Abstract
Shape memory alloys (SMAs) provide an attractive solid-state actuation alternative to engineers in various fields due to their ability to exhibit recoverable deformations while under substantial loads. This feature is of particular importance when utilising the smart composite materials reinforced by SMA. Many constitutive models describing this repeatable phenomenon have been proposed, where some models also capture the effects of rate-independent irrecoverable deformations in SMAs. This paper presents experimental investigations and numerical simulations on shape memory alloys. First, by consisting in determining the transformations of equiatomic Ti–Ni shape memory alloys by differential scanning calorimeter. Then, in order to validate a 3D numerical model of the pseudoelastic behaviour of SMA allowing a finite strain analysis, a set of experimental tests at various initial temperatures is proposed. Finally, the numerical simulations of uniaxial tests performed on shape memory alloys are presented and compared with experimental data, permitting the validation of the proposed modelling. Reasonably good correlation is obtained between the experimental and model predictions.
Similar content being viewed by others
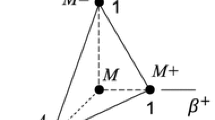
References
Nemat-Nassera S, Sua Y, Guob W-G, Isaacsa J (2005) Experimental characterization and micromechanical modeling of superelastic response of a porous NiTi shape-memory alloy. J Mech Phys Solids 53(2320):2346
Hsu Y-F, Wang WH, Wayman CM (1999) Microstructure and martensitic transformations in a dual-phase α/β Cu-Zn alloy. Metall Mater Trans 30(729):739
Lee E-S, Shin TH (2011) An evaluation of the machinability of nitinol shape memory alloy by electrochemical polishing. J Mech Sci Technol 25(4):963–969
Telmo, Santos G, Braz Fernandes F, Bernardo G, Miranda RM (2013) Analyzing mechanical properties and nondestructive characteristics of brazed joints of NiTi shape memory alloys to carbon steel rods. Int J Adv Manuf Technol 66:787–793
Lei X, Rui W, Yong L (2011) The optimization of annealing and cold-drawing in the manufacture of the Ni-Ti shape memory alloy ultra-thin wire. Int J Adv Manuf Technol 55:905–910
Tak W, Lee M, Kim B (2011) Ultimate load and release time controllable non-explosive separation device using a shape memory alloy actuator. J Mech Sci Technol 25(5):1141–1147
Nam TH, Hur SG, Ahn IS (1998) Phase transformation behaviours of Ti-Ni-Cu shape memory alloy powders fabricated by mechanical alloying. Met Mater Int 4(1):61–66
Brook G-B (1983) Applications of titanium–nickel shape memory alloys. Mater Des 4(835):840
Pan W, Qin Y, Law F, Ma Y, Brockett A, Juster N (2008) Feasibility study and tool design of using shape memory alloy as tool-structural elements for forming-error compensation in microforming. Int J Adv Manuf Technol 38(3-4):393–401
Jee K-K, Hana J-H, Jang WY (2006) A method of pipe joining using shape memory alloys. Mater Sci Eng A 438–440(1110):1112
Santos TG, Fernandes FB, Bernardo G, Miranda RM (2013) Analyzing mechanical properties and non-destructive characteristics of brazed joints of NiTi shape memory alloys to carbon steel rods. Int J Adv Manuf Technol 66(787):793
Petrini L, Migliavacca F, Massarotti P, Schievano S, Dubini G, Auricchio F (2005) Computational studies of shape memory alloy behavior in biomedical applications. J Biomech Eng 127(716):725
Hartl D-J, Lagoudas D-C (2007) Aerospace applications of shape memory alloys. Proc IMechE G J Aerosp Eng 221(535):552
DesRoches R, Smith B (2004) Shape memory alloys in seismic resistant design and retrofit: a critical review of their potential and limitations. J Earthq Eng 8(415):429
Lee JK, Kim GD (2005) A theoretical comparison of two possible shape memory processes in shape memory alloy reinforced metal matrix composite. J Mech Sci Technol 19(7):1460–1468
Leclerq S, Lexcellent C (1996) A general macroscopic description of the thermomechanical behavior of shape memory alloys. J Mech Phys Solids 44(953):980
Wei ZG (1998) Transformation relaxation and aging in a CuZnAl shape-memory alloy studied by modulated differential scanning calorimetry. Metall Mater Trans A 29(11):2697–2705
Chu C-L, Chung C-Y, Lin P-H (2005) Characterization of transformation behaviour in porous Ni-rich NiTi shape memory alloy fabricated by combustion synthesis. J Mater Sci 40(3):773–776
Sepulveda A, Muñoz R, Lovey F-C, Auguet C, Isalgue A, Torra V (2007) Metastable effects on martensitic transformation in SMA (II): the grain growth effects in Cu-Al-Be alloy. J Therm Anal Calorim 89(101):107
Pieczyska E-A, Gadaj S-P, Nowacki W-K, Tobushi H (2006) Martensite and reverse transformation during simple shear of niti shape memory alloy. Exp Mech 46(531):542
Fischer F-D, Berveiller M, Tanaka K, Oberaigner E-R (1994) Continuum mechanical aspects of phase transformations in solids. Arch Appl Mech 64(54):85
Green A-E, Nagdhi P-M (1977) On the thermodynamics and the nature of the second law. Proc Roy Soc London A357(253):270
Ivshin Y, Pence T-J (1994) A thermomechanical model for a one variant shape memory material. J Intell Mater Syst Struct 5(455):473
Lacarbonara W, Bernardini D, Vestroni F (2004) Nonlinear thermomechanical oscillations of shape-memory devices. Int J Solids Struct 41(1209):1234
Mariano P-M (2002) Multifield theories in mechanics of solids. Adv Appl Mech 38(1):93
Otsuka K, Shimizu K (1986) Pseudoelasticity and shape memory effects in alloys. Int Metals Rev 31(93):114
Menezes L-F, Teodosiu C (2000) Three-dimensional numerical simulation of the deepdrawing process using solid finite elements. J Mater Process Technol 97(100):106
Menezes L-F (1994) Modélisation tridimensionnelle et simulation numérique des processus de mise en forme: application a’ l’emboutissage des tôles métalliques. Ph.D. thesis, University of Coimbra–University of Paris 13
Paiva A, Savi M-A, Bragra AMB, Pacheco PMCL (2005) A constitutive model for shape memory alloys considering tensile-compressive asymmetry and plasticity. Int J Solids Struct 42(3439):3457
Yan W, Wang C, Zhang X, Mai Y (2003) Theoretical modelling of the effect of plasticity on reverse transformation in superelastic shape memory alloys. Mater Sci Eng A A354(146):157
Zhang Y, Cheng Y, Grummon D (2007) Finite element modeling of indentation-induced superelastic effect using a three-dimensional constitutive model for shape memory materials with plasticity. J Appl Phys 101(1):6
Wang X, Xu B-X, Yue Z (2008) Micromechanical modelling of the effect of plastic deformation on the mechanical behaviour in pseudoelastic shape memory alloys. Int J Plast 24(8):1307–1332
Hartl D, Lagoudas D (2009) Smart Mater Struct 18:1–17
Qidwai M-A, Lagoudas D-C (2000) On thermomechanics and transformation surfaces of polycrystalline NiTi shape memory alloy material. Int J Plast 16(1309):1343
Popov P, Lagoudas D-C (2007) A 3-D constitutive model for shape memory alloys incorporating pseudoelasticity and detwinning of self-accommodated martensite. Int J Plast 23(1679):1720
Arghavani J, Auricchio F, Naghdabadi R, Reali R, Sohrabpour S (2010) A 3-D phenomenological constitutive model for shape memory alloys under multiaxial loadings. Int J Plast 26(976):991
Mirzaeifar R, Shakeri M, Sadighi M (2009) Nonlinear finite element formulation for analyzing shape memory alloy cylindrical panels. Smart Mater Struct 12(5) doi:10.1088/0964-1726/18/3/035002
Mirzaeifar R, DesRoches R, Yavari A (2010) Exact solutions for pure torsion of shape memory alloy circular bars. Mech Mater 42(797):806
Auricchio F, Marfia S, Sacco E (2003) Modelling of SMA materials: training and two way memory effects. Comput Struct 81(2301):2317
Yue Z-F, Wan J-S, Zhang Q-M (2003) Rare Metal Mater Eng 32:246
Yan W, Wang C-H, Zhang X-P, Mai Y-W (2002) Effect of transformation volume contraction on the toughness of superelastic shape memory alloys. Smart Mater Struct 11(947):955
Wang X-M, Wang YF, Baruj A, Eggeler A, Yue ZF (2005) On the formation of martensite in front of cracks in pseudoelastic shape memory alloys. Mater Sci Eng A 394(393):398
Auricchio F, Sacco E (1997) A one-dimensional model for superelastic shape memory alloys with different elastic properties between austenite and martensite Int. J NonLinear Mech 32(1101):1114
Auricchio F, Sacco E (2001) Thermo-mechanical modelling of a superelastic shape-memory wire under cyclic stretching bending loadings. Int J Solids Struct 38(6123):6145
Gao X, Huang M, Brinson L-C (2000) A multivariant micromechanicalmodel for SMAs: part 1: crystallographic issues for single crystal model. Int J Plast 16(1345):1369
Thamburaja P, Anand L (2002) Superelastic behavior in tension-torsion of an initially-textured Ti-Ni shape-memory alloy. Int J Plast 18(1607):1617
Lim T-J, McDowell D-L (2002) Cyclic thermomechanical behavior of a polycrystalline pseudoelastic shape memory alloy. J Mech Phys Solids 50(651):676
Auricchio F, Taylor RL (1997) Shape-memory alloys: modelling and numerical simulations of the finite-strain superelastic behaviour. Comput Methods Appl Mech Eng 143:175–194
Auricchio F, Taylor R-L (1996) Shape memory alloy superelastic behavior: 3D finite element simulations. In: Proceedings of the 3rd international conference on intelligent materials, Lyon June 3-5
Brinson L-C (1993) One-dimensional constitutive behavior of shape memory alloys: termomechanical derivation with non-constant material functions and redefined martensite internal variable. J Intell Mater Syst Struct 4:229–242
Brinson L-C, Lammering R (1993) Finite element analysis of the behavior of shape memory alloys and their applications. Int J Solids Struct 30(23):3261–3280
Kouzak Z, Levy Neto F, Savi M-A (1998) Finite element model for composite beams using SMA fibers. In: Proceedings of CEM NNE 98 - V Congresso de Engenharia Mecânica Norte e Nordeste, vol II, Fortaleza, Brazil, 27-30 October, 112–119
Tanaka K (1986) A thermomechanical sketch of shape memory effect: one-dimensional tensile behavior. Res Mech 18(251):263
Tanaka K, Nagaki S (1982) Thermomechanical description of materials with internal variables in the process of phase transformation. Ing Arch 51(287):299
Auricchio F, Petrini L (2002) Improvements and algorithmical considerations on a recent three-dimensional model describing stress-induced solid phase transformation. Int J Numer Methods Eng 55(1255):1284
Auricchio F, Petrini L (2004) A three dimensional model describing stress-temperature induced solid phase transformations: solution algorithm and boundary value problems. Int J Numer Methods Eng 61(807):836
Qidwai MA, Lagoudas DC (2000) Numerical implementation of a shape-memory alloy thermomechanical constitutive model using return mapping algorithms. Int J Numer Methods Eng 47(1123):1168
Author information
Authors and Affiliations
Corresponding author
Rights and permissions
About this article
Cite this article
Sahli, M.L., Necib, B. Characterisation and modelling of behaviour of a shape memory alloys. Int J Adv Manuf Technol 70, 1847–1857 (2014). https://doi.org/10.1007/s00170-013-5416-9
Received:
Accepted:
Published:
Issue Date:
DOI: https://doi.org/10.1007/s00170-013-5416-9