Abstract
‘Closed loop’ end-of-life strategies such as remanufacturing must be applied to create eco-efficient products. Remanufacturing may be a key element in reducing the environmental impact of products, but this remains to be proved. The aim of this study is to help designers evaluate the environmental impacts of their remanufacturing process during the design phase. The first task is to identify, list and classify the various remanufacturing processes (disassembly, cleaning, sorting and controlling, reconditioning, reassembly) by the type of process and then estimate the environmental impact for each process. These processes are then formalized by characterization and organized in a database. Using a simulator, the different processes can be aggregated to assess the environmental impacts of a remanufacturing line. An example is presented in the last part of this paper to illustrate the proposal.
Similar content being viewed by others
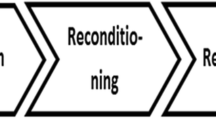
Avoid common mistakes on your manuscript.
Background
Introduction
Technology currently has a considerable impact on production. Advances in tools such as machinery, computer technology and information technology make production easier, quicker and cheaper. However, this has indirectly created environmental problems such as more waste because large volumes of products reach the end of their lifetimes. To solve these problems, many programs have been introduced and governments are placing pressure on manufacturers to recover and exploit these products [1].
Several strategies exist for managing the end-of-life of a system. One of them is the closed loop end-of-life strategy or ‘Cradle to Cradle’ system. This strategy outlines a life cycle of a product or service, in which the ‘good’, once beyond effective use, is reintegrated in the manufacturing process to form an autonomous process (loop) that requires little or no new input to function. This strategy is believed to be a favourable option for the environment and will be reviewed in this study.
Figure 1 shows several options for the closed-loop end-of-life strategy. Reuse implies that items are used by a second customer without prior repair operations or as originally designed [2], whereas recycling is the process of taking a component material and processing it to make the same material or useful degraded material [2]. Current proposals for closed-loop end-of-life strategy focus on the material recycling process. While it is certainly successful in retrieving raw materials, it also results in the loss of product shape [1]. This destroys most of the product's added value [3] and may represent a significant environmental impact [4].
Closed-loop end-of-life product life cycle[23].
Remanufacturing is defined as ‘A process that brings a used product back to a new state through reuse, refurbishment and replacement of its components’ [5]. It is a process of disassembling, cleaning, inspection and sorting, reconditioning or replacing parts (if applicable) and reassembling a used product in order to make it at least as good as, or better, than a brand new one. By applying the remanufacturing process, a product can be returned to service with a reasonably high degree of confidence that it will at least endure another full life cycle. The main purpose of remanufacturing is to prolong the product life cycle and at the same time preserve the added value of the material during the design and manufacturing phases. From the environmental point of view, remanufacturing ensures sustainable products that save energy and material resources. Remanufacturing also diverts material from landfills and creates jobs for skilled workers [6]. The decision to integrate the product remanufacturing process must be made at the earliest stages of the product life cycle [7, 8] to ensure the outcome is successful.
To better understand this field, Lund [9] defined three different types of remanufacturing company. The first type is the Original Equipment Manufacturer (OEM) which remanufactures its own products. The second type is the Subcontractor Company which remanufactures products made by other companies (usually the OEMs). The last type is the Independent Remanufacturer (IR) which remanufactures products without much contact with the OEMs. They also have to buy or collect the core components for their process. Many studies have confirmed that remanufacturing is more profitable for OEMs [10, 11].
Several studies have focused on proving the environmental benefits of remanufacturing. These studies include that of Kerr et al. [12] on the eco-efficiency gains derived from remanufacturing, based on a study of Xerox photocopiers in Australia. It attempted to quantify the overall life cycle environmental benefits of remanufacturing by comparing the remanufacturing of a traditionally designed photocopier and a photocopier designed to facilitate its own remanufacturing. In addition, Lindahl et al. [13] identified the general environmental advantages and disadvantages of remanufacturing by modelling a life cycle assessment of several households' appliances and products. An environmental comparison was performed of end-of-life scenarios (remanufacturing and recycling) for all of these appliances and products. Likewise with J. Amaya, who carried out a comparative assessment of remanufactured product life-cycles and classical life-cycle scenarios. This approach was illustrated by a case study of a truck injector [14].
Even though there have been many difficulties in defining and interpreting the system's boundaries, the measurement methods used and the result itself, this research has come to the conclusion that remanufacturing is a preferable option in comparison to new manufacturing and other end-of-life options [15].
Despite these advantages, remanufacturing lacks a tool for modelling the environmental impact of its process. Tools such as Remanufacturing Decision-Making Framework (RDMF) have been developed by researchers to promote remanufacturing in companies. It is a decision-making framework which helps management to make decisions on whether or not to remanufacture a product [7, 8]. REPRO2 [16] is a tool that allows designers to monitor the evolution of design parameters for remanufacturing [1]. The CLOEE tool [17] has been developed at the G-SCOP laboratory. This tool is designed to assess the environmental impact of multiple end-of-life scenarios. It is particularly useful when many end-of-life strategies with different options have to be tested. However, CLOEE requires a certain quantity of environmental impact data as inputs in order to test each process or scenario. These data could be obtained using the remanufacturing process library proposed in this paper.
Nowadays, there are evermore methods and technologies that complete each remanufacturing phase. This phenomenon is positive for remanufacturers because they have a large variety of options available for setting up the remanufacturing system. Moreover, current technologies allow the remanufacturing of products that could not be remanufactured before. But having more choices leads to more confusion for designers when they are not equipped with the tools they need to highlight the environmental impacts of their process.
The absence of this kind of tool always leads designers to ask about the basic rules for choosing a method/technology capable of minimizing environmental impacts. The global impact of remanufacturing on the environment is also one of the aspects that still remain uncertain. All these issues show the importance of having tools to help designers answer these questions.
From the environmental standpoint, it is difficult to optimize the remanufacturing process without knowing its impacts on the environment for the entire product life cycle. Data from studies of existing remanufacturing systems have been limited and patchy [12]. Because of these problems, it is reasonable to propose a database and a tool intended to help remanufacturers and designers to evaluate and compare the environmental impacts of their remanufacturing process during the design phase. This study is aimed at helping remanufacturers and designers to compare environmental impacts and make decisions.
In this article, a global representation of the remanufacturing process is presented. This is followed by a detailed explanation of the construction of the remanufacturing process library, the calculation of the environmental parameters, and the creation of the environmental impact simulator. In the last part, the simulator is tested in a case study on an engine block and the results are discussed before the conclusion.
Methods
Global presentation of the remanufacturing process
It is essential to have a global picture of the different processes involved in order to create a remanufacturing library. Hence, this study started with research to obtain data on the variety of methods and technologies involved in performing each remanufacturing step. Normally, these data are available online and are often obtained from remanufacturers' and suppliers' websites. Once collected, they were sorted and arranged in a readable and structured format.
A mind mapping form was chosen to represent these data. It is a simple visual tool used to help structure information and better analyse, comprehend and synthesize ideas. The mind mapping diagrams always take the same basic format of a tree, with a single starting point in the middle that branches out and divides continuously. The tree is made up of words or short sentences connected by lines.
In the mind mapping task, the data were classified by types of processes. It started with a remanufacturing process which was divided into five essential steps: disassembly, cleaning, inspection and sorting, reconditioning and reassembly. Figure 2 shows these five steps arranged in a mind mapping form. For each step, the different methods and technologies currently used were listed. Since the cleaning and repair steps are the most critical steps in the remanufacturing process [18], their methods and technologies were explored and listed until the deepest level (see Figure 3[25–28]). It is essential to list them thoroughly, because every component is a variable that can change the environmental impact and must be taken into account during the design phase.
This global presentation is an attempt to identify as many existing technologies and methods as authors can. This list should be expanded as soon as new technologies or methods appear.
Standard characterization sheet (Remanufacturing process library)
After taking a global view of the remanufacturing process, designers require detailed information about the methods and technologies listed in the previous mind map. This detailed information is important to give designers a basic idea about such methods and technologies, and help them make their choice. This study offers essential and useful information about these methods and technologies, classified in a library of available processes for remanufacturing. In particular, it includes a sheet that contains the important parameters that differentiate one process from another from the environmental standpoint.
This characterization sheet was specially designed to be used with the remanufacturing simulator, in order to compute environmental impacts. Information and parameters were synchronized between this sheet and the simulator database. This sheet contains information about the process, i.e. its name, a useful description of the process, drawings, machine technology, durations of cycles, inputs and outputs of the process, parameters to be used by the simulator for modelling the process, parameters that affect the environment and consumable materials.
The characterization sheet works like a catalogue from which designers can choose methods and technologies suitable for their product and processes. The information gathered in this sheet can generally be obtained from remanufacturers' and suppliers' websites, and designers can also choose methods and technologies based on environmental objectives. The use of the data contained in this sheet helps designers to model their remanufacturing process in the environmental simulator.
Calculation of environmental parameters
In the remanufacturing process library, the most important data to store for the creation of the remanufacturing tool are the generic parameters. Firstly, all the physical parameters of the process are listed. Then, a single physical parameter that best describes the size of the machine or process is chosen. It will be called size parameter in the following section. Then, for all the input parameters of the process, the aim is to calculate the generic parameters to be listed in the process characterization sheet.
As for the other input parameters, they are listed in correlation with the size parameter. Supplier data are used to plot a graph of input parameters as a function of the size parameter. Then the generic parameters are calculated based on a linear slope that best fits all the points plotted. These data are important because they link the remanufacturing process library and the environmental impact simulator. The size parameter is the only data that users must know in order to assess the environmental impact of the corresponding process when using the simulator. Consequently, the table providing the correspondence between the size parameter and generic parameter must be stored in the characterization sheet of the process.
The importance of the generic parameter is that it gives a generic value for one input parameter of a process. It avoids the recalculation of an input parameter every time the user changes the characteristics of the machine, such as its size. Consequently, only one value has to be keyed in for one input parameter of a process.
In this section, an example of an ultrasonic cleaning process is used to explain how the generic parameter (see Table 1) is calculated. To understand more about the function of this parameter and on the relation between the remanufacturing process library and the environmental impact simulator, a case study was performed.
The main parameters used to design one remanufacturing process can be identified from the diagram of inputs and outputs (see Figure 4) found in the remanufacturing process library. The input parameters that generate a potential environmental impact were identified, and research into the machine was carried out using the constructor's data. The environmental input parameter section in the remanufacturing process library contained the research results. The information found was used to draw curves and linear slopes, and to calculate the generic parameters later on.
Figures 5 and 6 show an example from an ultrasonic cleaning process. A table with the machine range (see Figure 5) is the constructor's data. It presents the volume as the size parameter, and the electrical power and solution weight as input parameters. In order to calculate the generic value for these input parameters, a power-vs.-volume curve was plotted using the information in the table (see Figure 6). From the curve, a power-vs.-volume slope that passes through zero and best fitted the previous points was drawn. In this case, a value of 19.23 (W/m3) was found for this parameter. This value is a value of the generic parameter for the electrical power that was keyed in as information for the environmental impact simulator database. The same steps were performed to obtain the generic parameter for the solution weight and the other processes.
Environmental impact simulator
Once the remanufacturing process information was gathered and the essential generic parameters were calculated, it was then possible to create a remanufacturing simulator. In this study, a life cycle assessment (LCA) was used as it is an accepted environmental management tool that holistically and systematically quantifies the environmental burdens and their potential impacts over the whole life cycle of a product, process or activity from a multidisciplinary standpoint [19]. The LCA is a comparative tool and is used in the comparison of different ways of achieving a defined function [20] and as a decision-making tool in process selection, design, and optimization in order to identify clean technologies.
The Simapro software was chosen as it is probably the most used at present for LCA applications and has been described and used in a large number of published works [19]. Simapro includes several LCA methods such as Eco-indicator 99, EDIP 1997 and 2003 and EPS 2000. It allows viewing parts of the life cycle at different scales, and displaying their contributions to the total score [21].
A remanufacturing function was created in Simapro under the ‘processing’ category. In this function, all the remanufacturing process data found in the remanufacturing process library were listed and keyed in. The study focused on the ‘cleaning’ and ‘reconditioning’ steps, because the other steps consumed almost no energy or consumable material. It was created from scratch for the ‘cleaning’ process, while the ‘reconditioning’ process was created directly using data available in Simapro databases. A step-by-step user guide was also created to help designers use the simulator.
Below are some important pieces of information concerning the environmental impact simulator:
-
1.
Number of processes performed: four (cleaning).
-
2.
Number of process found in Simapro: three (reconditioning).
-
3.
Some processes cannot be modelled (e.g. water jet cleaning).
-
4.
The database used was ‘Ecoinvent unit processes’, located in the European Union (indicator U/RER). The unavailable data were noted in the characterization sheet.
-
5.
The ‘Impact 2002+’ method was used to analyse the LCA results as it is universal and simple to use.
Basically, users simply have to choose the remanufacturing process, enter their product size parameters (weight, volume, etc.), choose the consumable material, enter the consumable material parameters, and change the duration of the cycle (depending on the type of product). The software performs the calculation and the results appear in a few minutes afterwards.
Case study on an engine block
A case study was carried out on a commercially remanufactured product, using the simulator to make ensure that it functioned as wished. This case study allowed detecting malfunctions of the system and the user's difficulties when they used it. It was also important in order to improve the simulator and its user guide. In this case study, a remanufacturing process was modelled for an engine block using the information found on the remanufacturer's website.
Engine block specification
Figure 7 shows the engine block used in this study. The specifications are given below:
Ford engine block[28]].
Brand: Ford
Material: Aluminium
Weight: 58 kg
Volume: 191 l (dm3)
Dimensions: 53 × 68 × 53 cm
Remanufacturing process
Once the engine block was defined, the remanufacturing process comprising more detailed information on the method and technology for each step was described using data obtained from promarengine.com.
The flowchart in Figure 8 shows a remanufacturing process for the engine chosen (see Figure 7). It can be seen that the engine block was subjected to two different inspection technologies, four cleaning methods and five reconditioning processes. These were the methods and technologies that will be entered in the environmental impact simulator using the information in the remanufacturing process library.
Remanufacturing process of an engine block[29]].
Field of study
The same methodology and phases used in the classical Life Cycle Assessment were applied to evaluate the previous remanufacturing process. According to the ISO 14040 and 14044 standards, a Life Cycle Assessment is carried out in four different phases, as listed below:
-
a)
Definition of objective and scope: In this phase, a functional unit (which defines precisely what is being studied and quantifies the service delivered by the product system) of a product or service, the system boundaries, and any assumptions and limitations are defined. Normally, information research had been carried out beforehand to gather the information required.
-
b)
Life cycle inventory: In this phase, all the inputs and outputs of a product or service are listed. The data must be related to the functional unit defined in the previous phase.
-
c)
Impact assessment: The aim of this phase is to evaluate the significance of potential environmental impacts based on the Life cycle inventory (LCI) flow results. It consists of the selection of impact categories, category indicators, and characterization models.
-
d)
Interpretation: The results from the inventory analysis and impact assessment are summarized during this phase. The outcome of the interpretation phase is a set of conclusions and recommendations for the study.
Definition of objective and scope
For this case study, the main objective was to test the environmental impact simulator in order to determine whether it performed the calculation or not. Once the results were obtained, the environmental impacts generated by several remanufacturing process scenarios were compared. Scenarios were created so that different input parameters could be filled in, making it possible to see the different impacts.
The functional unit used for this objective was the remanufacturing process of one engine block whose dimensions, weight and volume were those given in the previous section. This functional unit however cannot compare the scenario created in terms of functionality. For example, the same input weight has been used to perform the different types of material tests, even though their density and cleaning capacity were different. The geographical boundary of the system for this case study was that of the European Union. The study limitation was that only cleaning and reconditioning processes were included since it was assumed that the environmental impact of disassembly, inspection and sorting, and reassembly were negligible in comparison.
Life cycle inventory
Following the definition of the objective and scope, the life cycle inventory is provided in Table 2. The table shows the detailed process undergone by the engine block and the information keyed-in in the remanufacturing simulator. The ‘step’ column represents the process realized, while the ‘simapro’ column states the process name found in the remanufacturing simulator. The ‘quantity’ column indicates the estimated input quantity used in realizing the process. The ‘remark’ column is where the process is shortly described.
Once the result for the process in Table 2 is obtained, several tests using different scenarios were performed to determine whether the simulator was able to compare the environmental impacts. In every test that was performed, only one input was manipulated. Two remanufacturing scenarios are explained below and the results are shown in the later section.
Scenario 1: ‘Abrasive cleaning’ step: In this scenario, three different inputs, stainless steel, steel and zinc, were used as abrasive material.
Scenario 2: ‘Ultrasonic cleaning’ step: In this scenario, two different types of detergent are compared: ‘aluminium-safe powder detergent, heavy contaminant’ detergent and ‘pH controlled and low foaming liquid detergent, heavy contaminant’ detergent.
Results and discussion
Impact assessment
In this phase, the environmental impacts were assessed using the SimaPro software and the ‘impact 2002+’ [22] calculation method. The remanufacturing process library was introduced into the SimaPro process database. Figures 9 and 10 show some of the results for the different scenarios considered for the remanufacturing of the block engine.
The results showed the different environmental impacts for each scenario. The simulator performed the calculation and compared the environmental impact easily thanks to the creation of the remanufacturing process library.
Since the main objective of this case study was to test the simulator, it is not the authors' intention to interpret the results or give any recommendation concerning them. In order to do so, a further study must be carried out considering many aspects, such as the functionality, level of quality, and quantity used of each parameter chosen to carry out processes that can be compared in terms of functionality.
Conclusions and perspectives
The remanufacturing industry lacks tools to help designers choose their remanufacturing process. This study can help them to bridge this gap by providing an effective, comprehensive and simple tool that provides a global view of the remanufacturing process even in the design stage.
The generic data for a certain process was successfully synthesized in the remanufacturing process library and environmental impact simulator studies. Furthermore, the results from the engine block case study showed that the environmental impact simulator compares the remanufacturing scenarios well. With this result, this simulator could be used in the design phase as a design tool (in addition to classical design tool) in order to define the best product and remanufacturing scenario couple. It could help designers to design a remanufacturable product and its corresponding low-environmental impact remanufacturing processes.
The authors think that the outlook for improving this tool in the future is promising and that it could be used commercially. It will first be necessary to add data on different remanufacturing processes to the simulator database so that this simulator can serve the needs of designers more completely. Second, tests must be carried out to ensure that the results provided by the environmental impact simulator are useful for design activities. Such tests are important since the results of this study do not take this aspect into account.
References
Lopez Ontiveros M: Intégration des contraintes de remanufacturabilité en conception de produits. PhD Thesis. Institut National Polytechnique de Grenoble; 2004.
Ijomah WL, Bennett JP, Pearce J: Remanufacturing: evidence of environmentally conscious business practice in the UK. In Environmentally Conscious Design and Inverse Manufacturing, 1999. Proceedings. EcoDesign'99: First International Symposium On IEEE. Edited by: Yoshikawa H. Tokyo; 1999:192–196.
Lund R: Remanufacturing. In The American Edge: Leveraging Manufacturing's Hidden Assets. Edited by: Klein J, Miller J. New York: McGraw-Hill; 1993:228–229.
Steinhilper R: Remanufacturing. Stuttgart: Fraunhofer IRB Verlag; 1998.
Amezquita T, Hammond R, Salazar M, Bras B: Characterizing the remanufacturability of engineering systems. In ASME Advances in Design Automation Conference, 1995, vol. 82. Edited by: Yoshimura M, Eschenauer HA, Gilmore B. U.S.: American Society of Mechanical Engineersm; 1995:271–278.
Gray C, Charter M: Remanufacturing and product design. International Journal of Product Development 2007,6((3–4)):375–392.
Subramoniam R, Huisingh D, Chinnam R: Remanufacturing for the automotive aftermarket strategic factors: literature review and future research needs. J. Clean. Prod. 2009, 17: 1163–1174. 10.1016/j.jclepro.2009.03.004
Subramoniama R, Huisingh D, Chinnam R, Subramoniam S: Remanufacturing decision-making framework (RDMF): research validation using the analytical hierarchical process. J. Clean. Prod. 2013, 40: 212–220.
Lund R: Remanufacturing: an American perspective. Responsive Manufacturing–Green Manufacturing (ICRM 2010), 5th International Conference, 11–13 Jan. 2010 2010, 1–6.
Hammond R, Amezquita T, Bras B: Issues in the automotive parts remanufacturing industry: discussion of results from surveys performed among remanufacturers. Int. J. Eng. Des. Auto-Spec. Issue Environ. Conscious. Des. Manuf. 1998,4(1):27–46.
Guide R Jr, Daniel V: Production planning and control for remanufacturing: industry practice and research needs. J. Oper. Manag. 2000, 18: 467–483. 10.1016/S0272-6963(00)00034-6
Kerr W, Ryan C: Eco-efficiency gains from remanufacturing a case study of photocopier remanufacturing at Fuji Xerox Australia. J. Clean. Prod. 2001, 9: 75–81. 10.1016/S0959-6526(00)00032-9
Lindahl M, Sundin E, Ostlin J: Environmental issues within the remanufacturing industry. The 13th CIRP International Conference on Life Cycle Engineering 2006.
Amaya J, Zwolinski P, Brissaud D: Environmental benefits of parts remanufacturing: the truck injector case. In 17th CIRP International Conference on Life Cycle Engineering Proceedings. Edited by: Zhang H-C. Hefei, China: University of Technology Press; 2010.
Sundin E, Lee HM: In what way is remanufacturing good for the environment? In Design for Innovative Value Towards a Sustainable Society. Edited by: Matsumoto M, Umeda Y, Fukushige S. Netherlands: Springer; 2012:552–557.
Online software REPRO2 . Accessed 20 March 2013 http://repro2.inpg.fr/ang/indexa.php
Online software CLOEE . Accessed 20 March 2013 https://cloee.g-scop.grenoble-inp.fr/apps/WebObjects/CloeeApplication/
Sundin E, Bras B: Making functional sales environmentally and economically beneficial through product remanufacturing. J. Clean. Prod. 2005, 13: 913–925. 10.1016/j.jclepro.2004.04.006
Pieragostini C, Mussati M, Aguirre P: On process optimization considering LCA methodology. J. Environ. Manag. 2012, 96: 43–54. 10.1016/j.jenvman.2011.10.014
Olsen S, Christensen F, Hauschild M, Pedersen F, Larsen H, Torslov J: Life cycle impact assessment and risk assessment of chemicals—a methodological comparison. Environ. Impact Assess. Rev. 2001, 21: 385–404. 10.1016/S0195-9255(01)00075-0
Simapro database manual . Accessed 5 April 2013 http://www.pre-sustainability.com/download/manuals/DatabaseManualMethods.pdf
Life cycle impact assessment methodology: Impact 2002+ user guide . Accessed 3 August 2013 http://www.quantis-intl.com/pdf/IMPACT2002_UserGuide_for_vQ2.21.pdf
Amaya Rivas J: Evaluation des bénéfices environnementaux liés à des cycles de vie de produits en flux bouclés. PhD Thesis. Université de Grenoble; 2012.
Supplier’s website for ultrasonic cleaning chemical . Accessed 3 January 2013 http://www.ultrawave.co.uk
Supplier’s website for abrasive cleaning media . Accessed 3 January 2013 http://www.kramerindustriesonline.com
Suppliers website for abrasive cleaning media . Accessed 3 January 2013 http://www.coldjet.com
Suppliers website for abrasive cleaning media . Accessed 3 January 2013 http://www.surfacepreparation.com
Photo of Ford engine block . Accessed 3 October 2013 http://www.autoblog.com/2009/06/13/ford-racing-introduces-new-boss-351-engine-block/
Remanufacturer’s website for a remanufacturing process of an engine block . Accessed 3 October 2013 http://www.promarengine.com/engines.asp
Acknowledgements
Nurul Hanna Ismail sincerely appreciates the G-Scop Laboratory for the financial support during this Master thesis period.
Author information
Authors and Affiliations
Corresponding author
Additional information
Competing interests
The authors declare that they have no competing interests.
Authors’ contributions
PZ defined the research aim and identified the problem. NHI collected data, carried out the literature review, conducted technical and environmental analyses and finally completed this manuscript. GM and PZ reviewed the technical and environmental analyses, participated in the discussion of the results and corrected the manuscript. All the authors read and approved the final manuscript.
Authors’ original submitted files for images
Below are the links to the authors’ original submitted files for images.
Rights and permissions
Open Access This article is distributed under the terms of the Creative Commons Attribution 2.0 International License (https://creativecommons.org/licenses/by/2.0), which permits unrestricted use, distribution, and reproduction in any medium, provided the original work is properly cited.
About this article
Cite this article
Ismail, N.H., Mandil, G. & Zwolinski, P. A remanufacturing process library for environmental impact simulations. Jnl Remanufactur 4, 2 (2014). https://doi.org/10.1186/2210-4690-4-2
Received:
Accepted:
Published:
DOI: https://doi.org/10.1186/2210-4690-4-2