Abstract
To remove atmospheric pressure loading (ATML) effect from GNSS coordinate time series, surface pressure (SP) models are required to predict the displacements. In this paper, we modeled the 3D ATML surface displacements using the latest MERRA-2 SP grids, together with four other products (NCEP-R-1, NCEP-R-2, ERA-Interim and MERRA) for 596 globally distributed GNSS stations, and compared them with ITRF2014 residual time series. The five sets of ATML displacements are highly consistent with each other, particularly for those stations far away from coasts, of which the lowest correlations in the Up component for all the four models w.r.t MERRA-2 become larger than 0.91. ERA-Interim-derived ATML displacement performs best in reducing scatter of the GNSS height for 90.3% of the stations (89.3% for NCEP-R-1, 89.1% for NCEP-R-2, 86.4% for MERRA and 85.1% for MERRA-2). We think that this may be possibly due to the 4D variational data assimilation method applied. Considering inland stations only, more than 96% exhibit WRMS reduction in the Up direction for all five models, with an average improvement of 3–4% compared with the original ITRF2014 residual time series before ATML correction. Most stations (> 67%) also exhibit horizontal WRMS reductions based on the five models, but of small magnitudes, with most improvements (> 76%) less than 5%. In particular, most stations in South America, South Africa, Oceania and the Southern Oceans show larger WRMS reductions with MERRA-2, while all other four SP datasets lead to larger WRMS reduction for the Up component than MERRA-2 in Europe. Through comparison of the daily pressure variation from the five SP models, we conclude that the bigger model differences in the SP-induced surface displacements and their impacts on the ITRF2014 residuals for coastal/island stations are mainly due to the IB correction based on the different land–sea masks. A unique high spatial resolution land–sea mask should be applied in the future, so that model differences would come from only SP grids. Further research is also required to compare the ATML effect in ice-covered and high mountainous regions, for example the Qinghai–Tibet Plateau in China, the Andes in South America, etc., where larger pressure differences between models tend to occur.













Similar content being viewed by others
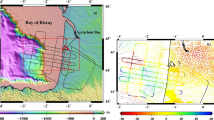
Data availability statement
The ATML time series involved in the research and the scripts to generate the integer land–sea masks for MERRA and MERRA-2 are available upon request.
Notes
References
Altamimi Z, Rebischung P, Métivier L, Collilieux X (2016) ITRF2014: a new release of the international terrestrial reference frame modeling nonlinear station motions. J Geophys Res 121(8):6109–6131. https://doi.org/10.1002/2016JB013098
Berrisford P, Dee D, Poli P, Brugge R, Fielding K, Fuentes M, Kallberg P, Kobayashi S, Uppala S, Simmons A (2011) ERA report series: the ERA-Interim archive Version 2.0. European Centre for Medium Range Weather Forecasts, Shinfield Park, pp 1–27
Blewitt G (2003) Self-consistency in reference frames, geocenter definition, and surface loading of the solid Earth. J Geophys Res. https://doi.org/10.1029/2002JB002082
Bosilovich MG, Lucchesi R, Suarez M (2016) MERRA-2: file specification. GMAO Office Note No. 9 (Version 1.1). https://gmao.gsfc.nasa.gov/pubs/office_notes
Brondeel M, Willems T (2003) Atmospheric pressure loading in GPS height estimates. Adv Space Res 31(8):1959–1964. https://doi.org/10.1016/S0273-1177(03)00157-1
Dach R, Böhm J, Lutz S, Steigenberger P, Beutler G (2011) Evaluation of the impact of atmospheric pressure loading modeling on GNSS data analysis. J Geod 85:75–91. https://doi.org/10.1007/s00190-010-0417-z
Dee DP, Uppala SM, Simmons AJ, Berrisford P, Poli P, Kobayashi S, Andrae U, Balmaseda MA, Balsamo G, Bauer P, Bechtold P, Beljaars ACM, van de Berg L, Bidlot J, Bormann N, Delsol C, Dragani R, Fuentes M, Geer AJ, Haimberger L, Healy SB, Hersbach H, Hólm EV, Isaksen L, Kållberg P, Köhler M, Matricardi M, McNally AP, Monge-Sanz BM, Morcrette JJ, Park BK, Peubey C, de Rosnay P, Tavolato C, Thépaut JN, Vitart F (2011) The ERA-Interim reanalysis: configuration and performance of the data assimilation system. Q J R Meteorol Soc 137:553–597. https://doi.org/10.1002/qj.828
Deng LS, Jiang WP, Li Z, Chen H, Wang KH, Ma YF (2017) Assessment of second- and third-order ionospheric effects on regional networks: case study in China with longer CMONOC GPS coordinate time series. J Geod 91:207–227. https://doi.org/10.1007/s00190-016-0957-y
Dong D, Dickey JO, Chao Y, Cheng MK (1997) Geocenter variations caused by atmosphere, ocean and surface ground water. Geophys Res Lett 24(15):1867–1870
Dong D, Yunck T, Heflin M (2002JB) Origin of the international terrestrial reference frame. J Geophys Res. https://doi.org/10.1029/2002JB002035
Farrell WE (1972) Deformation of the earth by surface loads. Rev Geophys Space Phys 10(3):751–797
Fritsche M, Döll P, Dietrich R (2012) Global-scale validation of model-based load deformation of the Earth’s crust from continental water mass and atmospheric pressure variations using GPS. J Geodyn 59–60:133–142
Griffiths J (2019) Combined orbits and clocks from IGS second reprocessing. J Geod 93:177–195. https://doi.org/10.1007/s00190-018-1149-8
Jiang WP, Li Z, van Dam T, Ding WW (2013) Comparative analysis of different environmental loading methods and their impacts on the GPS height time series. J Geod 87(7):687–703. https://doi.org/10.1007/s00190-013-0642-3
Johnston G, Riddell A, Hausler G (2017) The International GNSS Service. In: Teunissen PJG, Montenbruck O (eds) Springer Handbook of Global Navigation Satellite Systems, 1st edn. Springer International Publishing, Cham, pp 967–982. https://doi.org/10.1007/978-3-319-42928-1
Kalnay E, Kanamitsu M, Kistler R, Collins W, Deaven D, Gandin L, Iredell M, Saha S, White G, Woollen J, Zhu Y, Chelliah M, Ebisuzaki W, Higgins W, Janowiak J, Mo K, Ropelewski C, Wang J, Leetmaa A, Reynolds R, Jenne R, Joseph D (1996) The NCEP/NCAR 40-year reanalysis project. Bull Am Meteorol Soc 77(3):437–471. https://doi.org/10.1175/1520-0477
Kanamitsu M, Ebisuzaki W, Woollen J, Sk Y, Hnilo JJ, Fiorino M, Potter GL (2002) NCEP-DOEAMIP-II reanalysis (R-2). Bull Am Meteorol Soc 83(83):1631–1643. https://doi.org/10.1175/BAMS-83-11-1631
King MA, Watson CS (2010) Long GPS coordinate time series: multipath and geometry effects. J Geophys Res. https://doi.org/10.1029/2009JB006543
Li Z (2012) Research on the non-linear variation of GPS coordinate time series. Ph.D. dissertation. Wuhan University
Li Z, Jiang WP, Ding WW, Deng LS, Peng LF (2014) Estimates of minor ocean tide loading displacement and its impact on continuous GPS coordinate time series. Sensors 14(3):5552–5572. https://doi.org/10.3390/s140305552
Li Z, van Dam T, Collilieux X, Altamimi Z, Rebischung P, Nahmani S (2015) Quality evaluation of the weekly vertical loading effects induced from continental water storage models. In: IAG 150 years, International Association of Geodesy Symposia, vol 143, Springer International Publishing, Switzerland, pp 45–54. https://doi.org/10.1007/1345_2015_174
Li Z, van Dam T (2015) The phase 2 North America land data assimilation system (NLDAS-2) products for modeling water storage displacements for plate boundary observatory GPS stations. In: International Association of Geodesy Symposia, Springer International Publishing, Switzerland, pp 1–9. https://doi.org/10.1007/1345_2015_176
Li Z, Chen W, Jiang WP, Deng LS, Yang RH (2018) The magnitude of diurnal/semidiurnal atmospheric tides (S1/S2) and their impacts on the continuous GPS coordinate time series. Remote Sens 10:1125. https://doi.org/10.3390/rs10071125
Lindsay R, Wensnahan M, Schweiger A, Zhang J (2014) Evaluation of seven different atmospheric reanalysis products in the arctic. J Climate 27:2588–2606. https://doi.org/10.1175/JCLI-D-13-00014.1
Lucchesi, R. (2012). File specification for MERRA products. GMAO Office Note No. 1 (Version 2.3). https://gmao.gsfc.nasa.gov/pubs/office_notes. Accessed 25 Sept 2018
Penna NT, Stewart MP (2003) Aliased tidal signatures in continuous GPS height time series. Geophys Res Lett 30(23):2184. https://doi.org/10.1029/2003GL018828
Penna NT, King MA, Stewart MP (2007) GPS height time series: short-period origins of spurious long-period signals. J Geophys Res. https://doi.org/10.1029/2005JB004047
Ray J, Altamimi Z, Collilieux X, van Dam T (2008) Anomalous harmonics in the spectra of GPS position estimates. GPS Solut 12:55–64
Ray J, Griffiths J, Collilieux X, Rebischung P (2013) Subseasonal GNSS positioning errors. Geophys Res Lett 40(22):5854–5860. https://doi.org/10.1002/2013GL058160
Rienecker MM, Suarez MJ, Gelaro R, Todling R, Bacmeister J, Liu E, Bosilovich MG, Schubert SD, Takacs L, Kim GK, Bloom S, Chen JY, Collins D, Conaty A, da Silva A, Gu W, Joiner J, Koster RD, Lucchesi R, Molod A, Owens T, Pawson S, Pegion P, Redder CR, Reichle R, Robertson FR, Ruddick AG, Sienkiewicz M, Woollen J (2011) MERRA-NASA's modern-era retrospective analysis for research and applications. J Clim 24(14):3624–3648. https://doi.org/10.1175/JCLI-D-11-00015.1
Rebischung P, AltamimiZ RJ, Garayt B (2016) The IGS contribution to ITRF2014. J Geod 90(7):611–630. https://doi.org/10.1007/s00190-016-0897-6
Salstein DA, Ponte RM, Cady-Pereira K (2008) Uncertainties in atmospheric surface pressure fields from global analyses. J Geophys Res. https://doi.org/10.1029/2007JD009531
Steigenberger P, Rothacher M, Schmid R, Rülke A, Fritsche M, Dietrich R, Tesmer V (2009) Effects of different antenna phase center models on GPS-derived reference frame. In: Geodetic reference frames. International Association of Geodesy Symposia, vol 134, pp 83–88
Tregoning P, van Dam T (2005) Atmospheric pressure loading corrections applied to GPS data at the observation level. Geophys Res Lett. https://doi.org/10.1029/2005GL024104
Tregoning P, Watson C (2009JB) Atmospheric effects and spurious signals in GPS analyses. J Geophys Res 114:B09403. https://doi.org/10.1029/2009JB006344
Tregoning P, Watson C, Ramillien G, McQueen H, Zhang J (2009) Detecting hydrologic deformation using GRACE and GPS. Geophys Res Lett. https://doi.org/10.1029/2009GL038718
van Dam TM, Wahr J (1987) Displacements of the Earth's surface due to atmospheric loading: Effects on gravity and baseline measurements. J Geophys Res 92:1281–1286
van Dam T, Blewitt G, Heflin M (1994) Atmospheric pressure loading effects on global positioning system coordinate determinations. J Geophys Res 99(B12):23939–23950
van Dam TM, Wahr J, Chao Y, Leuliette E (1997) Predictions of crustal deformation and of geoid and sea-level variability caused by oceanic and atmospheric loading. Geophys J Int 129(3):507–517
van Dam T, Wahr J, Milly PCD, Shmakin AB, Blewitt G, Lavallée D, Larson KM (2001) Crustal displacements due to continental water loading. Geophys Res Lett 28(4):651–654
van Dam T, Altamimi Z, Collilieux X, Ray J (2010) Topographically induced height errors in predicted atmospheric loading effects. J Geophys Res. https://doi.org/10.1029/2009JB006810
van Dam T, Collilieux X, Wuite J, Altamimi Z, Ray J (2012) Nontidal ocean loading: amplitudes and potential effects in GPS height time series. J Geod 86(11):1043–1057. https://doi.org/10.1007/s00190-012-0564-5
Wessel P, Smith WHF (2018) The generic mapping tools. Version 4.5.18 technical reference and cookbook. Laboratory for Satellite Altimetry, NOAA/NESDIS/STAR
Wijaya DD, Böhm J, Karbon M, Krásná H, Schuh H (2013) Atmospheric pressure loading. In: Böhm J, Schuh H (eds) Atmospheric effects in space geodesy. Springer, Berlin, pp 137–157. https://doi.org/10.1007/978-3-642-36932-2_4
Williams SDP, Penna NT (2011) Non-tidal ocean loading effects on geodetic GPS heights. Geophys Res Lett. https://doi.org/10.1029/2011GL046940
Yuan P, Li Z, Jiang WP, Ma YF, Chen W, Sneeuw N (2018) Influences of environmental loading corrections on the nonlinear variations and velocity uncertainties for the reprocessed global positioning system height time series of the crustal movement observation network of China. Remote Sens 10(6):958. https://doi.org/10.3390/rs10060958
Acknowledgements
We thank Mike Bosilovich for helping us generate the MERRA integer land–sea mask. We thank the IGN for providing us the latest ITRF2014 residuals. We also thank Dr. Xavier Collilieux for helping us improve our paper. Figures in this paper are plotted with the GMT and MATLAB software. This research is supported by the National Key Research and Development Program of China (Project 2016YFB0502101), the European Commission/Research Grants Council (RGC) Collaboration Scheme sponsored by the Research Grants Council of Hong Kong Special Administrative Region, China (Project No. E-PolyU 501/16), and the National Science Foundation for Distinguished Young Scholars of China (Grant No. 41525014).
Author information
Authors and Affiliations
Contributions
ZL designed and performed the research; PR and ZA provided the GPS data; TVD provided the software; ZL analyzed the data and wrote the manuscript; WC, TVD and PR revised the manuscript.
Corresponding author
Electronic supplementary material
Below is the link to the electronic supplementary material.
Appendix
Appendix
To help users deal with the non-integer constants files, here we give a simple step of how to generate integer land/sea mask for MERRA and MERRA-2 datasets:
- 1.
Download the MERRA constant file with short name as MAC0NXASM.
- 2.
Create a local binary file that has integer masks for land and ocean in MERRA using gradsdap script. Then, generate a descriptor file that can be used to open the above binary integer mask file in GrADS.
- 3.
Write the land and sea variables from the above descriptor file into separate files in netcdf format using GrADS command sdfwrite.Footnote 14 Note that for using the command sdfwrite, users should install a fairly recent version of GrADS, e.g., version 2.0.a3 at least.
- 4.
Combine the separate land and sea netcdf files to generate the MERRA integer land/sea mask.
- 5.
Download the MERRA-2 constant file with short name as M2C0NXASM, and repeat the above procedures from (1) to (4) to generate the MERRA-2 integer land–sea mask
After implementing the above five steps, we can use the obtained integer land/sea mask for modeling the ATML effects using both MERRA and MERRA-2 PS grids. When creating the binary file from MERRA and MERRA-2 that containing integer masks for land and ocean, a fractional cutoff value is used to determine that whether a point is classified land or sea. Here, we choose the cutoff value as 0.25 for global study (from Mike Bosilovich’s cookbook). For regional studies where the land and ocean are intend to be discretized, more attempts should be examined to determine an appropriate cutoff value.
Rights and permissions
About this article
Cite this article
Li, Z., Chen, W., van Dam, T. et al. Comparative analysis of different atmospheric surface pressure models and their impacts on daily ITRF2014 GNSS residual time series. J Geod 94, 42 (2020). https://doi.org/10.1007/s00190-020-01370-y
Received:
Accepted:
Published:
DOI: https://doi.org/10.1007/s00190-020-01370-y