Abstract
Background
Antimicrobial resistance is one of the common global public health problems. The emergence of antimicrobial resistance is multifactorial, and tackling its development is challenging. Consequently, infections caused by resistant bacteria are unresponsive to conventional drugs, resulting in prolonged and severe illnesses, higher mortality rates, and considerable healthcare costs. Therefore, understanding the antimicrobial resistance profiles of bacterial pathogens is essential to optimize treatments and reduce the risks associated with infections. This study aimed to determine the antimicrobial resistance patterns of bacterial isolates from different clinical specimens at the Ethiopian Public Health Institute (EPHI).
Materials and methods
The retrospective cross-sectional study was conducted on the bacterial culture and antibiotic susceptibility reports of different clinical specimens referred to the Bacteriology Laboratory of EPHI from September 2015 to August 2019. Standard bacteriological techniques were used for the isolation and identification of the bacteria. Data were extracted from 840 patients’ records, which included the type of clinical sample cultured, the name of the bacteria, the representations of the antibiotics used for susceptibility testing, and the susceptibility results. Descriptive statistics were used to describe the bacterial isolates and the antimicrobial resistance profiles.
Results
Eight types of clinical specimens were analyzed for bacterial isolates and urine specimens were the most analyzed. Ten different genera of bacteria were identified by culture. Almost all the isolates were gram-negative bacteria, while only one species of gram-positive (Staphylococcus aureus) was reported. Antibiotic sensitivity patterns were tested on 840 culture isolates. Escherichia coli strains revealed more than 57% resistance to seventeen antibiotics. Klebsiella pneumoniae showed nearly 70% or greater resistance rates for 17 of the antibiotics used. The overall detected multidrug resistance (MDR) was 64.29%. The highest MDR was reported in Acinetobacter strains (84%) followed by K. pneumoniae (80%).
Conclusions
The multidrug resistance rates found in this study were alarming. Strengthening antimicrobial resistance surveillance at the national level is mandatory, and antimicrobial sensitivity testing should be accessible at local diagnostic centers.
Similar content being viewed by others
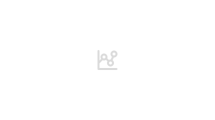
Introduction
Antimicrobial resistance (AMR) is a phenomenon that occurs when different groups of microorganisms shift over a period and no longer respond to medicines that have been effective so far [1]. Although AMR is a natural process, the public health emergency due to the uncontrolled spread of this phenomenon is the consequence of overuse and/or misuse of antibiotics [2]. However, other driving factors are also mainly responsible for the increase in its prevalence. These include poor community hygiene, poor infection control in hospitals and clinics, the accumulation of antibiotics in the environment, and their use in the animal and food industries [3, 4]. Consequently, infections caused by resistant microorganisms often fail to respond to conventional treatment, resulting in prolonged illness, severe illness, the risk of disease spreading, a greater risk of death, and higher costs. In addition, new resistance mechanisms have emerged, making the latest generation of antibiotics virtually ineffective [5].
AMR is a global health and development threat that emerged as one of the major public health problems of the 21st century and warns against the effective prevention and treatment of an ever-increasing range of infections [6]. The World Health Organization (WHO) has declared that AMR is one of humanity's top 10 global public health threats [1]. It has been noted over many decades that bacteria causing common or severe infections have developed resistance to every new antibiotic entering the market [3, 6]. Tackled by this reality, the need to prevent a rising global crisis in health care is crucial, requiring urgent multisectoral action to achieve the Sustainable Development Goals.
The World Health Organisation (WHO) has recognized for a long time that a better, more coordinated global effort is required to combat AMR. In 2001, the WHO Global Strategy for Containment of Antimicrobial Resistance provided an agenda of interferences to slow the emergence and reduce the distribution of antimicrobial-resistant microorganisms [7]. In 2012, WHO published ‘The Evolving Threat of Antimicrobial Resistance: Options for Action’ [8], proposing a combination of interventions that include strengthening health systems and surveillance, improving the use of antimicrobials in hospitals and the community, infection prevention and control, encouraging the development of appropriate new drugs and vaccines, and political commitment.
In 2014, following the proposed role of the surveillance system, WHO published the first global report on the surveillance of AMR, collecting experiences from national and international surveillance networks [9]. This report showed that surveillance data, where available, can be beneficial for orienting treatment choices, understanding AMR trends, identifying priority areas for interventions, and monitoring the impact of interventions to contain resistance. However, the lack of adequate surveillance in many parts of the world leaves significant gaps in existing knowledge of the distribution and extent of this phenomenon. In 2017, the WHO first published a list of 12 families of bacteria that pose the greatest threat to human health [10]. The WHO's list categorizes bacteria into three priority categories: critical, high, and medium priority, according to the need to develop new antibiotics to combat these pathogens [11].
The problem of antimicrobial resistance is not only the cause of the development of the resistance but also the transmission of the resistant strains from one person to another, especially in a health facility setting. The problem worsens in countries where poor sanitation makes transmitting the bacteria easy [12]. In addition, Ethiopia has a shortage of clinical microbiology laboratories to identify the specific etiologic agents and their antimicrobial susceptibility testing [13]. Therefore, treating most bacterial infections is usually done empirically, leading to an AMR emergency. AMR development in Ethiopia was mostly influenced locally by self-antibiotic prescription, lack of access to local antibiogram data, and low prescriber understanding of AMR [12, 13].
Understanding and acting on the local or national AMR situation is critical to gaining consensus on implementing appropriate interventions [14]. Furthermore, identifying the most common bacterial pathogens and their respective AMR profiles would be valuable to optimize treatment and reduce morbidity and mortality associated with infectious disease. Thus, up-to-date information on microbial resistance is needed at local and national levels to guide the rational use of the existing antimicrobials. Therefore, this study aimed to determine the antimicrobial resistance patterns of the bacterial isolate from different clinical specimens referred to EPHI.
Materials and methods
Study design, period, and setting
A retrospective cross-sectional study was conducted on bacterial culture and antibiotic susceptibility reports of different clinical specimens referred to the Bacteriology laboratory of the Ethiopian Public Health Institute (EPHI) between September 2015 and August 2019. The EPHI was established by the Council of Ministers regulation No. 301/2013. It recognizes the institute as an autonomous federal government office with its personality and is accountable to the Ethiopian Federal Minister of Health. The EPHI works with the mission of improving the health of the general public of the country through undertaking research on the priority health and nutrition issues for evidence-based information using and applying technology transfer, effective public health emergency management, establishing quality laboratory system, and training public health researchers and practitioners for the best public health interventions (https://ephi.gov.et/about-us/). Among the institute's vast activities of clinical laboratory services, strengthening national systems for referral specimen transportation, result delivery, and backup testing services are the ones (https://ephi.gov.et/laboratory-services/).
Data extraction
A total of 840 records with complete information were selected for data extraction. The information extracted from the laboratory included the sex and age of patients, the type of clinical sample cultured, the name of bacteria isolated, names of antibiotics used for susceptibility testing, and the susceptibility results recorded in the laboratory report.
Specimen collection, processing, and bacterial identifications
The standard operating procedures (SOPs) of collecting, storing, and transporting different clinical specimens were implemented, and specimens were referred to EPHI Bacteriology Laboratory. The collected clinical samples were delivered to the laboratory and processed following standard procedures. Bacteriological analyses were performed in the laboratory following SOPs adopted from the Clinical and Laboratory Standards Institute (CLSI) guidelines. Clinical samples, including urine, blood, sputum, wound/pus, cerebrospinal fluid, body fluid, discharge (ear/eye), and tracheal aspirate, were cultured. Each clinical sample employed standard microbiological culturing techniques. Specimens were inoculated into the appropriate isolation culture media and incubated at 35 to 37 °C conferring to standard protocols for each sample [15]. Bacterial identification was made mainly based on the colony characteristics, gram-stain reaction, and proper biochemical tests as per suitability according to CLSI guidelines and developed SOPs.
Antimicrobial susceptibility testing
Antimicrobial sensitivity testing (AST) was performed using the Kirby Bauer disk diffusion technique on Mueller-Hinton agar. Standard discs with specified concentrations were used to detect the resistance patterns of each isolate. The plates were incubated overnight. After incubation was completed, the zone inhibition diameter was measured in millimeters (mm). The zones were interpreted as susceptible, intermediate, or resistant according to CLSI [16]. The definition of CDC was used in this study for multidrug resistance (MDR): resistance of bacterial isolates to at least one antibiotic in three or more drug classes (https://www.cdc.gov/narms/resources/glossary.html). The following standard antibiotics, with abbreviated names and disk contents in brackets, were used to test the resistance profiles of bacterial isolates: Amikacin (AMK) (30µg), Amoxicillin (AMX) (10µg), Amoxicillin/clavulanic acid (AMC) (20/10µg), Ampicillin (AMP) (10µg), Cefepime (FEP) (30µg), Cefamandole (CFM) (30µg), Cefotaxime (CTX) (30µg), Cefoxitin (FOX) (30µg), Ceftazidime (CAZ) (30µg), Ceftriaxone (CRO) (30µg), Cefuroxime (CXM) (30µg), Ciprofloxacin (CIP) (5µg), Chloramphenicol (CHL) (30µg), Clarithromycin (CLI) (15µg), Doripenem (DOR) (30µg), Erythromycin (ERY) (15µg), Gentamicin (GEN) (10µg), Meropenem (MEM) (10µg), Nalidixic acid (NAL) (30µg), Nitazoxanide (NIT) (300µg), Norfloxacin (NOR) 10µg), Oxacillin (OXA) (30µg), Penicillin (PEN) (10 units), Piperacillin (PIP) (100µg), Tazobactam (TZP) (100/10µg), Tobramycin (TOB) (10µg), Sulfamethoxazole-Trimethoprim (SXT) (1.25/23.75µg), Imipenem (IMP) (10µg), Cefazolin (KZ) (15µg), Tetracycline (TET) (15µg), Cefoperazone (CFP) (75µg) and Cefuroxime (CRX) (30µg).
Quality control
American Typing Culture Collection isolates of Escherichia coli (ATCC-25922), Staphylococcus aureus (ATCC-25923), and Pseudomonas aeruginosa (ATCC-27853) were used as reference strains in the laboratory.
Data analysis
The data entry was done using Microsoft Office Excel, and then it was imported to STATA version 14 for further analysis. Descriptive statistics were used to designate the demographic characteristics of the participants and the bacteriological and antimicrobial resistance profiles of the isolates.
Results
Profile of patients, clinical specimens, and bacterial isolates
Clinical specimens were collected from 840 patients; females accounted for 51.07% (n = 429). Most of the patients were in the age category of greater than 15 years old, 70.71% (n = 594). Eight different types of clinical specimens were analyzed for bacterial isolates, where urine specimens were the most analyzed, 64.52% (542/840). Analyzed by culture technique, ten different genera of bacteria were identified from the clinical samples. Almost all the isolates were gram-negative bacteria with only one species of gram-positive, Staphylococcus aureus. More than half of the gram-negative isolates reported were Escherichia coli (51.43%; 432/840). Please, refer to Table 1.
Bacterial isolates from various clinical specimens
Table 2 shows the type and frequency of bacterial isolates from the analyzed clinical specimens. Escherichia coli were identified from all types of clinical specimens with the majority from urine specimens, 83.3% (360/432). Similarily, Klebsiella pneumoniae was identified from all the analyzed specimens with the highest frequency from blood samples, 42.2% (95/225).
Antibiotic resistance patterns of bacterial isolates
The antibiotic resistance patterns were tested for 840 bacterial isolates over five years, from September 2015 to August 2019. As data are shown in Additional file 1: Table S1, different antibiotics were used to evaluate the resistance patterns of the bacterial isolates.
Escherichia coli strains were tested for 29 different antibiotics. For the fifteen antibiotics used, the E. coli strains revealed resistance of more than 57% (57.8% to 100%). The strains showed resistance of 98.9% and 100% to Ampicillin (AMP) and Cefuroxime (CXM), respectively. However, E. coli strains did not show resistance against Amoxicillin/clavulanic acid (AMC) and Chloramphenicol (CHL). The resistance patterns of K. pneumoniae were evaluated using 27 types of antibiotics. Nearly 70% and greater resistances were recorded for 17 antibiotics applied. On the other hand, lower resistances, less than 11%, were reported to a few antibiotics such as Amikacin (AMK), Doripenem (DOR), Erythromycin (ERY), and Imipenem (IMP) while no resistance was reported to Amoxicillin/clavulanic acid (AMC). Acinetobacter spp showed 100% resistance for the majority of the drugs: Cefepime (FEP), Cefotaxime (CTX), Ceftazidime (CAZ), Ceftriaxone (CRO), Cefuroxime (CXM), Nalidixic acid (NAL), Nitazoxanide (NIT), Tazobactam (TZP), and Tetracycline (TET). The resistance profiles of S. aureus strains were checked against eight drugs, and the strains showed no resistance for three antibiotics, such as Cefoxitin (FOX), Clarithromycin (CLI), and Gentamicin (GEN). Resistances of 70% and 50% were revealed against Penicillin (PEN) and Ciprofloxacin (CIP), respectively (Additional file 1: Table S1).
Multidrug resistance (MDR) profiles of bacterial isolates
Overall, multidrug resistance (MDR) was 64.29% (540/840). The highest MDR was reported in Acinetobacter strains, 84% (21/25). The proportions of MDR - K. pneumoniae and MDR - E. coli were 80% (180/225) and 62.02% (268/432), respectively (Table 3).
Discussion
Antibiotic-resistant bacterial infections are among the most challenging public health concerns, especially in developing countries. The absence of effective antibiotic treatment will challenge clinicians to manage infectious diseases and their complications, particularly in immune-suppressed patients. This study assessed the antimicrobial resistance profiles of bacterial isolates from different clinical specimens referred to the Ethiopian Public Health Institute from the hospitals in Addis Ababa and the surrounding areas. This study showed that gram-negative bacteria were predominantly isolated from most clinical samples, which aligns with a study conducted in Northwest Ethiopia [13] and Tanzania [17]. The major bacterial isolate was recovered from the urine sample, which was 64.5% (542/840), followed by a blood sample of 19.05% (160/840). Therefore, most isolated bacteria in this review were gram-negative (98.33%), which varies from a study done in southwestern Ethiopia in which the most isolated bacteria (60%) were gram-positive [18]. The difference may be due to the large number of samples and types of clinical samples used in the studies used for this review. In addition, the isolated bacteria susceptibility test in the review was multidrug-resistant (85%), which is synonymous (81%) with the study done at a Referral Hospital in Northwest Ethiopia [19].
E. coli was the most commonly isolated bacterial pathogen, followed by K. pneumoniae; from all 840 isolates, E. coli accounts for 51.43%, regardless of specimen type this finding agrees with other studies done in India (53.3%) [20]. On the other hand, E. coli was the leading (51.43%) bacteria isolated among the others, not synonymous with a study done in Jimma University Specialized Hospital (JUSH) from April to August 2016 (25.4%), there is a high variation in percentage [21]. Therefore, the percentage difference may be due to sampling size variation. But more elevated than the study done in Hawassa tertiary hospital, Ethiopia (16.7%) [22], in Arbaminch General Hospital Ethiopia (40.5%) [23], Jimma, Ethiopia(42.0%) [24], Yemen(46.3%) [25]. The second most common gram-negative bacteria isolated from our study was Klebsiella pneumoniae (26.79%) which is higher than the study done in Saudi Arabia (20%) [26], Yemen (18.5%) [23], and India(17%) [27] but lower than the study done in Vinayaka Missions Medical College, India (41.55%) [28].
In our study, the overall prevalence MDR result showed a high rate of bacterial isolates, which was 64.29% (540/840). This result is lower than the studies done by Mengistu H. et al. [29], Ten Hove RJ. et al. [30], and Beyene D. et al. [31]. But in agreement with the studies done in Debre Markos Comprehensive Specialized Hospital (59%) [32], Gondar (64.6%) [32], and St. Paul’s Hospital Millennium Medical College (66.4%) [33], southern Ethiopia (69.9%) [34]. The most concerning part of this study was that a significant number of bacterial isolates showed drug resistance against the majority of antibiotics used for sensitivity testing. E. coli, Klebsiella spp, Acinetobacter spp, and Citrobacter spp were highly resistant to commonly prescribed drugs like Sulfamethoxazole-Trimethoprim (Cotrimoxazole), Ceftriaxone, Ampicillin, Ciprofloxacin, Cefotaxime, Cefepime, and Ceftazidime. However, these bacteria are highly susceptible to Amoxicillin/clavulanic acid, Amikacin, Doripenem, Meropenem, and Imipenem. The overall observed high rate of MDR could be linked to irrational use and/or self-medication of antibiotics, possibly contributing to the resistance rates in the study area. This study has some limitations. Since our study was retrospective, it could not indicate the current antimicrobial resistance patterns of the isolates. This study also couldn’t determine whether the identified resistance was due to hospital-acquired or community-acquired.
Conclusions
The most prevalent bacteria in this study, Escherichia coli, showed resistance of more than 57% to seventeen different antibiotics, while the next prevalent bacteria, Klebsiella pneumoniae, revealed nearly 70% or greater resistance rates. Overall, the multidrug resistance rates found in this study were alarming, 64.29%. Therefore, strengthening antimicrobial resistance surveillance at the national level, and antimicrobial sensitivity testing at local diagnostic centers are very important in reducing the challenges of antimicrobial resistance.
Availability of data and materials
All the data are available with the corresponding author.
Change history
02 January 2024
A Correction to this paper has been published: https://doi.org/10.1186/s12879-023-08921-6
References
WHO. Antimicrobial Resistance. 17 November 2021. Available at: https://wwwwhoint/news-room/fact-sheets/detail/antimicrobial-resistance. Accessed on 14 Oct 2022.
Coculescu BI. Antimicrobial resistance induced by genetic changes. J Med Life. 2009;2(2):114.
WHO. Antibiotic resistance. 31 July 2020. Available at: https://wwwwhoint/news-room/fact-sheets/detail/antibiotic-resistance. Accessed on 14 Oct 2022.
Collignon P, Beggs JJJA. Socioeconomic enablers for contagion: factors impelling the antimicrobial resistance epidemic. Antibiotics. 2019;8(3):86.
Jindal A, Pandya K, Khan ID. Antimicrobial resistance: a public health challenge. Med J Armed Forces India. 2015;71(2):178–81.
Prestinaci F, Pezzotti P, Pantosti A. Antimicrobial resistance: a global multifaceted phenomenon. Pathog Glob Health. 2015;109:309–18.
WHO. WHO global strategy for containment of antimicrobial resistance. World Health Organization; 2001. Available at: https://iris.who.int/bitstream/handle/10665/66860/WHO_CDS_CSR_DRS_2001.2.pdf.
WHO. The evolving threat of antimicrobial resistance: options for action. Geneva: World Health Organization; 2012. Available at: https://iris.who.int/bitstream/handle/10665/44812/?sequence=1.
WHO. Antimicrobial resistance: global report on surveillance. Geneva: World Health Organization; 2014. Available at: https://iris.who.int/bitstream/handle/10665/112642/?sequence=1.
Mulani MS, Kamble EE, Kumkar SN, Tawre MS, Pardesi KR. Emerging strategies to combat ESKAPE pathogens in the era of antimicrobial resistance: a review. Front Microbiol. 2019;10:539.
De Oliveira DM, Forde BM, Kidd TJ, Harris PN, Schembri MA, Beatson SA, et al. Antimicrobial resistance in ESKAPE pathogens. Clin Microbiol Rev. 2020;33(3):e00181-e219.
Abera B, Kibret M, Mulu WJ. Knowledge and beliefs on antimicrobial resistance among physicians and nurses in hospitals in Amhara Region Ethiopia. BMC Pharmacol Toxicol. 2014;15(1):1–7.
Abebe M, Tadesse S, Meseret G, Derbie AJ. Type of bacterial isolates and antimicrobial resistance profile from different clinical samples at a Referral Hospital, Northwest Ethiopia: five years data analysis. BMC Res Notes. 2019;12(1):1–6.
Joshi MP, Chintu C, Mpundu M, Kibuule D, Hazemba O, Andualem T, et al. Multidisciplinary and multisectoral coalitions as catalysts for action against antimicrobial resistance: implementation experiences at national and regional levels. Glob Public Health. 2018;13(12):1781–95.
Monica C. District laboratory practice in tropical countries. United Kingdom: Cambridge University Press; 2006.
Wayne P. Performance Standards for Antimicrobial Susceptibility Testing. USA: 30th CLSI supplement M100. CLSI; 2020.
Mnyambwa NP, Mahende C, Wilfred A, Sandi E, Mgina N, Lubinza C, et al. Antibiotic susceptibility patterns of bacterial isolates from routine clinical specimens from Referral Hospitals in Tanzania: a prospective hospital-based observational study. Infection and drug resistance. 2021;14:869–78.
Gebre-Sealsssie S. Antimicrobial resistance patterns of clinical bacterial isolates in southwestern Ethiopia. Ethiop Med J. 2007;45(4):363–70.
Abosse S, Genet C, Derbie A. Antimicrobial Resistance Profile of Bacterial Isolates Identified from Surgical Site Infections at a Referral Hospital. Northwest Ethiopia Ethiop J Health Sci. 2021;31(3):635–44.
Koshariya M, Songra M, Namdeo R, Chaudhary A, Agarwal S, Rai A. Prevalence of pathogens and their antimicrobial susceptibility in catheter associated urinary tract infection. 2015.
Gashe F, Mulisa E, Mekonnen M, Zeleke G. Antimicrobial resistance profile of different clinical isolates against third-generation cephalosporins. J Pharm (Cairo). 2018;2018:5070742.
Alemayehu T, Ali M, Mitiku E, Hailemariam M. The burden of antimicrobial resistance at tertiary care hospital, southern Ethiopia: a three years’ retrospective study. BMC Infect Dis. 2019;19:1–8.
Oumer Y, Regasa Dadi B, Seid M, Biresaw G, Manilal A. Catheter-associated urinary tract infection: Incidence, associated factors and drug resistance patterns of bacterial isolates in southern ethiopia. Infect Drug Resist. 2021:14:2883–94.
Awoke N, Kassa T, Teshager L. Magnitude of biofilm formation and antimicrobial resistance pattern of bacteria isolated from urinary catheterized inpatients of Jimma university medical center Southwest Ethiopia. Int J Microbiol. 2019;2019:5729568.
Saleh AM, Al-Shamahy HA, Al-Hrazi RMA, Jaadan BM, AL-Magrami RTF, Al-Sharani AA. Biofilm formation and antibiotic susceptibility of uropathogens in patients with catheter associated urinary tract infections in ibb City-Yemen. Universal J Pharm Res. 2019;4(6):1–7.
Saleem M, Syed Khaja AS, Hossain A, Alenazi F, Said KB, Moursi SA, et al. Catheter-associated urinary tract infection in intensive care unit patients at a tertiary care hospital, Hail, Kingdom of Saudi Arabia. Diagnostics. 2022;12(7):1695.
Singh A, Kujur A, Lakshmi M, Daharwal A. The study of antibiotic-sensitivity and resistance pattern of bacteria causing catheter associated urinary tract infection. Int J Reprod Contracept Obstet Gynecol. 2020;9:3196.
Divyashanthi C, Adithiyakumar S, Bharathi N. Study of prevalence and antimicrobial susceptibility pattern of bacterial isolates in a tertiary care hospital. International Journal of Pharmacy and Pharmaceutical Sciences. 2015;7(1):185–90.
Hailemariam M, Alemayehu T, Tadesse B, Nigussie N, Agegnehu A, Habtemariam T, et al. Major bacterial isolate and antibiotic resistance from routine clinical samples in Southern Ethiopia. Sci Rep. 2021;11(1):19710.
Ten Hove R-J, Tesfaye M, Ten Hove WF, Nigussie M. Profiling of antibiotic resistance of bacterial species recovered from routine clinical isolates in Ethiopia. Ann Clin Microbiol Antimicrob. 2017;16(1):1–8.
Beyene D, Bitew A, Fantew S, Mihret A, Evans M. Multidrug-resistant profile and prevalence of extended spectrum β-lactamase and carbapenemase production in fermentative Gram-negative bacilli recovered from patients and specimens referred to National Reference Laboratory, Addis Ababa, Ethiopia. PLoS One. 2019;14(9):e0222911.
Haile Z, Mengist HM, Dilnessa T. Bacterial isolates, their antimicrobial susceptibility pattern, and associated factors of external ocular infections among patients attending eye clinic at Debre Markos Comprehensive Specialized Hospital, Northwest Ethiopia. PLoS One. 2022;17(11):e0277230.
Aklilu A, Bitew A, Dessie W, Hailu E, Asamene N, Mamuye Y. Prevalence and drug susceptibility pattern of bacterial pathogens from ocular infection in St. Paul’s Hospital Millennium Medical College, Ethiopia. J Bacteriol Mycol. 2018;5(8):1085.
Aweke T, Dibaba G, Ashenafi K, Kebede M. Bacterial pathogens of exterior ocular infections and their antibiotic vulnerability pattern in southern Ethiopia. Afr J Immunol Res. 2014;1(1):019–25.
Acknowledgements
The authors would like to acknowledge the Ethiopian Public Health Institute for allowing the utilization of the data.
Funding
Not applicable.
Author information
Authors and Affiliations
Contributions
B.T.R., W.T., D.E., and T.A. conceptualized the study. B.T.R., W.T., and D.E. were involved in data analysis and interpretation, and drafting the manuscript. B.T. and T.A. revised the manuscript. D.B., A.A., A.A., D.S., and G.T. participated in laboratory work and interpretation, and data cleaning. All authors read and approved the final manuscript.
Corresponding author
Ethics declarations
Ethics approval and consent to participate
All methods applied in this work were carried out by following guidelines and regulations. All experimental protocols were performed in line with the prepared standard operating procedures (SOPs) approved by the EPHI. The study was approved by the ethics committee of College of Medicine and Health Sciences, Ambo University. Informed consent was obtained from all subjects and/or their legal guardian(s) during specimen collection. A letter of permission to use antimicrobial resistance data was written to EPHI from Ambo University College of Medicine and Health Sciences. After obtaining permission from the EPHI, the information recorded in electronic data was utilized for data extraction. Patient records were anonymized before analysis.
Consent for publication
Not applicable.
Competing interests
The authors declare no competing interests.
Additional information
Publisher’s Note
Springer Nature remains neutral with regard to jurisdictional claims in published maps and institutional affiliations.
This article has been updated to correct 2 author names.
Supplementary Information
Additional file 1: Table S1.
Antibiotic resistance patterns of bacterial isolates.
Rights and permissions
Open Access This article is licensed under a Creative Commons Attribution 4.0 International License, which permits use, sharing, adaptation, distribution and reproduction in any medium or format, as long as you give appropriate credit to the original author(s) and the source, provide a link to the Creative Commons licence, and indicate if changes were made. The images or other third party material in this article are included in the article's Creative Commons licence, unless indicated otherwise in a credit line to the material. If material is not included in the article's Creative Commons licence and your intended use is not permitted by statutory regulation or exceeds the permitted use, you will need to obtain permission directly from the copyright holder. To view a copy of this licence, visit http://creativecommons.org/licenses/by/4.0/. The Creative Commons Public Domain Dedication waiver (http://creativecommons.org/publicdomain/zero/1.0/) applies to the data made available in this article, unless otherwise stated in a credit line to the data.
About this article
Cite this article
Regassa, B.T., Tosisa, W., Eshetu, D. et al. Antimicrobial resistance profiles of bacterial isolates from clinical specimens referred to Ethiopian Public Health Institute: analysis of 5-year data. BMC Infect Dis 23, 798 (2023). https://doi.org/10.1186/s12879-023-08803-x
Received:
Accepted:
Published:
DOI: https://doi.org/10.1186/s12879-023-08803-x