Abstract
Backgound
The effects of extremely low-frequency electromagnetic fields (ELF-EMF) on the blood serum and liver lipid concentrations of male Wistar rats were assessed.
Methods
Animals were exposed to a single stimulation (2 h) of ELF-EMF (60 Hz, 2.4 mT) or sham-stimulated and thereafter sacrificed at different times (24, 48 or 96 h after beginning the exposure).
Results
Blood lipids showed, at 48 h stimulated animals, a significant increase of cholesterol associated to high density lipoproteins (HDL-C) than those observed at any other studied time. Free fatty acid serum presented at 24 h significant increases in comparison with control group. The other serum lipids, triacylglycerols and total cholesterol did not show differences between groups, at any time evaluated. No statistical differences were shown on total lipids of the liver but total cholesterol was elevated at 24 h with a significant decrease at 96 h (p = 0.026). The ELF-EMF stimulation increased the liver content of lipoperoxides at 24 h.
Conclusion
Single exposures to ELF-EMF increases the serum values of HDL-C, the liver content of lipoperoxides and decreases total cholesterol of the liver. The mechanisms for the effects of ELF-EMF on lipid metabolism are not well understand yet, but could be associated to the nitric oxide synthase EMF-stimulation.
Similar content being viewed by others
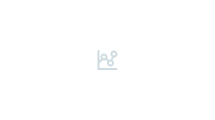
Background
Some recent epidemiologic studies have suggested that the exposure to extremely low frequency (ELF) electromagnetic fields (EMF) affect human health, because of the incidence of certain types of cancer, depression, and miscarriage have been increased among individuals living or working in environments exposed to such fields [1–3]. Some of these studies have shown associations between exposure to power-frequency (50–60 Hz) magnetic fields and increased health risk [4, 5], but other studies have not shown such a link [6]. The results described above are not completely conclusive, since in several cases they are contradictory.
Extremely low-frequency electromagnetic fields exposure is generally believed to be innocuous for human health due to their low-level energy exposition, which is of a magnitude well below that required to affect the metabolic rate of the human body [3, 7, 8]. However, an increasing number of studies have reported that ELF-EMF exposure is capable to eliciting in vivo and in vitro bioeffects [9–12]. ELF-EMF exposure has shown to increase oxidative-stress in some models like chick embryos [13], mammalian cultured cells [14], and human erythrocytes [15]. The increased oxidative-stress involves oxidative DNA damage, lipid peroxidation [16], and may cause a number of systemic disturbances [17].
Spreading evidence suggests that environmental and artificial magnetic fields have significant impact on cardiovascular systems of animals and humans [18–21]. Recent studies showed that static magnetic fields decreased arterial baroreflex sensitivity in rabbits [22]. Under pharmacologically induced hypertensive conditions, the exposure to nonuniform MF to rabbits, significantly attenuated the vasoconstriction and suppressed the elevation of blood pressure [23]. In contrast, microwaves increase skin temperature and therefore cause vasodilatation in normal subjects exposed during 30 minutes [24]. Also, human blood platelets exposed in vitro to microwaves produced by mobile phones (operating at 900 MHz), increased the thiobarbituric acid reactive substances (TBARS) production and significantly depleted the superoxide dismutase-1 activity [17]. Recently, it has been studied the possibility that heat generation and the activation of the inducible form of nitric oxide (NO) synthase may be the possible causes of the biological effects of EMF exposure [25–27].
On the other hand, beneficial effects of ELF-EMF have also been reported. In diet-induced hypercholesterolemic rabbits, pulsed of EMF lowers total cholesterol and triacylglycerols levels [28]; similar results have been found in rats [29] and mice [30], both fed on control diets. Despite the above mentioned studies, there are not enough data to know the time-course effects of ELF-EMF on lipid and lipoperoxide levels in biological models. Then, the aim of the present study was to investigate, in the rat, the time-course effects of a single ELF-EMF stimulation on serum and liver lipid concentrations, as well as liver lipoperoxide production.
Results
Serum lipid concentrations
There were no significant differences between experimental and control groups for triacylglycerols and total cholesterol serum levels, at any time analyzed. However, 48 h after beginning the treatment, HDL-C concentrations were higher in stimulated rats (48.2 ± 4.3 mg/dL) than those observed in control group (38.7 ± 7.1 mg/dL); however, a significant decrease on HDL-C levels was observed at 96 h in ELF-EMF stimulated rats (24 h 46.1 ± 8.1 mg/dL and 96 h 29.24 ± 2.20 mg/dL) (Fig. 1). On the other hand, FFA serum levels increased at 24 h (20 ± 2.25 mg/dL) with a statistically significant difference respect control (16.6 ± 3 mg/dL; p = 0.026) but no differences were found at 48 or 96 h (Fig. 2).
Liver analyses
There were no significant differences, in total lipids, triacylglycerols and protein values at any time (24, 48 and 96 h, data not shown). Liver total cholesterol showed a transient increase level at 24 h (48.6 ± 8.3 mg/dL) that decreased at 96 h (32.8 ± 6.1 mg/dL; p = 0.026) (Fig. 3). Furthermore, liver TBARS concentration was the variable where the ELF-EMF exposure induced major increases 24 h after beginning the treatment (198.80 ± 56.9 vs. control group 102.18 ± 27.8 ng/mg total lipids) meanwhile no significant differences were found at 48 or 96 h (Fig. 4).
Discussion
Extremely low frequency electromagnetic fields interact with an animal by inducing electric fields inside the body. These induced fields represent the internal exposure or "dose" [31]. In living animals, a variety of natural endogenous electric fields also exist internally. These fields arise from normal physiological activity, and extend into adjacent tissues throughout the body. The endogenous fields will combine by simple addition with any field induced by external exposure to electromagnetic fields.
Some studies about biological effects due to 50–60 Hz electromagnetic fields exposure have been performed with rodents and other animal models [32, 33]. Assessment of possible human health effects has been to a certain extent supports on these studies. It has been recognized that induced electric field in rodents are much lower than in humans for the same exposure field [34], this is a direct result of differences in size [35].
Since in situ measurement of induced field cannot be performed in humans, dosimetric models and animal studies are used. Comparisons of all dosimetric models are agreed within 2% or less; however, differences dues of size, shape, postural and individual organ have been observed [36].
In the present study, we use the rodent model to test the time-course effects of a single exposure to ELF-EMF (60 Hz, 2.4 mT, by two hours) on serum and liver lipids, as well as liver lipoperoxide content. The whole body exposure approach could stimulate any tissue; however, among the most susceptible tissues to EMF exposure are brain, blood and liver [37]. No changes were observed in total cholesterol and triacylglycerol serum levels. But HDL-C and free fatty acids, were higher at 48 and 24 hours, respectively, in stimulated group than in sham-stimulated; however, only HDL-C showed lower levels at 96 hours after beginning the exposure. Our results are partially in accordance with previous studies showing that a single one hour exposure to a 12 Hz, 1.5–12 mT pulsed magnetic fields decreased, in a reversible way, cholesterol and triacylglycerol plasma levels in male rats 24 hours after their exposure [29, 38]. Furthermore, when hyperlipidemic diet-induced female rabbits were exposed to a 15 Hz, 0.4 mT, 10 hours/day by 8 weeks, both cholesterol and triacylglycerols were significantly decreased, while HDL-C values were increased [28]. Harakawa and colleagues [37] observed that the exposition for 14 days to ELF-electric fields of ischemic rats significantly decreased free fatty acids and triacyglycerol plasma levels. The fact that total cholesterol and triacylglycerol serum levels did not change in our study could be explained in part by the frequency and length of stimulation used, as well as the basal lipemia of animals. Because of changes in serum free fatty acid concentration under our experimental conditions were observed at 24 h, it can be proposed that ELF-EMF have early effects on adipocytes lipolysis, at least after a single EMF stimulation.
On the other hand, TBARS levels were also increased in experimental group at 24 hours after the exposure. Because of the nitric oxide (NO) synthase may be stimulated by the EMF exposure [25–27], and this compound has been involved in the stimulation of lipid peroxidation during the initial states of ischemia-reperfusion injury [39], it is possible that increased free fatty acid deposition in the liver after ELF-EMF could occur as a consequence of the NO production. This hypothesis could explain also the increased TBARS production in stimulated group.
Lipid peroxidation products have been accepted as biomarkers for oxidative stress in biological systems [40]. Furthermore, few studies have been focused on the involvement of oxidative stress in the action mechanism of EMF exposure [41, 42]. Some authors have showed that exposition to electric fields (50 Hz) of Sprague-Dawley rats did not change the antioxidant activity and lipid peroxide level of unstressed animals but decreased the plasma peroxide level in stressed rats [43]. The authors suggest that the electric fields might have some influence on lipid peroxide metabolism. In the present study we also obtained changes in liver peroxide level when Wistar rats were exposed to electromagnetic fields. Recently, Zwirska-Korczala and colleagues [44] using preadipocytes, showed a diminution in the activity of superoxide dismutase after 24 and 48 h of EMF exposition (180–195 Hz). The early increase in the liver TBARS concentration observed in our study after 24 h of EMF stimulation could be the result of the reduction in the activity of antioxidant enzymes or/and the increase of free radical production.
No differences were observed in cholesterol content of the liver between both groups studied. This result contrast with those finding in other studies [28, 29], but could be explained by the different stimulation conditions used in that studies.
On the other hand, reduction in serum total lipids has also been observed in human beings. The most pronounced changes were found in steelworkers with the longest exposure (over 10 years) to electromagnetic fields [45].
Conclusion
In conclusion, the results described here demonstrate the adaptative temporary response on lipid metabolism after the single exposure to ELF-EMF. The increased TBARS level after a single exposure to ELF-EMF deserves more research in other ELF-EMF conditions. Although the mechanisms for the effects of ELF-EMF are not well understand yet, the effects described above could be useful in the comprehension of lipid changes observed during chronic exposure to ELF-EMF.
Methods
Reagents
All reagents and chemicals used were of analytical grade (Sigma-Aldrich, México). Solvents were purchased from Merck (México). Total cholesterol (TC), high-density lipoprotein cholesterol (HDL-C), triacylglycerols (TAG), and free fatty acids (FFA) were assessed by enzymatic kits (Roche, México).
Animals
Forty male rats of the Wistar strain, weighing 220–250 g were used. All animals were housed per group (five animals per acrylic cage) with free access to food and water, in a room with controlled temperature (25 ± 2°C) and light-dark cycles (07:00 – 19:00 h, light on). All experiments were conducted during the light phase of the cycle, between 09:00 and 15:00 h.
All procedures were performed in strict accordance with the international guidelines for care of experimental animals.
The rats were either exposed to a single stimulation of ELF-EMF (experimental groups) or sham-stimulated (control groups). The time-course effects of the single ELF-EMF exposure on serum and liver lipid concentrations, as well as liver lipoperoxides (measured as TBARS) were assessed at 24, 48 and 96 h after beginning the treatment (five rats per group at each time).
ELF-EMF exposure
Electromagnetic field exposure was applied with a device used previously in our laboratory [46]. Electromagnetic fields were generated inside the exposure chamber with a pair of circular Helmholtz coils (30 cm internal diameter) composed of 18-gauge copper wire (350 turns). The two coils were connected in parallel to minimize the total impedance of the wire and to map the magnetic field. Coils were connected to a 120 V adjustable transformer (Staco Energy Products, Dayton, OH, USA). An oscilloscope (Tektronix, 5103N, USA) was coupled to the system for monitoring the 60 Hz sinusoidal MF waveform. Magnetic flux density was measured using a hand-held Gauss/Tesla Meter (Alpha-Lab). The sinusoidal magnetic flux density was 2.4 mT. Helmholtz coils provide a very uniform field over a relatively large volume in the space between the coils.
Coils were spaced apart at a distance equal to their radii in the upper and lower face of the plastic exposure chamber (30 × 30 × 15 cm). A single stimulation was applied during 2 h from 09:00 to 11:00 h. The exposure chamber housed five rats each experimental session. Sham-stimulated animals were maintained simultaneously to experimental animals for an equal period of time inside of another chamber with the coils turned off. The magnetic field ambient background level was <0.04 mT. Inside the exposure chamber the temperature was 25.4 ± 0.4°C and illumination intensity was 17 ± 2 Lux.
Serum analyses
The animals were fasted twelve hours previously to the end of experimental period. The rats were anesthetized with diethyl ether and killed by cervical dislocation. The serum was obtained by blood centrifugation and stored at -78°C until triacylglycerols, total cholesterol and free fatty acids were assessed. HDL-C was determined using aliquots of fresh serum.
Liver analyses
The liver was excised, weighed and stored at -78°C. For each liver, a sample of fresh tissue was obtained for lipid analyses.
Total lipids were extracted with chloroform-methanol (3:1 v/v) by a modified Folch's method [47]. For liver samples, 1.0 g of fresh tissue was homogenized in 4 volumes of 0.05 M phosphate buffer, pH 7.2 containing 0.025% butylated hydroxytoluene (BHT), as antioxidant. Then, the pH was adjusted to 6.0 by the addition of HCl solution and this suspension was extracted three times with 3 volumes each of the chloroform-methanol mixture. The extract was washed with 10 mL of water, the organic fraction was evaporated under a nitrogen stream, then weighed (for total lipids), and stored at -78°C until cholesterol, triacylglycerol, and TBARS analyses were performed. Liver lipoperoxides were measured by determining TBARS as previously described [48]. Total protein content was determinated in homogenate aliquots, using Bradford's method [49].
Statistical analyses
Results were evaluated by one way analysis of variance (ANOVA) except those from serum and liver triacylglycerols (Kolmogorov-Smirnov). Differences among groups were assessed by Tukey and Mann Withney-U tests, using SPSS software v. 12. A statistical p value less than 0.05 was considered significant.
References
Lacy-Hubert A, Metcalfe JC, Hesketh R: Biological responses to electromagnetic fields. FASEB J. 1998, 12: 395-420.
Ahlbom A, Feychting M: Electromagnetic radiation. British Medical Bull. 2003, 68: 157-165. 10.1093/bmb/ldg030. 10.1093/bmb/ldg030
Feychting M, Ahlbom A, Kheifets L: EMF and health. Annu Rev Public Health. 2005, 26: 165-189. 10.1146/annurev.publhealth.26.021304.144445
Valberg PA, Kavet R, Rafferty CN: Can low-level 50/60 Hz electric and magnetic fields cause biological effects?. Radiation Research. 1997, 148: 2-21. 10.2307/3579533
Wertheimer N, Savitz DA, Leeper E: Childhood cancer in relation to indicators of magnetic fields from ground current sources. Bioelectromagnetics. 1995, 16 (2): 86-96. 10.1002/bem.2250160204
Preece AW, Hand JW, Clarke RN, Stewart A: Power frequency electromagnetic fields and health. Where's the evidence?. Phys Med Biol. 2000, 45: 139-154. 10.1088/0031-9155/45/9/201. 10.1088/0031-9155/45/9/201
Adey WR: Tissue interactions with nonionizing electromagnetic fields. Physiol Rev. 1981, 61 (2): 435-514.
Habash RW, Brodsky LM, Leiss W, Krewski D, Repacholi M: Health risks of electromagnetic fields. Part I: Evaluation and assessment of electric and magnetic fields. Crit Rev Biomed Eng. 2003, 31 (3): 141-195. 10.1615/CritRevBiomedEng.v31.i3.10
Litovitz TA, Montrose CJ, Wang W: Dose-response implications of the transient nature of electromagnetic-field-induced bioeffects: theoretical hypotheses and predictions. Bioelectromagnetics. 1992, 237-246. Suppl 1.
Repacholi MH, Greenebaum B: Interaction of static and extremely low frequency electric and magnetic fields with living systems: health effects and research needs. Bioelectromagnetics. 1999, 20: 133-160. 10.1002/(SICI)1521-186X(1999)20:3<133::AID-BEM1>3.0.CO;2-O
Foster KR: Mechanisms of interaction of extremely low frequency electric fields and biological systems. Radiat Prot Dosimetry. 2003, 106 (4): 301-310.
Juutilainen J: Developmental effects of extremely low frequency electric and magnetic fields. Radiat Prot Dosimetry. 2003, 106 (4): 385-390.
Di Carlo AL, White NC, Litovitz TA: Mechanical and electromagnetic induction of protection against oxidative stress. Bioelectrochemistry. 2000, 53: 87-95. 10.1016/S0302-4598(00)00116-1.
Hook GJ, Spitz DR, Sim JE, Higashikubo R, Baty JD, Moros EG, Roti JL: Evaluation of parameters of oxidative stress after in vitro exposure to FMCW and CDMA modulated radio frequency radiation fields. Radiat Res. 2004, 162 (5): 497-504. 10.1667/RR3251
Dachà M, Accorsi A, Pierotti C, Vetrano F, Mantovani R, Guidi G, Conti R, Nicolini P: Studies on the possible biological effects of 50 Hz electric and/or magnetic fields: evaluation of some glycolitic enzymes, glycolitic flux, energy and oxido-reductive potentials in human erythrocytes exposed in vitro to power frequency fields. Bioelectromagnetics. 1993, 14 (4): 383-391. 10.1002/bem.2250140410
Yokus B, Cakir DU, Akdag MZ, Sert C, Mete N: Oxidative DNA damage in rats exposed to extremely low frequency electromagnetic fields. Free Radic Res. 2005, 39 (3): 317-323. 10.1080/10715760500043603
Stopezyk D, Gnitecki W, Buczynski A, Markuszewski A, Buczynski J: Effect of electromagnetic field produced by mobile phones on the activity of superoxide (SOD-1) and the level of malonyldialdehyde (MDA) in vitro study. Med Pr. 2002, 53 (4): 311-314.
Sastre A, Graham C, Cook MR: Brain frequency magnetic fields alter cardiac autonomic control mechanisms. Clin Neurophysiol. 2000, 111 (11): 1942-1948. 10.1016/S1388-2457(00)00438-7
Szmigielski S, Bortkiewicz A, Gadzicka E, Zmyslony M, Kubacki R: Alteration of diurnal rhythms of blood pressure and heart rate to workers exposed to radiofrequency electromagnetic fields. Blood Press Monit. 1998, 3 (6): 323-330.
Graham C, Sastre A, Cook MR, Kavet R: Heart rate variability and physiological arousal in men exposed to 60 Hz magnetic fields. Bioelectromagnetics. 2000, 21 (6): 480-482. 10.1002/1521-186X(200009)21:6<480::AID-BEM8>3.0.CO;2-J
Jauchem JR, Frei MR, Ryan KL, Merritt JH, Murphy MR: Lack of effects on heart rate and blood pressure in ketamine-anesthetized rats briefly exposed to ultra-wideband electromagnetic pulses. IEEE Trans Biomed Eng. 1999, 46 (1): 117-120. 10.1109/10.736767
Gmitrov J, Ohkubo C: Artificial static and geomagnetic field interrelated impact on cardiovascular regulation. Bioelectromagnetics. 2002, 23 (5): 329-338. 10.1002/bem.10020
Okano H, Ohkubo C: Anti-pressor effects of whole body exposure to static magnetic field on pharmacologically induced hypertension in conscious rabbits. Bioelectromagnetics. 2003, 24 (2): 139-147. 10.1002/bem.10092
Paredi P, Kharitonov SA, Hanazawa T, Barnes PJ: Local vasodilator response in mobile phones. Laryngoscope. 2001, 111 (1): 159-162. 10.1097/00005537-200101000-00027
Kavaliers M, Choleris E, Prato FS, Ossenkopp KP: Evidence for the involvement of nitric oxide and nitric oxide synthase in the modulation of opiod-induced antinociception and the inhibitory effects of exposure to 60-Hz magnetic fields in the land snail. Brain Res. 1998, 809 (1): 50-57. 10.1016/S0006-8993(98)00844-0
Kim SS, Shin HJ, Eom DW, Huh JR, Woo Y, Kim H, Ryu SH, Suh PG, Kim MJ, Kim JY, Koo TW, Cho YH, Chung SM: Enhanced expression of neuronal nitic oxide synthase and phospholipase C-gamma1 in regenerating murine neuronal cells by pulse electromagnetic field. Esp Mol Med. 2002, 34 (1): 53-59.
Seaman RL, Parker JE, Kiel JL, Mathur SP, Grubbs TR, Prol HK: Ultra-wideband pulses increase nitric oxide production by RAW 264.7 macrophages incubated in nitrate. Bioelectromagnetics. 2002, 23 (1): 83-87. 10.1002/bem.100
Luo EP, Jiao LC, Shen GH, Wu XM, Cao YX: Effects of exposing rabbits to low-intensity pulsed electromagnetic fields on levels of blood lipid and properties of hemorheology. Chinese J Clin Rehabilitation. 2004, 8 (18): 3670-3671.
Bellossi A, Pouvreau-Quillien V, Rocher C, Ruelloux M: Effect of pulsed magnetic fields on triglyceride and cholesterol levels in plasma of rats. Panminerva Med. 1998, 40 (4): 276-279.
Kumosani TA, Qari MH: The effect of magnetic field on the biochemical parameters of mice blood. Pak J Med Sci. 2003, 19 (1): 36-40.
Miller DL, Creim JA: Comparison of cardiac and 60 Hz magnetically induced electric fields measured in anesthetized rats. Bioelectromagnetics. 1997, 18: 317-323. 10.1002/(SICI)1521-186X(1997)18:4<317::AID-BEM4>3.0.CO;2-5
Babbit JT, Kharazi AI, Taylor JM, Boods CB, Mirell SG, Frumkin E, Zhuang D, Hahn TJ: Hematopoietic neoplasia in C57BL/6 mice exposed to split-dose ionizing radiation and circularly polarized 60 Hz magnetic fields. Carcinogenesis. 2000, 21: 1379-1389. 10.1093/carcin/21.7.1379
Morris JE, Sasser LB, Miller DL, Dagle GE, Rafferty CN, Ebi KL, Anderson LE: Clinical progression of transplanted large granular lymphocytic leukemia in Fischer 344 rats exposed to 60 Hz magnetic fields. Bioelectromagnetics. 1999, 20: 48-56. 10.1002/(SICI)1521-186X(1999)20:1<48::AID-BEM7>3.0.CO;2-8
, : Assessment of health effects from exposure to power-line frequency electric and magnetic fields. NIH Publication No. 98. 1998.
Dawson TW, Caputa K, Stuchly MA: Electric fields induced in humans and rodents by 60 Hz magnetic fields. Phys Med Biol. 2002, 47: 2561-2568. 10.1088/0031-9155/47/14/313
Caputa K, Dimbylow PJ, Dawson TW, Stuchly MA: Modelling fields induced in humans by 50/60 Hz magnetic fields: reliability of the results and effects of model variations. Phys Med Biol. 2002, 47: 1391-1398. 10.1088/0031-9155/47/8/311
Harakawa S, Inoue N, Hori T, Tochio K, Kariya T, Kunihito Takahashi K, Doge F, Martin ED, Saito A, Suzuki H, Nagasawa H: Effects of exposure to a 50 Hz electric field on plasma levels of lactate, glucose, free fatty acids, triglycerides and creatine phosphokinase activity in hind-limb ischemic rats. J Vet Med Sci. 2005, 67: 969-974. 10.1292/jvms.67.969
Bellossi A, Pouvreau-Quillien V, Rocher C, Ruelloux M: Effect of pulsed magnetic fields on cholesterol and triglyceride levels in rats study of field intensity and length of exposure. Z Naturforsch. 1996, 51 (7–8): 603-606.
Jaeschke H: Molecular mechanism of hepatic ischemia-reperfusion injury and preconditioning. Am J Physiol Gastrointest Liver Physiol. 2003, 284: G15-G26.
Laval J: Role of DNA repair enzymes in the cellular resistence to oxidative stress. Pathol Biol. 1996, 44 (11): 14-24.
Moustafa YM, Moustafa RM, Belacy A, Abou-El-Ela SH, Ali FM: Effects of acute exposure to the radiofrequency fields of cellular phones on plasma lipid peroxide and antioxidase activities in human erythrocytes. J Pharm Biomed Anal. 2001, 26 (4): 605-608. 10.1016/S0731-7085(01)00492-7
Simko M, Droste S, Kriehuber R, Weiss DG: Stimulation of phagocytosis and free radical production in murine macrophages by 50 Hz electromagnetic fields. Eur J Cell Biol. 2001, 80 (8): 562-566. 10.1078/0171-9335-00187
Harakawa S, Inoue N, Hori T, Tochio K, Kariya T, Takahashi K, Doge F, Suzuki H, Nagasawa H: Effects of a 50 Hz electric field on plasma lipid peroxide level and antioxidant activity in rats. Bioelectromagnetics. 2005, 26: 589-594. 10.1002/bem.20137
Zwirska-Korczala K, Jochem J, Adamcczyk-Sowa M, Sowa P, Polaniak R, Birkner C, Latocha M, Pilc K, Suchanek R: Effect of extremely low frequency electromagnetic fields on cell proliferation, antioxidative enzyme activities and lipid peroxidation in 3T3-L1 preadipocytes-an in vitro study. J Physiol Pharmacol. 2005, 56 (Supp 6): 101-108.
Kula B, Sobczak A, Grabowska-Bochenek R, Piskorska D: Effect of electromagnetic field on serum biochemical parameters in steelworkers. J Occup Health. 1999, 41: 177-180.
Vázquez-García M, Elías-Viñas D, Reyes-Guerrero G, Domínguez-González A, Verdugo-Díaz L, Guevara-Guzmán R: Exposure to extremely low-frequency electromagnetic fields improves social recognition in male rats. Physiol Behav. 2004, 82 (4): 685-690. 10.1016/j.physbeh.2004.06.004
Torres-Durán PV, Miranda-Zamora R, Paredes-Carbajal MC, Mascher D, Ble-Castillo J, Díaz-Zagoya JC, Juárez-Oropeza MA: Studies on the preventive effect of Spirulina maxima on fatty liver development induced by carbon tetrachloride, in the rat. J Ethnopharmacol. 1999, 64: 141-147. 10.1016/S0378-8741(98)00120-2
Torres-Durán PV, Paredes-Carbajal MC, Mascher D, Zamora-González J, Díaz-Zagoya JC, Juárez-Oropeza MA: Protective effect of Arthrospira maxima on fatty acid composition in fatty liver. Arch Med Res. 2006, 37: 479-483. 10.1016/j.arcmed.2005.08.005
Bradford MM: A rapid and sensitive method for the quantitation of microgram quantities of protein utilizing the principle of protein-dye binding. Anal Biochem. 1976, 72: 248-254. 10.1016/0003-2697(76)90527-3
Acknowledgements
This work was supported in part by a grant from PAPIIT-UNAM (IN218107, MAJO).
Author information
Authors and Affiliations
Corresponding author
Additional information
Competing interests
The author(s) declare that they have no competing interests.
Authors' contributions
PVTD participated in the collection, design, analysis and interpretation of data and writing of the manuscript; AFH participated in the collection and analysis of data and performed the statistical analysis; MAJO participated in the design, analysis and interpretation of data and writing of the manuscript; DEV participated in the design of equipment; LVD participated in the analysis and interpretation of data and writing of the manuscript. All authors read and approved the final manuscript.
Authors’ original submitted files for images
Below are the links to the authors’ original submitted files for images.
Rights and permissions
Open Access This article is published under license to BioMed Central Ltd. This is an Open Access article is distributed under the terms of the Creative Commons Attribution License ( https://creativecommons.org/licenses/by/2.0 ), which permits unrestricted use, distribution, and reproduction in any medium, provided the original work is properly cited.
About this article
Cite this article
Torres-Duran, P.V., Ferreira-Hermosillo, A., Juarez-Oropeza, M.A. et al. Effects of whole body exposure to extremely low frequency electromagnetic fields (ELF-EMF) on serum and liver lipid levels, in the rat. Lipids Health Dis 6, 31 (2007). https://doi.org/10.1186/1476-511X-6-31
Received:
Accepted:
Published:
DOI: https://doi.org/10.1186/1476-511X-6-31