Abstract
Peptidic natural products have received much attention as potential drug leads, and biosynthetic studies of peptidic natural products have contributed to the field of natural product chemistry over the past several decades. However, the key biosynthetic intermediates are generally not isolated from natural sources, and this can hamper a detailed analysis of biosynthesis. Furthermore, reported unusual structures, which are targets for biosynthetic studies, are sometimes the results of structural misassignments. Chemical synthesis techniques are imperative in solving these problems. This review focuses on the chemical syntheses of surugamides and thioamycolamides toward understanding their biosynthesis. These studies can provide the key biosynthetic intermediates that can reveal the biosynthetic pathways and/or true structures of these natural products.
Graphical abstract

Similar content being viewed by others
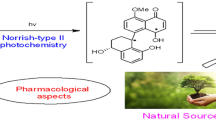
Avoid common mistakes on your manuscript.
Introduction
Small peptides (molecular weight ranging from 500 to 6000 Daltons), which have physicochemical and biological properties intermediate between those of antibodies and small molecules [1], have received much attention as ideal drug leads. The specific bioactivity of a peptide drug lead is a result of its particular structures [2], and an unprecedented structure would be constructed by a novel biosynthetic pathway.
Over the last few decades, biosynthetic studies of peptidic natural products have played a pivotal role in the discovery of new drug leads. Homology searching of biosynthetic enzymes and/or genetic manipulation of the biosynthetic processes can lead to the discovery of cryptic natural products [3]. However, the key biosynthetic intermediates, namely the substrates of the unusual enzymes, are generally not isolated from the natural sources, and this hampers the mechanistic analysis of the new biosynthetic enzymes. Additionally, the reported structures of new natural products with unusual features, which are fascinating targets for biosynthetic studies, are sometimes turned out to be the products of structural misassignments [4]. Chemical synthesis techniques are imperative for solving these problems.
There have been many reviews on the total syntheses of peptide natural products, particularly regarding synthetic methodologies, but this review highlights the total syntheses of peptidic natural products that provide the synthetic entry to the key biosynthetic intermediates to reveal the biosynthetic pathways and determine the correct structures of unusual peptides.
Total synthesis of surugamide B and identification of a new offloading cyclase family
The cyclic octapeptides surugamides A–E (Fig. 1, 1–5) were isolated from the marine actinomycete Streptomyces sp. JAMM992 [5]. In 2016, the biosynthetic gene cluster of surugamides was identified, which led to the discovery of the new linear decapeptide surugamide F (6) [6]. The cyclic peptides 1–5 show cathepsin B inhibitory activity, whereas the biological activity of the newly isolated 6 has not yet been evaluated. These cyclic and acyclic peptides are generated by a single gene cluster consisting of four successive non-ribosomal peptide synthetases (NRPSs), surA, surB, surC, and surD. Among these four NRPSs, surB and surC produce linear decapeptide 6, which are sandwiched by the genes for 1–5 (surA and surD). Additionally, thioesterase (TE) domain, which terminates the peptide chain elongation by the hydrolysis/cyclization, was not identified at the terminus of this gene cluster [7]. Because of their unique NRPS genes, there has been much interest in the biological activity and also the biosynthetic mechanisms of the surugamides. However, the chemical synthesis for the structural confirmation and material supply of surugamides were not reported until 2018.
In 2018, Wakimoto and co-workers reported the first total synthesis of surugamide B (2). The development of an efficient synthetic route to 2 enabled the synthesis of the acyclic biosynthetic precursor, which led to the identification of a new offloading cyclase family [8].
The challenge in the synthesis of surugamides lies in the cyclization of the peptide, which is a long-standing problem for chemists [9]. The biggest obstacle in peptide chemical synthesis is the Cα epimerization, especially in the synthesis of cyclic peptides consisting of totally epimerizable α-amino acids, such as surugamides A–E (1–5). To minimize the side reactions including the isomerization, these cyclic peptides are usually synthesized with the optimization of the chemical reaction conditions (e.g., coupling reagent, solvent, and cyclization site) [10]. In contrast, the organisms generally produce cyclic peptides as stereochemically pure form, and surugamide B (2) is biosynthesized without detectable isomerization. Therefore, the synthesis of the cyclic peptide surugamide B was designed on the basis of the biosynthesis, similar to the preceding work on a cyclic depsipeptide theonellapeptolide Id [11].
The partly deciphered biosynthesis of 2 is illustrated in Fig. 2. However, the cyclization mechanism of 2 had not yet been elucidated due to the lack of TE domain. Wakimoto and co-workers identified the start and end points of the peptide synthesis. Namely, the biosynthesis of the linear peptide is initiated by the substrate-specific recognition of l-Ile by the first A domain (A1) and finishes with the attachment of l-Leu and the stereochemical inversion to d-Leu by the terminal E domain. Then, intramolecular amidation between l-Ile and d-Leu gives 2 without epimerization at the C-terminus.
Wakimoto’s plan for the total synthesis is summarized in Fig. 3. Using the genomic analysis, the peptide 2 was retrosynthetically linearized to 7. The cyclization site was definitively determined from the genome analysis. The linear peptide 7 was then synthesized by the solid-phase peptide synthesis [12].
The synthesis of 2 commenced with the treatment of Fmoc-d-Leu loaded 2-chlorotrityl resin (9) with piperidine to liberate the amine 10. Then, seven cycles of DIC/Oxyma [13]-mediated amide coupling and Nα-deprotection were applied to 10, leading to 8. The cleavage of 8 from the resin was realized by the use of (CF3)2CHOH–CH2Cl2 (3:7) without the deprotection of the side-chain. The liberated acyclic peptide was then biomimetically cyclized using PyBOP [14]/HOAt [15] as coupling reagents to give cyclic peptide 11. Finally, treatment of 11 with TFA-iPr3SiH-H2O (95:2.5:2.5) afforded 2 with trace isomerization (dr = > 25:1). After ODS-HPLC purification, 2 was obtained in 34% yield in 18 steps. The average yield per step in the total synthesis was 94%, which also supported the efficiency of the genome analysis-guided chemical synthesis (Fig. 4).
Having developed the biomimetic entry to 2, Wakimoto and co-workers then elucidated the biosynthetic mechanism of surugamides. Considering the lack of a TE domain in the NRPS module of surugamide B, the non-enzymatic cyclizaition of the linear biosynthetic precursor was first examined. To investigate the cyclization mechanism, the N-acetylcysteamine (SNAC) thioester 16 was chemically synthesized as a mimic of the peptidyl carrier protein-bound peptide (Fig. 5) [16]. The resin-bound peptide 14 was synthesized in the same manner as the total synthesis of 2 utilizing the safety-catch linker strategy [17]. The sulfonamide of 14 was activated by TMS-diazomethane, and then reacted with SNAC to give thioester 15. Then, the Boc group of 15 was removed by the action of TFA to deliver the linear biosynthetic precursor 16.
Initially, a non-enzymatic cyclization of the 16 was performed in the presence of Et3N. However, the hydrolysis of the thioester was faster than the cyclization, and a considerable amount of isopeptide 17 was detected. These data strongly suggested that the head-to-side chain cyclization is more favorable in the non-enzymatic conditions, and an as yet unidentified peptide thioester cyclase is required for the head-to-tail macrocyclization in the later stage of the biosynthesis of 2. The candidate genes for the off-loading enzyme would be SurE, which is encoded just upstream of SurA. The enzyme exhibits sequential similarity with penicillin-binding protein (PBP), which is a group of enzymes responsible for the transpeptidation step in the biosynthesis of bacterial cell wall peptide glycan [18]. To analyze the function of SurE, the enzyme was cloned into the pET-28a vector and expressed in the E. coli BL21 as a His-tagged protein, and then purified. When the recombinant SurE was mixed with 16, the linear precursor 16 was efficiently transformed into 2 without any detectable by-products. These results strongly indicated that SurE plays a role in both chain termination and macrocyclization in the biosynthesis of surugamides A–E (1–5).
Although this section focuses on the chemical synthesis of surugamide B (2) and its biosynthetic precursors, Wakimoto and co-workers also reported the mechanistic studies of SurE after accomplishment of the total synthesis [19, 20]. Furthermore, the database search identified several homologues of SurE in the biosynthetic gene cluster of NRP such as mannopeptimycins [21], desotamides [22], ulleungmycins [23], and noursamycins [24]. Thus the total synthesis of a peptide natural product had opened an avenue for the discovery and the detailed functional analysis of a new cyclase family in the NRPSs.
Total synthesis and structural revision of surugamide A
Another structural feature of surugamides is the d-Ile residue present in 1 and 3–5 (Fig. 1, 2 is a derivative with d-Val at this position). d-Ile is the enantiomer of l-Ile with the epimerization at both Cα-, and Cβ-positions. The epimerization at the Cα-position is commonly observed in the non-ribosomal peptide (NRP), and the Cα-position of Ile is likely to be epimerized by the E-domain in the case of surugamides biosynthesis. However, the biosynthetic mechanism for the epimerization at the Cβ-position is obscure (Fig. 6).
The epimerization at the Cβ-position is very rare in nature [22, 25, 26]. Therefore, before launching an investigation into the biosynthesis of d-Ile residue, Wakimoto and co-workers first synthesized d-Ile-containing peptide 1a to confirm the existence of d-Ile in 1 by taking advantage of the established route for the total synthesis of 2 (vide supra, Fig. 3). However, synthesized 1a was not identical to natural 1 in the HPLC experiments, therefore, the reported structures of cyclic octapeptide surugamides 1 and 3–5 needed to be corrected. To determine the structure of 1, solid phase peptide synthesis of d-allo-Ile-containing 1b was also conducted (Fig. 7) [27].
The Fmoc group of 9 was removed by the treatment with piperidine to afford 10, then three rounds of DIC/Oxyma-mediated amidation and Nα-deprotection with piperidine yielded resin-bound tetrapeptide 18. The amine 18 was first conjugated to Fmoc-d-Ile-OH to afford 19a, and a further three rounds of solid-phase peptide synthesis (SPPS) was performed to generate the resin-bound octapeptide (21a). Successive treatment of 21a with (CF3)2CHOH cleaved the peptide from the resin, and the peptide was subsequently cyclized by using PyBOP and HOAt to give the cyclic peptide. Finally, the peptide was treated with TFA/i-Pr3SiH/H2O (95:2.5:2.5) to remove the Boc group, which resulted in the originally reported structure of surugamide A (1a). However, the HPLC analysis revealed that synthetic 1a was not identical to natural surugamide A. To reveal the true structure of 1, d-allo-Ile-containing peptide 1b was synthesized in the same manner as 1a. Expectedly, the HPLC analysis showed that 1b and natural 1 were identical.
In the original structural elucidation [5], the configuration of Ile was identified by a subtle chromatographic difference of the 2,3,4,6-tetra-O-acetyl-β-d-glucopyranosyl isothiocyanate (GITC) derivatives [28, 29], resulting in the misassignment. In Wakimoto’s study, the synthetic efforts confirmed that 1 contains a d-allo-Ile residue instead of d-Ile. Because of the commonality of the biosynthetic pathway, the d-Ile residue previously identified in other derivatives such as 3–5 should also be corrected to d-allo-Ile.
This section highlights the problem that the reported unusual structures of new natural products, especially targets for biosynthetic studies, are sometimes misassignments. As is the case with non-peptidic natural products, chemical synthesis plays an indispensable role in structural confirmation [4].
Total synthesis of thioamycolamide A toward understanding the thioether biosynthesis
Organosulfur compounds are of interest in organic chemistry because of their particular bioactivities and biosynthses [30,31,32]. In 2020, Kakeya and co-workers reported the identification of the rare sulfur-containing cyclic lipopeptide thioamycolamide A (22, Fig. 8) along with the minor analogues thioamycolamides B–E (23–26) from the culture broth of Amycolatopsis sp. 26-4 [33]. The peptide 22 showed moderate inhibitory activities against several human cancer cell lines, and the chemical structure of 22 was established by a combination of spectroscopic analyses and the chemical synthesis of its partial structure. The cyclic skeletal structure of 22 contains a d-configured thiazoline, a thioether bridge, a fatty acid-side chain, and a reduced C-terminus.
While the highly modified structure of 22 is of interest to chemists, biosynthetically unusual structures are sometimes the result of structural misassignments. Therefore, the unprecedented structure of 22 needed to be confirmed by chemical synthesis. However, although several total syntheses of natural products that bear thiazoline rings [34, 35] or a reduced C-terminus [36] had been accomplished, the total synthesis of thioamycolamides was not reported until 2021.
In 2021, Kakeya and co-workers reported a concise total synthesis of 22, which was designed based on the putative biosynthetic pathway, that corroborated the structure of 22 and the biosynthesis of the thioether bridge formation [37]. The retrosynthesis of 22 is summarized in Fig. 9. While the biosynthetic gene cluster has not been identified yet, the structural features of 22 suggest that this cytotoxin is assembled by an NRPS as illustrated in Fig. 9. Using this plausible biosynthesis as a guide, the macrolactam 22 was retrosynthetically acyclized to 27. Then, the linear peptide 27 could be synthesized by the assembly of components 28–31 using isomerization-suppression procedures.
The challenge in the total synthesis of 22 had arose from the highly epimerizable nature of the thiazoline moiety [38,39,40,41]. Accordingly, the synthesis of the linear peptide 27 had to be constructed using limited reactions that would suppress the isomerization of the thiazoline.
To overcome these problems, the total synthesis of 22 started from the chemical construction of the thiazoline moiety using Wipf’s cyclodehydration of β-thioamide [42] as the key reaction (Fig. 10). Initially, Boc-l-Phe-OH (32) was amidated with 33 to give 34. The hydroxy function of 34 was protected by the TBS group to form 35, and then dipeptide 35 was transformed into the corresponding thioamide 36 by the action of Lawesson’s reagent [43, 44]. To avoid the acid-promoted epimerization of the thiazoline, the fatty acid was attached ahead of thiazoline formation, i.e., the Boc group of 36 was selectively removed in the presence of the TBS group by the treatment with TMSOTf [45], and then the liberated amine 37 was condensed with acid 28. At this stage, Kakeya and co-workers then attempted to transform a thioamide into thiazoline to prevent thiazolinone formation [46]. The undesired 1,4-additions to the α,β-unsaturated amide [47] of 38 also needed to be avoided. The TBS group of 38 was cleaved by TBAF/AcOH followed by successful cyclodehydration using diethylaminosulfur trifluoride (DAST) to provide thiazoline 40. Finally, 40 was converted to carboxylic acid 41 by Nicolaou’s method [48] without appreciable thiazoline isomerization in the reaction. However, it should be noted that the epimerization of acid 41 was observed during silica gel column chromatography. Therefore, 41 had to be used immediately without chromatographic purification.
With stereochemically pure 41 in hand, the total synthesis of 22 was accomplished by peptide chain elongation followed by bioinspired cyclization (Fig. 11). In Kakeya’s total synthesis, the dimeric cystine, from which the active thiol function can be liberated under mild reductive conditions, was used as an S-protected cysteine. Boc-l-cystine (42) was converted to the active ester, which was reduced in situ to alcohol 43 by NaBH4. The Boc group of 43 was removed by HCl, and then another building block 41 was attached to the N-terminus under EDCI·HCl/Oxyma conditions. Then, linear peptide 27 was successfully bridged by a thioether bond in pH 9 Na2CO3/NaHCO3 buffer containing TCEP·HCl [49] as a reductant. After reversed-phase ODS HPLC purification, 22 was obtained in 67% yield from 45. This bioinspired total synthesis provided support for the proposal that the thioether bridge of thioamycolamides is stereoselectively biosynthesized by thio-Michael addition without any specific enzymes.
Although the NMR spectra of synthetic 22 agreed with the spectra of natural 22, the spectroscopic data of peptides can vary depending on the solution conditions in the NMR tubes, and this often results in the structural misassignment of peptidic natural products [50,51,52,53]. An essential task at that stage was the structural confirmation of the chemically constructed 22 because the thio-Michael addition used in this synthesis may give 22 as a diastereomeric mixture due to the absence of a chiral catalyst. Notably, Kakeya and co-workers have recently developed several labeling reagents [54] inspired by the synthetic and structural studies on the peptidic natural products yaku’amides A and B [55, 56]. These highly sensitive labeling reagents are useful for the structural determination of peptides [57, 58] and identification of the scarce reactive natural products [59]. After the accomplished the total synthesis, the highly isomerizable structure of synthesized 22 was confirmed by utilizing their labeling reagents [60]. These experiments gave further support for the stereochemical purity of synthesized 22, providing evidence that the thioether bridge of 22 can be formed by thio-Michael addition without biosynthetic enzymes.
This section has summarized the chemical synthesis of a biosynthetic intermediate that is spontaneously transformed into the natural product, shedding light on a non-enzymatic pathway in its biosynthesis.
Conclusion
This review highlights the chemical syntheses of peptidic natural products that enabled synthetic entry to key biosynthetic intermediates, revealing the unique biosynthetic pathways and/or true structures of the natural products. The synthetic challenges involved in the construction of the cyclic octapeptide surugamide B (2) led to the discovery of a new cyclase family in the NRPSs; the synthesis of surugamide A (1) revealed the true structures of the surugamides, which have d-allo-Ile residues rather than d-Ile; and the biomimetic total synthesis of thioamycolamide A (22) demonstrated a non-enzymatic pathway for stereoselective thioether formation that may be present in its biosynthesis. Because biosynthetic studies on peptidic natural products are gaining importance in the identification of new natural products, the chemical synthesis of the key biosynthetic intermediates is becoming increasingly significant.
Although this review focuses on the chemical synthesis of non-ribosomal peptides (NRPs), the biosynthetic intermediates of ribosomal peptides [61] and non-peptidic natural products [62] can also be provided by the chemical synthesis, giving insights into the biosynthetic pathways of these natural products.
References
Apostolopoulos V, Bojarska J, Chai TT, Elnagdy S, Kaczmarek K, Matsoukas J, New R, Parang K, Lopez OP, Parhiz H, Perera CO, Pickholz M, Remko M, Saviano M, Skwarczynski M, Tang Y, Wolf WM, Yoshiya T, Zabrocki J, Zielenkiewicz P, AlKhazindar M, Barriga V, Kelaidonis K, Sarasia EM, Toth I (2021) A global review on short peptides: frontiers and perspectives. Molecules 26:430
Dang T, Sussmuth RD (2017) Bioactive peptide natural products as lead structures for medicinal use. Acc Chem Res 50:1566–1576
Hemmerling F, Piel J (2022) Strategies to access biosynthetic novelty in bacterial genomes for drug discovery. Nat Rev Drug Discov 5:359–378
Nicolaou KC, Snyder SA (2005) Chasing molecules that were never there: misassigned natural products and the role of chemical synthesis in modern structure elucidation. Angew Chem Int Ed 44:1012–1044
Takada K, Ninomiya A, Naruse M, Sun Y, Miyazaki M, Nogi Y, Okada S, Matsunaga S (2013) Surugamides A–E, cyclic octapeptides with four d-Amino acid residues, from a marine Streptomyces sp.: LC–MS-aided inspection of partial hydrolysates for the distinction of d- and l-amino acid residues in the sequence. J Org Chem 78:6746–6750
Ninomiya A, Katsuyama Y, Kuranaga T, Miyazaki M, Nogi Y, Okada S, Wakimoto T, Ohnishi Y, Matsunaga S, Takada K (2016) Biosynthetic gene cluster for surugamide A encompasses an unrelated decapeptide, surugamide F. ChemBioChem 17:1709–1712
Du L, Lou L (2010) PKS and NRPS release mechanisms. Nat Prod Rep 27:255–278
Kuranaga T, Matsuda K, Sano A, Kobayashi M, Ninomiya A, Takada K, Matsunaga S, Wakimoto T (2018) Total synthesis of the non-ribosomal peptide surugamide B and identification of a new offloading cyclase family. Angew Chem Int Ed 57:9447–9451
White CJ, Yudin AK (2011) Contemporary strategies for peptide macrocyclization. Nat Chem 3:509–524
Albericio F, El-Faham A (2018) Choosing the right coupling reagent for peptides: a 25-year journey. Org Process Res Dev 22:760–772
Kuranaga T, Enomoto A, Tan H, Fujita K, Wakimoto T (2017) Total synthesis of theonellapeptolide Id. Org Lett 19:1366–1369
Yan H, Chen F-E (2022) Recent progress in solid-phase total synthesis of naturally occurring small peptides. Adv Synth Catal 364:1–29
Subirós-Funosas R, Prohens R, Barbas R, El-Faham A, Albericio F (2009) Oxyma: an efficient additive for peptide synthesis to replace the benzotriazole-based HOBt and HOAt with a lower risk of explosion. Chem Eur J 15:9394–9403
Coste J, Le-Nguyen D, Castro B (1990) PyBOP®: a new peptide coupling reagent devoid of toxic by-product. Tetrahedron Lett 31:205–208
Carpino LA (1993) 1-Hydroxy-7-azabenzotriazole. An efficient peptide coupling additive. J Am Chem Soc 115:4397–4398
You YO, Khosla C, Cane DE (2013) Stereochemistry of reductions catalyzed by methyl-epimerizing ketoreductase domains of polyketide synthases. J Am Chem Soc 135:7406–7409
Backes BJ, Ellman JA (1996) An alkanesulfonamide “safety-catch” linker for solid-phase synthesis. J Org Chem 64:2322–2330
Pratt RF (2008) Substrate specificity of bacterial DD-peptidases (penicillin-binding proteins). Cell Mol Life Sci 65:2138–2155
Matsuda K, Kobayashi M, Kuranaga T, Takada K, Matsunaga S, Ikeda H, Wakimoto T (2019) SurE is a trans-acting thioesterase cyclizing two distinct non-ribosomal peptides. Org Biomol Chem 17:1058–1061
Matsuda K, Zhai R, Mori T, Kobayashi M, Sano A, Abe I, Wakimoto T (2020) Heterochiral coupling in non-ribosomal peptide macrolactamization. Nat Catal 3:507–515
Magarvey NA, Haltli B, He M, Greenstein M, Hucul JA (2006) Biosynthetic pathway for mannopeptimycins, lipoglycopeptide antibiotics active against drug-resistant gram-positive pathogens. Antimicrob Agents Chemother 50:2167–2177
Li Q, Song Y, Qin X, Zhang X, Sun A, Ju J (2015) Identification of the biosynthetic gene cluster for the anti-infective desotamides and production of a new analogue in a heterologous host. J Nat Prod 78:944–948
Son S, Hong YS, Jang M, Heo KT, Lee B, Jang JP, Kim JW, Ryoo IJ, Kim WG, Ko SK, Kim BY, Jang JH, Ahn JS (2017) Genomics-driven discovery of chlorinated cyclic hexapeptides ulleungmycins A and B from a Streptomyces species. J Nat Prod 80:3025–3031
Mudalungu CM, Von Törne WJ, Voigt K, Rückert C, Schmitz S, Sekurova ON, Zotchev SB, Süssmuth RD (2019) Noursamycins, chlorinated cyclohexapeptides identified from molecular networking of Streptomyces noursei NTR-SR4. J Nat Prod 82:1478–1486
Zhou X, Huang H, Li J, Song Y, Jiang R, Liu J, Zhang S, Hua Y, Ju J (2014) New anti-infective cycloheptadepsipeptide congeners and absolute stereochemistry from the deep sea-derived Streptomyces drozdowiczii SCSIO 10141. Tetrahedron 70:7795–7801
Li Q, Qin X, Liu J, Gui C, Wang B, Li J, Ju J (2016) Deciphering the biosynthetic origin of l-allo-isoleucine. J Am Chem Soc 138:408–415
Matsuda K, Kuranaga T, Sano A, Ninomiya A, Takada K, Wakimoto T (2019) The revised structure of the cyclic octapeptide surugamide A. Chem Pharm Bull 67:476–480
Nimura N, Toyama A, Kinoshita T (1984) Optical resolution of amino acid enantiomers by high-performance liquid chromatography. J Chromatogr A 316:547–552
Hess S, Gustafson KR, Milanowski DJ, Alvira E, Lipton MA, Pannell LK (2004) Chirality determination of unusual amino acids using precolumn derivatization and liquid chromatography–electrospray ionization mass spectrometry. J Chromatogr A 1035:211–219
Dunbar KL, Scharf DH, Litomska A, Hertweck C (2017) Enzymatic carbon–sulfur bond formation in natural product biosynthesis. Chem Rev 117:5521–5577
Wang N, Saidhareddy P, Jiang X (2020) Construction of sulfur-containing moieties in the total synthesis of natural products. Nat Prod Rep 37:246–275
Schwalen CJ, Hudson GA, Kille B, Mitchell DA (2018) Bioinformatic expansion and discovery of thiopeptide antibiotics. J Am Chem Soc 140:9494–9501
Pan C, Kuranaga T, Liu C, Lu S, Shinzato N, Kakeya H (2020) Thioamycolamides A–E, sulfur-containing cycliclipopeptides produced by the rare actinomycete Amycolatopsis sp. Org Lett 22:3014–3017
Liu H, Liu Y, Wang Z, Xing X, Maguire AR, Luesch H, Ye T (2013) Total synthesis and biological evaluation of grassypeptolide A. Chem Eur J 19:6774–6784
Wipf P, Fritch PC (1996) Total synthesis and assignment of configuration of lissoclinamide 7. J Am Chem Soc 118:12358–12367
Yamashita T, Kuranaga T, Inoue M (2015) Solid-phase total synthesis of bogorol A: stereocontrolled construction of thermodynamically unfavored (E)-2-amino-2-butenamide. Org Lett 17:2170–2173
Pan C, Kuranaga T, Kakeya H (2020) Total synthesis of thioamycolamide A via a biomimetic route. Org Biomol Chem 18:8366–8370
Boden CDJ, Pattenden G, Ye T (1995) The synthesis of optically active thiazoline and thiazole derived peptides from N-protected α-amino acids. Synlett 1995:417–419
Konigsberg W, Hill RH, Craig LC (1961) The oxidation and acid isomerization of bacitracin A. J Org Chem 26:3867–3871
Hirotsu Y, Shiba T, Kaneko T (1970) Synthetic studies on bacitracin. VII. Isomerization of amino acid components of thiazoline peptides. Bull Chem Soc Jpn 43:1870–1873
Yonetani K, Hirotsu Y, Shiba T (1975) Racemization of amino acid residues fused in thiazoline, oxazoline, and imidazoline rings. Bull Chem Soc Jpn 48:3302–3305
Wipf P, Fritch PC (1994) Synthesis of peptide thiazolines from β-hydroxythioamides. An investigation of racemization in cyclodehydration protocols. Tetrahedron Lett 35:5397–5400
Thomsen I, Clausen K, Scheibye S, Lawesson S-O (1984) Thiation with 2,4-bis(4-methoxyphenyl)-1,3,2,4-dithiadiphosphetane 2,4-disulfide: N-methylthiopyrrolidone. Org Synth 62:158
Ozturk T, Ertas E, Mert O (2007) Use of Lawesson’s reagent in organic syntheses. Chem Rev 107:5210–5278
Sakaitani M, Ohfune Y (1990) Syntheses and reactions of silyl carbamates. 1. Chemoselective transformation of amino protecting groups via tert-butyldimethylsilyl carbamates. J Org Chem 55:870–876
Burkhart JL, Kazmaier U (2011) A straightforward approach to protected (S)-dolaphenine (Doe), the unusual amino acid component of dolastatin 10. Synthesis 2011:4033–4036
Yamashita T, Matoba H, Kuranaga T, Inoue M (2014) Total syntheses of nobilamides B and D: application of traceless Staudinger ligation. Tetrahedron 70:7746–7752
Nicolaou KC, Estrada AA, Zak M, Lee SH, Safina BS (2005) A mild and selective method for the hydrolysis of esters with trimethyltin hydroxide. Angew Chem Int Ed 44:1378–1382
Burns JA, Butler JC, Moran J, Whitesides GM (1991) Selective reduction of disulfides by tris(2-carboxyethyl)phosphine. J Org Chem 56:2648–2650
Zou B, Long K, Ma D (2005) Total synthesis and cytotoxicity studies of a cyclic depsipeptide with proposed structure of palau’amide. Org Lett 7:4237–4240
Sugiyama H, Watanabe A, Teruya T, Suenaga K (2009) Synthesis of palau’amide and its diastereomers: confirmation of its stereostructure. Tetrahedron Lett 50:7343–7345
Ma B, Litvinov DN, He L, Banerjee B, Castle SL (2009) Total synthesis of celogentin C. Angew Chem Int Ed 48:6104–6107
Ma B, Banerjee B, Litvinov DN, He L, Castle SL (2010) Total synthesis of the antimitotic bicyclic peptide celogentin C. J Am Chem Soc 132:1159–1171
Kuranaga T, Minote M, Morimoto R, Pan C, Ogawa H, Kakeya H (2020) Highly sensitive labeling reagents for scarce natural products. ACS Chem Biol 15:2499–2506
Kuranaga T, Sesoko Y, Sakata K, Maeda N, Hayata A, Inoue M (2013) Total synthesis and complete structural assignment of yaku’amide A. J Am Chem Soc 135:5467–5474
Kuranaga T, Mutoh H, Sesoko Y, Goto T, Matsunaga S, Inoue M (2015) Elucidation and total synthesis of the correct structures of tridecapeptides yaku’amides A and B. Synthesis-driven stereochemical reassignment of the four amino acid residues. J Am Chem Soc 137:9443–9451
Jiang Y, Matsumoto T, Kuranaga T, Lu S, Wang W, Onaka H, Kakeya H (2021) Longicatenamides A–D, two diastereomeric pairs of antimicrobials cyclic hexapeptides produced by combined-culture of Streptomyces sp. KUSC_F05 and Tsukamurella pulmonis TP-B0596. J Antibiot 74:307–316
Pan C, Kuranaga T, Cao X, Suzuki T, Dohmae N, Shinzato N, Onaka H, Kakeya H (2021) Amycolapeptins A and B, cyclic nonadepsipeptides produced by combined-culture of Amycolatopsis sp. and Tsukamurella pulmonis TP-B0596. J Org Chem 86:1843–1849
Kuranaga T, Tamura M, Ikeda H, Terada S, Nakagawa Y, Kakeya H (2021) Identification and total synthesis of an unstable anticancer macrolide presaccharothriolide Z. Org Lett 23:7106–7111
Pan C, Kuranaga T, Kakeya H (2021) Application of the highly sensitive labeling reagent to the structural confirmation of readily isomerizable peptides. J Nat Med 75:339–343
Vagstad AL, Kuranaga T, Püntener S, Pattabiraman V, Bode JW, Piel J (2019) Introduction of d-amino acids in minimalistic peptide substrates by an S-adenosyl-l-methionine radical epimerase. Angew Chem Int Ed 58:2246–2250
Shirai T, Kuranaga T, Wright JLC, Baden DG, Satake M, Tachibana K (2010) Synthesis of a proposed biosynthetic intermediate of a marine cyclic ether brevisamide for study on biosynthesis of marine ladder-frame polyethers. Tetrahedron Lett 51:1394–1396
Acknowledgements
The author acknowledges Prof. Toshiyuki Wakimoto (Hokkaido University) and Prof. Hideaki Kakeya (Kyoto University) for their helpful support on this work. This work was financially supported in part by a Grant-in-Aid for Scientific Research from the Ministry of Education, Culture, Sports, Science and Technology, Japan (JSPS KAKENHI 22K05112), the SUNBOR GRANT, the Daiichi-Sankyo Award in The Society of Synthetic Organic Chemistry Japan, the Takeda Science Foundation, The Tokyo Biochemical Research Foundation, and the foundation of Tokyo Chemical Industry.
Author information
Authors and Affiliations
Corresponding author
Additional information
Publisher's Note
Springer Nature remains neutral with regard to jurisdictional claims in published maps and institutional affiliations.
Rights and permissions
Open Access This article is licensed under a Creative Commons Attribution 4.0 International License, which permits use, sharing, adaptation, distribution and reproduction in any medium or format, as long as you give appropriate credit to the original author(s) and the source, provide a link to the Creative Commons licence, and indicate if changes were made. The images or other third party material in this article are included in the article's Creative Commons licence, unless indicated otherwise in a credit line to the material. If material is not included in the article's Creative Commons licence and your intended use is not permitted by statutory regulation or exceeds the permitted use, you will need to obtain permission directly from the copyright holder. To view a copy of this licence, visit http://creativecommons.org/licenses/by/4.0/.
About this article
Cite this article
Kuranaga, T. Total syntheses of surugamides and thioamycolamides toward understanding their biosynthesis. J Nat Med 77, 1–11 (2023). https://doi.org/10.1007/s11418-022-01662-x
Received:
Accepted:
Published:
Issue Date:
DOI: https://doi.org/10.1007/s11418-022-01662-x