Abstract
The complexation of silicon with carbon materials is considered an effective method for using silicon as an anode material for lithium-ion batteries. In the present study, carbon frameworks with a 3D porous structure were fabricated using metal–organic frameworks (MOFs), which have been drawing significant attention as a promising material in a wide range of applications. Subsequently, the fabricated carbon frameworks were subjected to CVD to obtain silicon-carbon complexes. These silicon-carbon complexes with a 3D porous structure exhibited excellent rate capability because they provided sufficient paths for Li-ion diffusion while facilitating contact with the electrolyte. In addition, unoccupied space within the silicon complex, combined with the stable structure of the carbon framework, allowed the volume expansion of silicon and the resultant stress to be more effectively accommodated, thereby reducing electrode expansion. The major findings of the present study demonstrate the applicability of MOF-based carbon frameworks as a material for silicon complex anodes.







Similar content being viewed by others
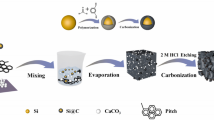
References
Hannan MA, Lipu MSH, Hussain A, Mohame A (2017) A review of lithium-ion battery state of charge estimation and management system in electric vehicle applications: Challenges and recommendations. Renew Sust Energ Rev 78:834–854
Gonzalez AF, Yang NH, Liu RS (2017) Silicon anode design for lithium-ion batteries: progress and perspectives. J Phys Chem C 121:27775–27787
Chen T, Jin Y, Lv H, Yang A, Liu M, Chen B, Xie Y, Chen Q (2020) Applications of lithium-ion batteries in grid-scale energy storage systems. Trans Tianj Univer 26:208–217
Asenbauer J, Eisenmann T, Kuenzel M, Kazzazi A, Chen Z, Bresser D (2020) The success story of graphite as a lithium-ion anode material–fundamentals, remaining challenges, and recent developments including silicon (oxide) composites. Sustain Energ Fuels 4:5387–5416
Zhao X, Lehto VP (2020) Challenges and prospects of nanosized silicon anodes in lithium-ion batteries. Nanotechnology 32:042002
Szczech JR, Jin S (2011) Nanostructured silicon for high capacity lithium battery anodes. Energy Environ Sci 4:56–72
Liu N, Wu H, McDowell MT, YaO Y, Wang C, Cui Y (2012) Kinetic competition model and size-dependent phase selection in 1-D nanostructures. Nano Lett 12:3315–3321
Li M, Hou X, Sha Y, Wang J, Hu S, Liu X, Shao Z (2014) Facile spray-drying/pyrolysis synthesis of core–shell structure graphite/silicon-porous carbon composite as a superior anode for Li-ion batteries. J Power Sour 248:721–728
Maranchi JP, Hepp AF, Evans AG, Nuhfer NT, Kumta PN (2006) Interfacial properties of the a-Si∕ Cu: active–inactive thin-film anode system for lithium-ion batteries. J Electrochem Soc 153:A1246
Wang F, Chen G, Zhang N, Liu X, Ma R (2019) Engineering of carbon and other protective coating layers for stabilizing silicon anode materials. Carbon Energy 1:219–245
Li Y, Paranthaman MP, Akato K, Naskar AK, Levine AM, Lee RJ, Manthiram A (2016) Tire-derived carbon composite anodes for sodium-ion batteries. J Power Sour 316:232–238
Dai F, Yi R, Yang H, Zhao Y, Luo L, Gordin ML, Wang D (2019) Minimized volume expansion in hierarchical porous silicon upon lithiation. ACS Appl Mater Interf 11:13257–13263
Domi Y, Usui H, Sugimoto K, Sakaguchi H (2019) Effect of silicon crystallite size on its electrochemical performance for lithium-ion batteries. Energy Technol 7:1800946
Jia H, Zheng J, Song J, Luo L, Yi R, Estevez L, Zhang JG (2018) A novel approach to synthesize micrometer-sized porous silicon as a high performance anode for lithium-ion batteries. Nano Energy 50:589–597
Magasinski A, Dixon P, Hertzberg B, Kvit A, Ayala J, Yushin G (2010) High-performance lithium-ion anodes using a hierarchical bottom-up approach. Nat Mater 9:353–358
Zou KY, Li ZX (2018) Controllable syntheses of MOF-derived materials. Eur J Chem 24:6506–6518
Ren J, Huang Y, Zhu H, Zhang B, Zhu H, Shen S, Liu Q (2020) Recent progress on MOF-derived carbon materials for energy storage. Carbon Energy 2:176–202
Ma X, Li L, Chen R, Wang C, Li H, Li H (2018) Highly nitrogen-doped porous carbon derived from zeolitic imidazolate framework-8 for CO2 Capture. Chem Asian J 13:2069–2076
Li Q, Dai Z, Wu J, Liu W, Di T, Jiang R, Zhou J (2020) Fabrication of ordered macro-microporous single-crystalline MOF and its derivative carbon material for supercapacitor. Adv Energy Mater 10:1903750
Son Y, Ma J, Kim N, Lee T, Lee Y, Sung J, Cho J (2019) Quantification of pseudocapacitive contribution in nanocage-shaped silicon–carbon composite anode. Adv Energy Mater 9:1803480
Park J, Ju J, Choi W, Kim S (2019) Highly reversible ZnO@ ZIF–8-derived nitrogen-doped carbon in the presence of fluoroethylene carbonate for high-performance lithium-ion battery anode. J Alloys Compd 773:960–969
Spinella C, Lombardo S, Priolo F (1998) Crystal grain nucleation in amorphous silicon. J Appl Phys 84:5383–5414
Viera G, Huet S, Boufendi L (2001) Crystal size and temperature measurements in nanostructured silicon using Raman spectroscopy. J Appl Phys 90:4175–4183
Lim SY (2019) Amorphous-silicon nanoshell on artificial graphite composite as the anode for lithium-ion battery. Solid State Sci 93:24–30
V. Sharova, A. Moretti, G. A. Giffin, D. V. Carvalho, S. Passerini (2017) Evaluation of carbon-coated graphite as a negative electrode material for Li-ion batteries. 3: 22
Yue L, Zhang W, Yang J, Zhang L (2014) Designing Si/porous-C composite with buffering voids as high capacity anode for lithium-ion batteries. Electrochim Acta 125:206–217
Ashuri M, He Q, Liu Y, Shaw LL (2020) Investigation towards scalable processing of silicon/graphite nanocomposite anodes with good cycle stability and specific capacity. Nano Mater Sci 2:297–308
Gao H, Wu Q, Hu Y, Zheng JP, Amine K, Chen Z (2018) Revealing the rate-limiting Li-ion diffusion pathway in ultrathick electrodes for Li-ion batteries. J Phys Chem Lett 9:5100–5104
Choi W, Shin HC, Kim JM, Choi JY, Yoon WS (2020) Modeling and applications of electrochemical impedance spectroscopy (EIS) for lithium-ion batteries. J Electrochem Sci Technol 11:1–13
Ahmadabadi VG, Shivanimoghaddam K, Kerr R, Showkath N, Naebe M (2020) Structure-rate performance relationship in Si nanoparticles-carbon nanofiber composite as flexible anode for lithium-ion batteries. Electrochim Acta 330:135232
Zhang X, Wang D, Qiu X, Ma Y, Kong D, Müllen K, Zhi L (2020) Stable high-capacity and high-rate silicon-based lithium battery anodes upon two-dimensional covalent encapsulation. Nat Commun 11:1–9
Augustyn V, Come J, Lowe MA, Kim JW, Taberna PL, Tolbert SH, Dunn B (2013) High-rate electrochemical energy storage through Li+ intercalation pseudocapacitance. Nat Mater 12:518–522
Acknowledgements
This work was also supported by the Technology Innovation Program (20006696, Development of isotropic graphite block for semiconductor process), funded by the Ministry of Trade, Industry & Energy (MOTIE, Korea). In addition, this research has been performed as a project No KK2311-30 (Development of economical low-carbon hydrogen production and storage technology from low-value carbon resources) and supported by the Korea Research Institute of Chemical Technology (KRICT).
Author information
Authors and Affiliations
Corresponding authors
Ethics declarations
Conflict of interest
The authors declare the following financial interests/personal relationships which may be considered as potential competing interests.
Additional information
Publisher's Note
Springer Nature remains neutral with regard to jurisdictional claims in published maps and institutional affiliations.
Supplementary Information
Below is the link to the electronic supplementary material.
Rights and permissions
Springer Nature or its licensor (e.g. a society or other partner) holds exclusive rights to this article under a publishing agreement with the author(s) or other rightsholder(s); author self-archiving of the accepted manuscript version of this article is solely governed by the terms of such publishing agreement and applicable law.
About this article
Cite this article
Ahn, W.J., Park, B.H., Seo, S.W. et al. Designing of 3D porous silicon/carbon complex anode based on metal-organic frameworks for lithium-ion battery. Carbon Lett. 33, 2349–2361 (2023). https://doi.org/10.1007/s42823-023-00572-6
Received:
Revised:
Accepted:
Published:
Issue Date:
DOI: https://doi.org/10.1007/s42823-023-00572-6