(6):467–77.
Chapoval AI, Ni J, Lau JS, Wilcox RA, Flies DB, Liu D, et al. B7–H3: a costimulatory molecule for T cell activation and IFN-γ production. Nat Immunol. 2001;2(3):269–74.
Zhao B, Li H, Xia Y, Wang Y, Wang Y, Shi Y, et al. Immune checkpoint of B7–H3 in cancer: from immunology to clinical immunotherapy. J Hematol Oncol. 2022;15(1):153.
Kontos F, Michelakos T, Kurokawa T, Sadagopan A, Schwab JH, Ferrone CR, et al. B7–H3: an attractive target for antibody-based immunotherapy. Clin Cancer Res. 2021;27(5):1227–35.
Kanchan RK, Doss D, Khan P, Nasser MW, Mahapatra S. To kill a cancer: targeting the immune inhibitory checkpoint molecule, B7–H3. Biochim Biophys Acta Rev Cancer. 2022;1877(5):188783.
Getu AA, Tigabu A, Zhou M, Lu J, Fodstad O, Tan M. New frontiers in immune checkpoint B7–H3 (CD276) research and drug development. Mol Cancer. 2023;22(1):43.
Vigdorovich V, Ramagopal UA, Lazar-Molnar E, Sylvestre E, Lee JS, Hofmeyer KA, et al. Structure and T cell inhibition properties of B7 family member, B7–H3. Structure. 2013;21(5):707–17.
Zhou WT, Jin WL. B7–H3/CD276: an emerging cancer immunotherapy. Front Immunol. 2021;12:701006.
Shenderov E, De Marzo AM, Lotan TL, Wang H, Chan S, Lim SJ, et al. Neoadjuvant enoblituzumab in localized prostate cancer: a single-arm, phase 2 trial. Nat Med. 2023;29(4):888–97.
Aggarwal C, Prawira A, Antonia S, Rahma O, Tolcher A, Cohen RB, et al. Dual checkpoint targeting of B7–H3 and PD-1 with enoblituzumab and pembrolizumab in advanced solid tumors: interim results from a multicenter phase I/II trial. J Immunother Cancer. 2022;10:4.
Patel MR, Doi T, Koyama T, Falchook GS, Friedman CF, Piha-Paul SA, et al. (2023) 690P Ifinatamab deruxtecan (I-DXd; DS-7300) in patients with advanced solid tumors: updated clinical and biomarker results from a phase I/II study. Annals of Oncology. 34:S481-S2
Pulanco MC, Madsen AT, Tanwar A, Corrigan DT, Zang X. Recent advancements in the B7/CD28 immune checkpoint families: new biology and clinical therapeutic strategies. Cell Mol Immunol. 2023;20(7):694–713.
Hwang J, Zorko N, Elliott A, Lozada JA, Radovich M, Heath EI, et al. Pan-cancer associations of B7–H3 (CD276) transcriptional expression across human malignancies. J Clin Oncol. 2023;41(16):2624.
Heather LM, Azin S, Andrew E, Ben XW, Sarah Rachel K, Patricia AS, et al. High expression of B7–H3 on stromal cells defines tumor and stromal compartments in epithelial ovarian cancer and is associated with limited immune activation. J Immunother Cancer. 2019;7(1):357.
Costa A, Kieffer Y, Scholer-Dahirel A, Pelon F, Bourachot B, Cardon M, et al. Fibroblast heterogeneity and immunosuppressive environment in human breast cancer. Cancer Cell. 2018;33(3):463–7910.
Aung PP, Parra ER, Barua S, Sui D, Ning J, Mino B, et al. B7–H3 Expression in merkel cell carcinoma-associated endothelial cells correlates with locally aggressive primary tumor features and increased vascular density. Clin Cancer Res. 2019;25(11):3455–67.
Zhang X, Fang C, Zhang G, Jiang F, Wang L, Hou J. Prognostic value of B7–H3 expression in patients with solid tumors: a meta-analysis. Oncotarget. 2017;8(54):93156–67.
Zang X, Thompson RH, Al-Ahmadie HA, Serio AM, Reuter VE, Eastham JA, et al. B7–H3 and B7x are highly expressed in human prostate cancer and associated with disease spread and poor outcome. Proc Natl Acad Sci. 2007;104(49):19458–63.
Tyagi A, Ly S, El-Dana F, Yuan B, Jaggupilli A, Grimm S, et al. Evidence supporting a role for the immune checkpoint protein B7–H3 in NK cell-mediated cytotoxicity against AML. Blood. 2022;139(18):2782–96.
Wang L, Zhang Q, Chen W, Shan B, Ding Y, Zhang G, et al. B7–H3 is overexpressed in patients suffering osteosarcoma and associated with tumor aggressiveness and metastasis. PLoS ONE. 2013;8(8):e70689.
Kim Nah I, Park Min H, Kweon S-S, Lee Ji S. B7–H3 and B7–H4 expression in breast cancer and their association with clinicopathological variables and T cell infiltration. Pathobiology. 2020;87(3):179–92.
Lin W, Xu Y, Gao J, Zhang H, Sun Y, Qiu X, et al. Multi-omics data analyses identify B7–H3 as a novel prognostic biomarker and predict response to immune checkpoint blockade in head and neck squamous cell carcinoma. Front Immunol. 2021;12:757047.
Zhao S, Wang Y, Yang N, Mu M, Wu Z, Li H, et al. Genome-scale CRISPR-Cas9 screen reveals novel regulators of B7–H3 in tumor cells. J Immunother Cancer. 2022;10:6.
Chen Y, Wu Y, Yang H, Wang J, Kong J, Yu L, et al. DNA methylation and mRNA expression of B7–H3 gene in ankylosing spondylitis: a case-control study. Immunol Invest. 2022;51(7):2025–34.
Huang Y, Zhang H-L, Li Z-L, Du T, Chen Y-H, Wang Y, et al. FUT8-mediated aberrant N-glycosylation of B7H3 suppresses the immune response in triple-negative breast cancer. Nat Commun. 2021;12(1):2672.
Ahangar NK, Hemmat N, Khalaj-Kondori M, Shadbad MA, Sabaie H, Mokhtarzadeh A, et al. The regulatory cross-talk between microRNAs and novel members of the B7 family in human diseases: a scoping review. Int J Mol Sci. 2021;22:5.
Zhao J, Lei T, Xu C, Li H, Ma W, Yang Y, et al. MicroRNA-187, down-regulated in clear cell renal cell carcinoma and associated with lower survival, inhibits cell growth and migration though targeting B7–H3. Biochem Biophys Res Commun. 2013;438(2):439–44.
Hu X, Xu M, Hu Y, Li N, Zhou L. B7–H3, negatively regulated by miR-128, promotes colorectal cancer cell proliferation and migration. Cell Biochem Biophys. 2021;79(2):397–405.
Yang X, Feng KX, Li H, Wang L, Xia H. MicroRNA-199a inhibits cell proliferation, migration, and invasion and activates AKT/mTOR signaling pathway by targeting B7–H3 in cervical cancer. Technol Cancer Res Treat. 2020;19:1533033820942245.
Wang S, Mou J, Cui L, Wang X, Zhang Z. Astragaloside IV inhibits cell proliferation of colorectal cancer cell lines through down-regulation of B7–H3. Biomed Pharmacother. 2018;102:1037–44.
Liu Z, Zhang W, Phillips JB, Arora R, McClellan S, Li J, et al. Immunoregulatory protein B7–H3 regulates cancer stem cell enrichment and drug resistance through MVP-mediated MEK activation. Oncogene. 2019;38(1):88–102.
Liao H, Ding M, Zhou N, Yang Y, Chen L. B7H3 promotes the epithelialmesenchymal transition of NSCLC by targeting SIRT1 through the PI3K/AKT pathway. Mol Med Rep. 2022;25:3.
Lim S, Liu H, Madeira da Silva L, Arora R, Liu Z, Phillips JB, et al. Immunoregulatory protein B7–H3 reprograms glucose metabolism in cancer cells by ROS-mediated stabilization of HIF1alpha. Cancer Res. 2016;76(8):2231–42.
Nunes-Xavier CE, Karlsen KF, Tekle C, Pedersen C, Oyjord T, Hongisto V, et al. Decreased expression of B7–H3 reduces the glycolytic capacity and sensitizes breast cancer cells to AKT/mTOR inhibitors. Oncotarget. 2016;7(6):6891–901.
Li Z, Liu J, Que L, Tang X. The immunoregulatory protein B7–H3 promotes aerobic glycolysis in oral squamous carcinoma via PI3K/Akt/mTOR pathway. J Cancer. 2019;10(23):5770–84.
Cheng N, Bei Y, Song Y, Zhang W, Xu L, Zhang W, et al. B7–H3 augments the pro-angiogenic function of tumor-associated macrophages and acts as a novel adjuvant target for triple-negative breast cancer therapy. Biochem Pharmacol. 2021;183:114298.
Cheng R, Chen Y, Zhou H, Wang B, Du Q, Chen Y. B7–H3 expression and its correlation with clinicopathologic features, angiogenesis, and prognosis in intrahepatic cholangiocarcinoma. APMIS. 2018;126(5):396–402.
Wang R, Ma Y, Zhan S, Zhang G, Cao L, Zhang X, et al. B7–H3 promotes colorectal cancer angiogenesis through activating the NF- kappaB pathway to induce VEGFA expression. Cell Death Dis. 2020;11(1):55.
Han S, Wang Y, Shi X, Zong L, Liu L, Zhang J, et al. Negative roles of B7–H3 and B7–H4 in the microenvironment of cervical cancer. Exp Cell Res. 2018;371(1):222–30.
Lu H, Shi T, Wang M, Li X, Gu Y, Zhang X, et al. B7–H3 inhibits the IFN-gamma-dependent cytotoxicity of Vgamma9Vdelta2 T cells against colon cancer cells. Oncoimmunology. 2020;9(1):1748991.
Si S, Wang L, Cao H, Xu Y, Zhan Q. Co-deficiency of B7–H3 and B7–H4 identifies high CD8 + T cell infiltration and better prognosis in pancreatic cancer. BMC Cancer. 2022;22(1):211.
Lee CC, Ho KH, Huang TW, Shih CM, Hsu SY, Liu AJ, et al. A regulatory loop among CD276, miR-29c-3p, and Myc exists in cancer cells against natural killer cell cytotoxicity. Life Sci. 2021;277:119438.
Miyamoto T, Murakami R, Hamanishi J, Tanigaki K, Hosoe Y, Mise N, et al. B7–H3 suppresses antitumor immunity via the CCL2-CCR2-M2 macrophage axis and contributes to ovarian cancer progression. Cancer Immunol Res. 2022;10(1):56–69.
Jian An H, Cheng C, Yibei Z, Xueguang Z. Induced expression of B7–H3 on the lung cancer cells and macrophages suppresses tumor-specific T cell immunity. Eur Respir J. 2012;40(Suppl 56):P1221.
Inamura K, Amori G, Yuasa T, Yamamoto S, Yonese J, Ishikawa Y. Relationship of B7–H3 expression in tumor cells and tumor vasculature with FOXP3+ regulatory T cells in renal cell carcinoma. Cancer Manag Res. 2019;11:7021–30.
Yim J, Koh J, Kim S, Song SG, Ahn HK, Kim YA, et al. Effects of B7–H3 expression on tumour-infiltrating immune cells and clinicopathological characteristics in non-small-cell lung cancer. Eur J Cancer. 2020;133:74–85.
Luo L, Chapoval AI, Flies DB, Zhu G, Hirano F, Wang S, et al. B7–H3 enhances tumor immunity in vivo by costimulating rapid clonal expansion of antigen-specific CD8+ cytolytic T cells. J Immunol. 2004;173(9):5445–50.
Cheon H, Wang Y, Wightman SM, Jackson MW, Stark GR. How cancer cells make and respond to interferon-I. Trends Cancer. 2023;9(1):83–92.
Boukhaled GM, Harding S, Brooks DG. Opposing roles of type i interferons in cancer immunity. Annu Rev Pathol. 2021;16:167–98.
Jorgovanovic D, Song M, Wang L, Zhang Y. Roles of IFN-gamma in tumor progression and regression: a review. Biomark Res. 2020;8:49.
Meissl K, Macho-Maschler S, Muller M, Strobl B. The good and the bad faces of STAT1 in solid tumours. Cytokine. 2017;89:12–20.
Cheon H, Holvey-Bates EG, Schoggins JW, Forster S, Hertzog P, Imanaka N, et al. IFNbeta-dependent increases in STAT1, STAT2, and IRF9 mediate resistance to viruses and DNA damage. Embo j. 2013;32(20):2751–63.
Cheon H, Holvey-Bates EG, McGrail DJ, Stark GR. PD-L1 sustains chronic, cancer cell-intrinsic responses to type I interferon, enhancing resistance to DNA damage. Proc Natl Acad Sci U S A. 2021;118:47.
Gato-Canas M, Zuazo M, Arasanz H, Ibanez-Vea M, Lorenzo L, Fernandez-Hinojal G, et al. PDL1 signals through conserved sequence motifs to overcome interferon- mediated cytotoxicity. Cell Rep. 2017;20(8):1818–29.
Liu HJ, Du H, Khabibullin D, Zarei M, Wei K, Freeman GJ, et al. mTORC1 upregulates B7–H3/CD276 to inhibit antitumor T cells and drive tumor immune evasion. Nat Commun. 2023;14(1):1214.
Huang M, Luo J, Ji X, Hu M, Xue Y, Liu Q. Deficiency of tumor-expressed B7–H3 augments anti-tumor efficacy of anti- PD-L1 monotherapy rather than the combined chemoimmunotherapy in ovarian cancer. Pharmacol Res. 2022;186:106512.
Purvis IJ, Avilala J, Guda MR, Venkataraman S, Vibhakar R, Tsung AJ, et al. Role of MYC-miR-29-B7-H3 in medulloblastoma growth and angiogenesis. J Clin Med. 2019;8:8.
Oh Y, Park R, Kim SY, Park SH, Jo S, Kim TH, et al. B7–H3 regulates osteoclast differentiation via type I interferon- dependent IDO induction. Cell Death Dis. 2021;12(11):971.
Costa-Pereira AP, Tininini S, Strobl B, Alonzi T, Schlaak JF, Is’harc H, et al. Mutational switch of an IL-6 response to an interferon-gamma-like response. Proc Natl Acad Sci U S A. 2002;99(12):8043–7.
Herrero C, Hu X, Li WP, Samuels S, Sharif MN, Kotenko S, et al. Reprogramming of IL-10 activity and signaling by IFN-gamma. J Immunol. 2003;171(10):5034–41.
Sharif MN, Tassiulas I, Hu Y, Mecklenbrauker I, Tarakhovsky A, Ivashkiv LB. IFN-alpha priming results in a gain of proinflammatory function by IL-10: implications for systemic lupus erythematosus pathogenesis. J Immunol. 2004;172(10):6476–81.
Ho HH, Ivashkiv LB. Role of STAT3 in type I interferon responses .Negative regulation of STAT1-dependent inflammatory gene activation. J Biol Chem. 2006;281(20):14111–8.
Yan J, Wang ZY, Yang HZ, Liu HZ, Mi S, Lv XX, et al. Timing is critical for an effective anti-metastatic immunotherapy: the decisive role of IFNgamma/STAT1-mediated activation of autophagy. PLoS ONE. 2011;6(9):e24705.
Regis G, Pensa S, Boselli D, Novelli F, Poli V. Ups and downs: the STAT1:STAT3 seesaw of Interferon and gp130 receptor signalling. Semin Cell Dev Biol. 2008;19(4):351–9.
Li G, Quan Y, Che F, Wang L. B7–H3 in tumors: friend or foe for tumor immunity? Cancer Chemother Pharmacol. 2018;81(2):245–53.
Cai D, Li J, Liu D, Hong S, Qiao Q, Sun Q, et al. Tumor-expressed B7–H3 mediates the inhibition of antitumor T-cell functions in ovarian cancer insensitive to PD-1 blockade therapy. Cell Mol Immunol. 2020;17(3):227–36.
Lee YH, Martin-Orozco N, Zheng P, Li J, Zhang P, Tan H, et al. Inhibition of the B7–H3 immune checkpoint limits tumor growth by enhancing cytotoxic lymphocyte function. Cell Res. 2017;27(8):1034–45.
Sun Y, Wang Y, Zhao J, Gu M, Giscombe R, Lefvert AK, et al. B7–H3 and B7–H4 expression in non-small-cell lung cancer. Lung Cancer. 2006;53(2):143–51.
Deng J, Ma M, Wang D, Zhu H, Hua L, Sun S, et al. Expression and clinical significance of immune checkpoint regulator B7–H3 (CD276) in human meningioma. World Neurosurg. 2020;135:e12–8.
Qin X, Sun W, Wang C, Li M, Zhao X, Li C, et al. Mifepristone inhibited the expression of B7–H2, B7–H3, B7–H4 and PD-L2 in adenomyosis. Reprod Biol Endocrinol. 2021;19(1):114.
Saeednejad Zanjani L, Madjd Z, Axcrona U, Abolhasani M, Rasti A, Asgari M, et al. Cytoplasmic expression of B7–H3 and membranous EpCAM expression are associated with higher grade and survival outcomes in patients with clear cell renal cell carcinoma. Ann Diagn Pathol. 2020;46:151483.
Ingebrigtsen VA, Boye K, Tekle C, Nesland JM, Flatmark K, Fodstad O. B7–H3 expression in colorectal cancer: nuclear localization strongly predicts poor outcome in colon cancer. Int J Cancer. 2012;131(11):2528–36.
Yonesaka K, Haratani K, Takamura S, Sakai H, Kato R, Takegawa N, et al. B7–H3 negatively modulates CTL-mediated cancer immunity. Clin Cancer Res. 2018;24(11):2653–64.
Shi W, Wang Y, Zhao Y, Kim JJ, Li H, Meng C, et al. Immune checkpoint B7–H3 is a therapeutic vulnerability in prostate cancer harboring PTEN and TP53 deficiencies. Sci Transl Med. 2023;15(695):eadf6724.
Kang FB, Wang L, Li D, Zhang YG, Sun DX. Hepatocellular carcinomas promote tumor-associated macrophage M2-polarization via increased B7–H3 expression. Oncol Rep. 2015;33(1):274–82.
Reya T, Morrison SJ, Clarke MF, Weissman IL. Stem cells, cancer, and cancer stem cells. Nature. 2001;414(6859):105–11.
Ailles LE, Weissman IL. Cancer stem cells in solid tumors. Curr Opin Biotechnol. 2007;18(5):460–6.
Vetrie D, Helgason GV, Copland M. The leukaemia stem cell: similarities, differences and clinical prospects in CML and AML. Nat Rev Cancer. 2020;20(3):158–73.
Zhang L-N, Zhang D-D, Yang L, Gu Y-X, Zuo Q-P, Wang H-Y, et al. Roles of cell fusion between mesenchymal stromal/stem cells and malignant cells in tumor growth and metastasis. FEBS J. 2021;288(5):1447–56.
Dittmar T, Hass R. Intrinsic signalling factors associated with cancer cell-cell fusion. Cell Commun Signal. 2023;21(1):68.
Gast CE, Silk AD, Zarour L, Riegler L, Burkhart JG, Gustafson KT, et al. Cell fusion potentiates tumor heterogeneity and reveals circulating hybrid cells that correlate with stage and survival. Sci Adv. 2018;4(9):eaat7828.
Cozzo AJ, Coleman MF, Hursting SD. You complete me: tumor cell-myeloid cell nuclear fusion as a facilitator of organ-specific metastasis. Front Oncol. 2023;13:1191332.
Ahmadzadeh K, Pereira M, Vanoppen M, Bernaerts E, Ko JH, Mitera T, et al. Multinucleation resets human macrophages for specialized functions at the expense of their identity. EMBO Rep. 2023;24(3):e56310.
Clawson GA, Matters GL, Xin P, Imamura-Kawasawa Y, Du Z, Thiboutot DM, et al. Macrophage-tumor cell fusions from peripheral blood of melanoma patients. PLoS ONE. 2015;10(8):e0134320.
Aguirre LA, Montalban-Hernandez K, Avendano-Ortiz J, Marin E, Lozano R, Toledano V, et al. Tumor stem cells fuse with monocytes to form highly invasive tumor-hybrid cells. Oncoimmunology. 2020;9(1):1773204.
Brito A, Merle C, Lagarde P, Faustin B, Devin A, Lartigue L, et al. Cell fusion enhances energy metabolism of mesenchymal tumor hybrid cells to sustain their proliferation and invasion. BMC Cancer. 2021;21(1):863.
Beziaud L, Young CM, Alonso AM, Norkin M, Minafra AR, Huelsken J. IFNγ-induced stem-like state of cancer cells as a driver of metastatic progression following immunotherapy. Cell Stem Cell. 2023;30(6):818-31.e6.
Jones DH, Nakashima T, Sanchez OH, Kozieradzki I, Komarova SV, Sarosi I, et al. Regulation of cancer cell migration and bone metastasis by RANKL. Nature. 2006;440(7084):692–6.
Li M, Basile JR, Mallya S, Lin YL. The impact and outcomes of cancer-macrophage fusion. BMC Cancer. 2023;23(1):497.
Sambandam Y, Ethiraj P, Hathaway-Schrader JD, Novince CM, Panneerselvam E, Sundaram K, et al. Autoregulation of RANK ligand in oral squamous cell carcinoma tumor cells. J Cell Physiol. 2018;233(8):6125–34.
Gao Y, Fang P, Li WJ, Zhang J, Wang GP, Jiang DF, et al. LncRNA NEAT1 sponges miR-214 to regulate M2 macrophage polarization by regulation of B7–H3 in multiple myeloma. Mol Immunol. 2020;117:20–8.
Zuo J, Wang B, Long M, Gao Z, Zhang Z, Wang H, et al. The type 1 transmembrane glycoprotein B7–H3 interacts with the glycolytic enzyme ENO1 to promote malignancy and glycolysis in HeLa cells. FEBS Lett. 2018;592(14):2476–88.
Shi T, Ma Y, Cao L, Zhan S, Xu Y, Fu F, et al. B7–H3 promotes aerobic glycolysis and chemoresistance in colorectal cancer cells by regulating HK2. Cell Death Dis. 2019;10(4):308.
Wang C, Li Y, Jia L, Kim JK, Li J, Deng P, et al. CD276 expression enables squamous cell carcinoma stem cells to evade immune surveillance. Cell Stem Cell. 2021;28(9):1597-613.e7.
Funding
This research was supported by the Basic Science Research Program through the National Research Foundation of Korea (NRF) funded by the Ministry of Science, ICT, and Future (2022R1A2C1003860 to Jong Dae Ji).
Author information
Authors and Affiliations
Contributions
Conceptualization: R.P. and J.J.; validation: R.P., J.Y., M.S., S.L., and J.J.; writing—original draft preparation: R.P. and J.J.; writing—review and editing: R.P., J.Y., M.S., S.L., and J.J.; visualization: R.P.; supervision: J.J.; project administration: R.P. and J.J. All authors have read and agreed to the published version of the manuscript.
Corresponding author
Ethics declarations
Ethics approval
Not applicable.
Consent for publication
Not applicable.
Competing interests
The authors declare no competing interests.
Additional information
Publisher's Note
Springer Nature remains neutral with regard to jurisdictional claims in published maps and institutional affiliations.
The original online version of this article was revised: A spelling error was identified in an author’s name, which has been corrected. Namely, Dr. Moazzam Shazad’s name has been corrected to “Shahzad”. The affiliation 1 were corrected from “Department of Hematology/Oncology, Moffitt Cancer Center, Tampa, FL, USA” to “Department of Hematology/Oncology, Moffitt Cancer Center/University of South Florida, Tampa, FL, USA.
Rights and permissions
Springer Nature or its licensor (e.g. a society or other partner) holds exclusive rights to this article under a publishing agreement with the author(s) or other rightsholder(s); author self-archiving of the accepted manuscript version of this article is solely governed by the terms of such publishing agreement and applicable law.
About this article
Cite this article
Park, R., Yu, J., Shahzad, M. et al. The immune regulatory function of B7-H3 in malignancy: spotlight on the IFN-STAT1 axis and regulation of tumor-associated macrophages. Immunol Res (2024). https://doi.org/10.1007/s12026-024-09458-9
Received:
Accepted:
Published:
DOI: https://doi.org/10.1007/s12026-024-09458-9