Abstract
In order to simulate the premixed turbulent flame and to study the thermodynamical and chemical state in the post flame region we integrate a number of appropriate submodels in a complete model. The flame front position is described as an iso-surface of a field variable G, and chemistry and turbulence-chemistry interaction models are conditioned on the flame front position following the conditioned progress variable approach (CPVA). Three special features of the model have been addressed to clarify following questions: (1) Is it really necessary to apply the conditioned progress variable approach, or is the ILDM based on the multivariate presumed PDF alone able to predict the flame behavior with reasonable accuracy? (2) Is the CPVA based on ILDM notedly advantageous compared to that based on the chemistry equilibrium? (3) How noticeable is the influence of some model parameters for the description of the flame brush thickness on the prediction of species concentration in the postflame region? For this purpose, some simulation results are presented and compared with experimental data of a turbulent premixed flame, the so-called “F2” case experimentally investigated by Chen et al. [Combust. Flame 107 (1996) 223–224]. Comparison results with different model combinations along with equilibrium chemistry consideration reveal that the CPVA based on ILDM achieved better agreement with experimental data provided suitable value for the model parameter is used.
Similar content being viewed by others
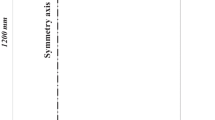
References
Peters, N., Turbulent Combustion. Cambridge University Press, Cambridge (2000).
Oberlack, M., Wenzel, H. and Peters, N., On symmetries, invariant solutions and averaging of the G-equation in premixed combustion. Combust. Theor. Model. 5 (2001) 363–383.
Bray, K.N.C., Libby, P.A. and Moss, J.B., Combust. Flame 61 (1985) 87–102.
Veynante, D. and Vervisch, L., Turbulent combustion modeling. Proc. Energy Combust. Sci. 28(3) (2002) 193–301.
Chen, Y.C., Peters, N., Schneemann, G.A., Wruck, N., Renz, U. and Mansour, M.S., Combust. Flame 107 (1996) 223–224.
Veynante, D., Piana, J., Duklos, J.M. and Martel, C., Experimental analysis of flame surface density model for premixed turbulent combustion. In: Twenty-Sixth Symposium (International) on Combustion, The Combustion Institute (1996) pp. 413–420.
Bailly, Garreton, D., Simonin, O., Bruel, P., Champion, M., Deshaies, B., Duplantier, S. and Sanquer, S., Experimental and numerical study of premixed flame stabilized by a rectangular section cylinder. In: Twenty Sixth Symposium (International) on Combustion, The Combustion Institute (1996) pp. 923–930.
Piana, J., Veynante, D., Candel, S. and Poinsot, T., Direct numerical simulation analysis of the G-equation in premixed combustion. In: Chollet, J.P., Voke, P.R. and Kleiser, L. (eds.), Direct and Large Eddy Simulation II, Kluwer Academic Publishers (1997) pp. 321–330.
Borger, M., Veynante, D., Boughanem, H. and Trouve, A., Direct Numerical Simulation analysis of the flame surface density concept for Large Eddy Simulation of turbulent premixed combustion. In: Twenty-Seventh Symposium (International) on Combustion, The Combustion Institute (1998) pp. 917–925.
Wenzel, H., Direkte Numerische Simulation der Ausbreitung einer Flammenfront in einem homogenen Turbulenzfeld. Ph.D. Thesis, Aachen (2000).
Vervisch, P., Domingo, R. and Haugel, Turbulent combustion in the light of direct and large eddy simulations. J. Turbul. 5(1) (2004) 4–4(1).
Pitsch, H. and Duchamp de Lageneste, L., Large-Eddy simulation of premixed turbulent combustion using a level-set approach. Proc. Combust. Inst. 29 (2002) 2001–2008.
Hawkes, E.R. and Cant, S.R., A flame surface density approach to large eddy simulation of premixed turbulent combustion. Proc. Combust. Inst. 28 (2000) 51–58.
Angelberger, C., Veynante, D., Egolfopoulos, F. and Poinsot, T., Large eddy simulation of combustion instabilities in turbulent premixed flames. In: Proceedings of the Summmer Program Center for Turbulence Research (1998) pp. 61–82.
Colin, O., Ducros, F., Veynante, D. and Poinsot, T., A thickened flame model for large-eddy simulation of turbulent premixed combustion. Phys. Fluids A 12(7) (2000) 1843–1863.
Duessing, K.M., Large-Eddy Simulations Turbulenter Vormischflammen. Ph.D. Thesis, TU-Darmstadt (2004).
Janicka, J. and Sadiki, A., Large eddy simulation of turbulent combustion systems. Pro. Combust. Inst. 30 (2005) 537–547.
Bray, K.N.C., Libby, P.A. and Moss, J.B., Unified modeling approach for premixed turbulent combustion. Part I: General formulation. Combust. Flame 61 (1985) 87–102.
Lindstedt, R.P. and Vaos, E.M., Modeling of premixed turbulent flames with second moment methods. Combust. Flame 116 (1999) 461–485.
Veynante, D., Trouve, A., Bray, K.N.C. and Mantel, T., Gradient and counter-gradient scalar transport in turbulent premixed flames. J. Fluid Mech. 332 (1997) 263–293.
Hermann, M., Numerical simulation of premixed turbulent combustion based on level-set flamelet model. Dissertation, RWTH Aachen (2001).
Nilsson, P. and Bai, X.S., Effects of flame stretch and wrinkling on CO formation in turbulent premixed combustion. In: Twenty-Ninth Symposium (International) on Combustion, Vol. 29 (2002) pp. 1873–1879.
Engdar, A., Klingmann, J. and Nilsson, P., Investigation of turbulence models applied to premixed combustion using a level-set flamelet library approach. In: ASME 2003, GT2003-38331.
Repp, S., Khvissiouk, E., Sadiki, A. and Janicka, J., Modeling and simulation of a turbulent premixed methane/air flame based on the G-equation. In: Proceedings of ASME Turbo Expo 2002, Amsterdam, Netherlands (2002).
Maltsev, A., Sadiki, A. and Janicka, J., A new BML-based modeling for the description of gas turbine typical combustion process. Prog. Comput. Fluid Dyn. (2004) (in press).
Maltsev, A., Sadiki, A. and Janicka, J., Coupling of extended BML model and advanced turbulence and mixing models in predicting partially premixed flames. In: Third International Symposium on Turbulence and Shear Flow Phenomena, TSFP3, Sendai, Japan, June 25–27, 2003, vol. 1, pp. 4–4(1).
Landenfeld, T., Sadiki, A. and Janicka, J., A turbulence-chemistry interaction model based on a multivariate presumed β-PDF method for turbulent flames. Flow Turbul. Combust. 68 (2002) 111–135.
Sussman, M., Smereka, P. and Osher, S., A level set approach for computing solution to incompressible two-phase flow. J. Comp. Phys. 114 (1994) 146–159.
Nilsson, P. and Bai, X.S., Level-set flamelet library approach for premixed turbulent combustion. Exp. Therm. Fluid. Sci. 21 (2000) 87–98.
Landenfeld, T., Sadiki, A. and Janicka, J., A turbulence-chemistry interaction model based on a multivariate presumed β-PDF method for turbulent flames. Flow Turbul. Combust. 68 (2002) 111–135.
Schmidt, D., Segatz, J., Riedel, U., Warnatz, J. and Maas, U., Simulation of laminar methane-air flame using automatically simplified chemical kinetics. Combust. Sci. Tech. 113–114 (1996) 3–16.
Launder, B.E. and Spalding, D.B., Mathematical Models of Turbulence. Academic Press, London, NY (1972).
Maltsev, A., Towards the Development and Assessment of Complete CFD Models for the Silulation of Stationary Gas Turbine Combustion Processes. Dissertation, TU-Darmstadt (2003).
Russo, G. and Smereka, P., A remark on computing distance function. Phys. Fluids 12(1).
Maas, U. and Pope, S.B., Simplifying chemical kinetics: Intrinsic low-dimensional manifolds in composition space. Combust. Flame 88 (1992) 239–264.
Janicka, J., Habilitationsschrift, RWTH Aachen (1984).
Durst, F. and Schäfer, M., A parallel blockstructured multigrid method for the prediction of incompressible flow. J. Numer. Meth. Fluids 22 (1996) 549–565.
Chen, M., Hermann, M. and Peters, N., Proc. Combust. Inst. 26 (2000) 167–174.
Repp, S., Hinz, A., Landenfeld, T., Schneider, C., Sadiki, A. and Janicka, J., Prediction of swirling confined diffusion flame with a Monte Carlo and a presumed PDF-model. Int. J. Heat Mass Transf. 45 (2002) 1271–1285.
Janicka, J. and Kollmann, W., The calculation of mean radical concentrations in turbulent diffusion flames. Combust. Flame 44 (1982) 319–336.
Girimaji, S.S., Assumed β-pdf model for turbulent mixing: Validation and extension to multiple scalar mixing. Combust. Sci. Technol. 78 (1991) 177–196.
Knikker, R., Veynante, D. and Meneveau, C., A priori testing of a similarity model for large eddy simulations of turbulent premixed combustion. Proc. Combust. Inst. 29 (2000) 2105–2111.
Nottin, C., Knikker, R., Borger, M. and Veynante, D., Large eddy simulations of an acoustically excited turbulent premixed flame. Proc. Combust. Inst. 28 (2000) 67–73.
Zimont, V.L., Biagioli, F. and Syed, K., Modeling turbulent combustion in the intermediate steady propagation regime. Prog. Comput. Fluid Dyn. 1(1/2/3) (2001) 14–28.
Peters, N., Laminar flame concept in turbulent combustion. In: Twenty-First Symposium (International) on Combustion, The Combustion Institute (1986) pp. 1231–1250.
Author information
Authors and Affiliations
Corresponding author
Rights and permissions
About this article
Cite this article
Schneider, E., Sadiki, A. & Janicka, J. Modeling and 3D-Simulation of the Kinetic Effects in the Post-Flame Region of Turbulent Premixed Flames Based on the G-Equation Approach. Flow Turbulence Combust 75, 191–216 (2005). https://doi.org/10.1007/s10494-005-8588-z
Received:
Accepted:
Issue Date:
DOI: https://doi.org/10.1007/s10494-005-8588-z