Abstract
The parametric resonance, found in a single floating body, discloses that the kinetic energy could be transferred from heave mode to roll mode and causes motion instability if there is an integer multiple relationship between the two mode natural frequencies. For multi-module floating structures, the event of parametric resonance has not been investigated, but important for the stability and safety design of the floating platforms. In this paper, an experimental test is carried out using five box-type floating modules in a wave flume and observes the existence of the parametric resonance between the heave mode and roll mode. A mathematical model, validated by the experiment data, is built up for the theoretical analysis of the influential factors of the parametric resonance. The effects on the motion instability of wave condition, connector stiffness and number of modules are analyzed. It reveals that an appropriate stiffness setting of the connectors could eliminate the parametric resonance of multi-module floating structures. This theoretical finding is confirmed in a further experiment test on a five-module floating structure in the wave flume.



















Similar content being viewed by others
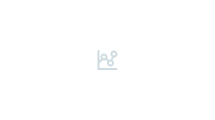
Data availability
Data will be made available on reasonable request.
References
To, C.W.S., Chen, Z.: First passage time of nonlinear ship rolling in narrow band non-stationary random seas. J. Sound Vib. 309, 197–209 (2008). https://doi.org/10.1016/j.jsv.2007.06.054
Froude, W.: Remarks on Mr. Scott-Russell’s paper on rolling. Trans. Inst. Nav. Archit. 4, 232–275 (1863)
Van Laarhoven, B.J.H.: Stability analysis of parametric roll resonance. Report, Eindhoven University of Technology Department Mechanical Engineering Dynamics and Control Group Eindhoven (2009)
Liaw, C.Y., Bishop, S.R.: Nonlinear heave-roll coupling and ship rolling. Nonlinear Dyn. 8, 197–211 (1995). https://doi.org/10.1007/BF00045774
Blocki, W.: Ship safety in connection with parametric resonance of the roll. Int. Shipbuild. Prog. 27, 36–53 (1980)
Spyrou, K.J.: Designing against parametric instability in following seas. Ocean Eng. 27, 625–653 (2000). https://doi.org/10.1016/S0029-8018(99)00019-0
Chang, B.C.: On the parametric rolling of ships using a numerical simulation method. Ocean Eng. 35, 447–457 (2008). https://doi.org/10.1016/j.oceaneng.2008.01.008
Paulling, J.R.: Parametric rolling of ships—then and now. In: Almeida Santos Neves, M., Belenky, V., de Kat, J., Spyrou, K., Umeda, N. (eds.) Contemporary Ideas on Ship Stability and Capsizing in Waves. Fluid Mechanics and Its Applications, vol 97, pp. 347–360. Springer, Dordrecht (2011). https://doi.org/10.1007/978-94-007-1482-3_19
Paulling, J., Rosenberg, R.: On unstable ship motions resulting from nonlinear coupling. J. Sh. Res. 3, 36–46 (1959)
Nayfeh, A.H., Mook, D.T., Marshall, L.R.: Nonlinear coupling of pitch and roll modes in ship motions. J Hydronaut. 7, 145–152 (1973). https://doi.org/10.2514/3.62949
Neves, M.A.S., Pérez, N.A., Rodriguez, C.A.: Hull design considerations for improved stability of fishing vessels in waves. In: Proceedings of the Eighth International Conference on the Stability of Ships and Ocean Vehicles, pp. 291–304 (2003)
Lugni, C., Greco, M., Faltinsen, O.M.: Influence of yaw-roll coupling on the behavior of a FPSO: an experimental and numerical investigation. Appl. Ocean Res. 51, 25–37 (2015). https://doi.org/10.1016/j.apor.2015.02.005
Greco, M., Lugni, C., Faltinsen, O.M.: Influence of motion coupling and nonlinear effects on parametric roll for a floating production storage and offloading platform. Phil. Trans. R. Soc. A. 373, 1–18 (2015)
Rho, J.B., Choi, H.S., Lee, W.C., Shin, H.S., Park, I.K.: Heave and pitch motions of a spar platform with damping plate. In: The Twelfth International Offshore and Polar Engineering Conference, Kitakyushu, Japan, pp. 198–201 (2002)
Lim, S.J., Rho, J.B., Choi, H.S.: An experimental study on motion characteristics of cell spar platform. In: The Fifteenth International Offshore and Polar Engineering Conference, Seoul, Korea, pp. 233–237 (2005)
Koo, B.J., Kim, M.H.Ã., Randall, R.E.: Mathieu instability of a spar platform with mooring and risers. Ocean Eng. 31, 2175–2208 (2004). https://doi.org/10.1016/j.oceaneng.2004.04.005
Liu, L., Zhou, B., Tang, Y.: Study on the nonlinear dynamical behavior of deepsea Spar platform by numerical simulation and model experiment. J. Vib. Control. 20, 1528–1537 (2014). https://doi.org/10.1177/1077546312472917
Giorgi, G., Gomes, R.P.F., Bracco, G., Mattiazzo, G.: Numerical investigation of parametric resonance due to hydrodynamic coupling in a realistic wave energy converter. Nonlinear Dyn. 101, 153–170 (2020). https://doi.org/10.1007/s11071-020-05739-8
Yang, H., Xu, P.: Effect of hull geometry on parametric resonances of spar in irregular waves. Ocean Eng. 99, 14–22 (2015). https://doi.org/10.1016/j.oceaneng.2015.03.006
Wei, H., Xiao, L., Tian, X., Low, Y.M.: Nonlinear coupling and instability of heave, roll and pitch motions of semi-submersibles with bracings. J. Fluids Struct. 83, 171–193 (2018)
Wei, H., Xiao, L., Low, Y.M., Tian, X., Liu, M.: Effects of bracings and motion coupling on resonance features of semi-submersible platform under irregular wave conditions. J. Fluids Struct. 92, 102783 (2020). https://doi.org/10.1016/j.jfluidstructs.2019.102783
Watanabe, E., Utsunomiya, T., Wang, C.M.: Hydroelastic analysis of pontoon-type VLFS: a literature survey. Eng. Struct. 26, 245–256 (2004). https://doi.org/10.1016/j.engstruct.2003.10.001
Wang, C.M., Tay, Z.Y.: Very large floating structures: applications, research and development. Procedia Eng. 14, 62–72 (2011). https://doi.org/10.1016/j.proeng.2011.07.007
Maeda, H., Inoue, R., Togawa, S., Maruyama, S., Watanabe, K., Suzuki, F.: On the motions of a floating structure which consists of two or three blocks with rigid or pin joints. Nav. Archit. Ocean Eng. 17, 91–98 (1979)
Fu, S.X., Moan, T., Chen, X.J., Cui, W.C.: Hydroelastic analysis of flexible floating interconnected structures. Ocean Eng. 34, 1516–1531 (2007). https://doi.org/10.1016/j.oceaneng.2007.01.003
Xia, S.Y., Xu, D.L., Zhang, H.C., Wu, Y.S.: Multivariable oscillation control of a modularized floating airport with disturbance of uncertain waves. J. Sound Vib. 439, 310–328 (2019). https://doi.org/10.1016/j.jsv.2018.10.006
Tay, Z.Y., Wang, C.M., Utsunomiya, T.: Hydroelastic responses and interactions of floating fuel storage modules placed side-by-side with floating breakwaters. Mar. Struct. 22, 633–658 (2009). https://doi.org/10.1016/j.marstruc.2008.11.002
Zhang, H.C., Xu, D.L., Lu, C., Qi, E.R., Hu, J.J., Wu, Y.S.: Amplitude death of a multi-module floating airport. Nonlinear Dyn. 79, 2385–2394 (2015). https://doi.org/10.1007/s11071-014-1819-x
Koh, H.S., Lim, Y.B.: The floating platform at the Marina Bay, Singapore. Struct. Eng. Int. 19, 33–37 (2009). https://doi.org/10.2749/101686609787398263
Waals, O.J., Bunnik, T.H.J., Otto, W.J.: Model test and numerical analysis for a floating mega island. In: Proceedings of the 37th International Conference on Ocean, Offshore & Arctic Engineering (OMAE2018), pp. 1–11 (2018)
Ding, R., Liu, C.R., Zhang, H.C., Xu, D.L., Shi, Q.J., Yan, D.L., Yang, W.Y., Chen, W.P., Wu, S.: Experimental investigation on characteristic change of a scale-extendable chain-type floating structure. Ocean Eng (2020). https://doi.org/10.1016/j.oceaneng.2020.107778
Chakrabarti, S.K.: Offshore Structure Modeling. World Scientific Publishing, Singapore (1994)
Kou, Y., Xiao, L., Tao, L.: Performance characteristics of a conceptual ring-shaped spar-type VLFS with double-layered perforated-wall breakwater. Appl. Ocean Res. 86, 28–39 (2019). https://doi.org/10.1016/j.apor.2019.02.011
Stoker, J.J.: Water Waves: The Mathematical Theory with Applications. Wiley, New Jersey (1992)
Ertekin, R.C., Riggs, H.R., Che, X.L., Du, S.X.: Efficient methods for hydroelastic analysis of very large floating structures. J. Sh. Res. 37, 58–76 (1993)
Riggs, H.R., Ertekin, R.C., Mills, T.R.J.: A Comparative study of RMFC and FEA models for the wave-induced response of a MOB. Mar. Struct. 13, 217–232 (2000). https://doi.org/10.1016/S0951-8339(00)00029-0
Zhao, J., Tang, Y., Shen, W.: A study on the combination resonance response of a classic spar platform. J. Vib. Control. 16, 2083–2107 (2010). https://doi.org/10.1177/1077546309349393
Gavassoni, E., Gonçalves, P.B., Roehl, D.M.: Nonlinear vibration modes and instability of a conceptual model of a spar platform. Nonlinear Dyn. 76, 809–826 (2014). https://doi.org/10.1007/s11071-013-1171-6
Strang, G.: Computational Science and Engineering. Wellesley-Cambridge Press, Wellesley (2007)
Zhang, H.C., Xu, D.L., Xia, S.Y., Lu, C., Qi, E.R., Tian, C., Wu, Y.S.: Nonlinear network modeling of multi-module floating structures with arbitrary flexible connections. J. Fluids Struct. 59, 270–284 (2015). https://doi.org/10.1016/j.jfluidstructs.2015.09.012
Zhang, X., Zhang, H., Zhou, X., Sun, Z.: Recent advances in wave energy converters based on nonlinear stiffness mechanisms. Appl. Math. Mech. 43, 1081–1108 (2022)
Funding
This research work was supported by the National Natural Science Foundation of China (Grant No. 52071138), the Natural Science Foundation of Hunan Province (Grant No 2022JJ30120) and the High-tech Ship Research Projects Sponsored by the Ministry of Industry and Information Technology (Grant No. [2019]357).
Author information
Authors and Affiliations
Contributions
RD, HZ and DX participated in the design of experiment and the data analysis. RD carried out the wave flume experiment, the data acquisition, the numerical analysis and the manuscript editing. HZ and DX performed the manuscript review. CL and QS helped in conducting the experiment. WZ, JL and YW contributed in the conception and design of this work. All authors have read and approved the content of the manuscript.
Corresponding author
Ethics declarations
Conflict of interest
The authors declare that there is no conflict of interests regarding the publication of this paper.
Additional information
Publisher's Note
Springer Nature remains neutral with regard to jurisdictional claims in published maps and institutional affiliations.
Rights and permissions
Springer Nature or its licensor (e.g. a society or other partner) holds exclusive rights to this article under a publishing agreement with the author(s) or other rightsholder(s); author self-archiving of the accepted manuscript version of this article is solely governed by the terms of such publishing agreement and applicable law.
About this article
Cite this article
Ding, R., Zhang, H., Xu, D. et al. Experimental and numerical study on motion instability of modular floating structures. Nonlinear Dyn 111, 6239–6259 (2023). https://doi.org/10.1007/s11071-022-08163-2
Received:
Accepted:
Published:
Issue Date:
DOI: https://doi.org/10.1007/s11071-022-08163-2