Abstract
The influence of the binding of orthosteric ligands on the conformational dynamics of the β-2-adrenoreceptor was identified using the molecular dynamics method. It was found that there was a small fraction of active states of the receptor in its apo (ligand free) ensemble. An analysis of the MD trajectories indicated that this spontaneous activation of the receptor was accompanied by the motion of its VI helix. Thus, the receptor’s constitutive activity is a direct result of its conformational dynamics. On the other hand, the binding of the full agonist resulted in a significant shift in the initial equilibrium towards its active state. Finally, the binding of the inverse agonist stabilized receptor in its inactive state. It is likely that the binding of the inverse agonists might be a universal method of the constitutive activity inhibition. Our results indicate that ligand binding redistributes preexisting conformational degrees of freedom (in accordance to the Monod-Wyman-Changeux Model), rather than causes an induced fit. Therefore, the ensemble of the biologically relevant receptors conformations has been encoded in its spatial structure and individual conformations from that ensemble and might be used by the cell according to the physiological behavior.
Access this article
We’re sorry, something doesn't seem to be working properly.
Please try refreshing the page. If that doesn't work, please contact support so we can address the problem.
Similar content being viewed by others
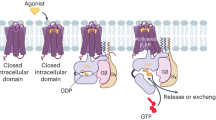
Abbreviations
- MD:
-
molecular dynamics
- EDS:
-
essential dynamics sampling
- GPCR:
-
G-protein coupled receptors
- 7-TM:
-
7-transmembrane
- B2AR:
-
β-2-adrenoreceptor
- TMD:
-
transmembrane domain
- PDB:
-
protein data bank
- SD:
-
standard deviation
- PCA:
-
principal componential analysis
- PC:
-
principal component
References
Ishima R., Torchia D.A. 2000. Protein dynamics from NMR. Nature Struct. Biol. 1, 740–743.
Congreve M., Marshall F. 2010. The impact of GPCR structures on pharmacology and structure-based drug design. Br. J. Pharmacol. 159, 986–996.
Bosier B., Hermans E. 2007. Versatility of GPCR recognition by drugs: from biological implications to therapeutic relevance. Trends Pharmacol. Sci. 28, 438–446.
Costa T., Cotecchia S. 2005. Historical review: Negative efficacy and the constitutive activity of G-protein-coupled receptors. Trends Pharmacol. Sci. 26, 618–624.
Deupi X., Standfuss J. 2011. Structural insights into agonist-induced activation of G-protein-coupled receptors. Curr. Opin. Struct. Biol. 21, 541–545.
Boehr D.D., Nussinov R., Wright P.E. 2009. The role of dynamic conformational ensembles in biomolecular recognition. Nature Chem. Biol. 5, 789–796.
Vaidehi N., Kenakin T. 2010. The role of conformational ensembles of seven transmembrane receptors in functional selectivity. Curr. Opin. Pharmacol. 10, 775–781.
Canals M, Lane J.R., Wen A., Scammells P.J., Sexton P.M., Christopoulos A. 2012. A Monod-Wyman-Changeux mechanism can explain G protein-coupled receptor (GPCR) allosteric modulation. J. Biol. Chem. 287, 650–659.
Kenakin T. 2002. Efficacy at G-protein-coupled receptors. Nature Rev. Drug Discov. 1, 103–110.
Kenakin T.P. 2009. 7TM receptor allostery: Putting numbers to shapeshifting proteins. Trends Pharmacol. Sci. 30, 460–469.
Klepeis J.L., Lindorff-Larsen K., Dror R.O., Shaw D.E. 2009. Long-timescale molecular dynamics simulations of protein structure and function. Curr. Opin. Struct. Biol. 19, 120–127.
Johnston J. M., Filizola M. 2011. Showcasing modern molecular dynamics simulations of membrane proteins through G protein-coupled receptors. Curr. Opin. Struct. Biol. 21, 552–558.
Durrant J.D., McCammon J.A. 2011. Molecular dynamics simulations and drug discovery. BMC Biol. 9, 71.
Johnson J., Liggett S. 2011. Cardiovascular pharmacogenomics of adrenergic receptor signaling: Clinical implications and future directions. Clin. Pharmacol. Therap. 89, 366–378.
Litonjua A.A. 2006. The significance of β2-adrenergic receptor polymorphisms in asthma. Curr. Opin. Pulm. Med. 12, 12–17.
Ma S., Dai Y. 2011. Principal component analysis-based methods in bioinformatics studies. Brief Bioinform. 12, 714–722
Hayward S., Kitao A., Go N. 1995. Harmonicity and anharmonicity in protein dynamics: A normal mode analysis and principal component analysis. Proteins. 23, 177–186.
Swaminath G., Deupi X., Lee T.W., Zhu W., Thian F.S., Kobilka T.S., Kobilka B. 2005. Probing the p2 adrenoceptor binding site with catechol reveals differences in binding and activation by agonists and partial agonists. J. Biol Chem. 280, 221–225.
Amadei A., Linssen A., De Groot B., Van Aalten D., Berendsen H. 1996. An efficient method for sampling the essential subspace of proteins. J. Biomol. Struct. Dyn. 13, 615–625.
Snow C., Qi G., Hayward S. 2007. Essential dynamics sampling study of adenylate kinase: Comparison to citrate synthase and implication for the hinge and shear mechanisms of domain motions. Proteins: Struct. Funct. Bioinform. 67, 325–337.
Daidone I., Amadei A., Roccatano D., Nola A.D. 2003. Molecular dynamics simulation of protein folding by essential dynamics sampling: Folding landscape of horse heart cytochrome c. Biophys. J. 85, 2865–2871.
Novikov G., Sivozhelezov V., Shaitan K. 2013. Study of structural dynamics of ligand-activated membrane receptors by means of principal component analysis. Biochemistry (Moscow). 78, 403–411.
Kobilka B.K., Deupi X. 2007. Conformational complexity of G-protein-coupled receptors. Trends Pharmacol. Sci. 28, 397–406.
Deupi X., Kobilka B.K. 2010. Energy landscapes as a tool to integrate GPCR structure, dynamics, and function. Physiology (Bethesda). 25, 293–303.
Bond R.A., IJzerman A.P. 2006. Recent developments in constitutive receptor activity and inverse agonism, and their potential for GPCR drug discovery. Trends Pharmacol. Sci. 27, 92–96.
Smit M.J., Vischer H.F., Bakker R.A., Jongejan A., Timmerman H., Pardo L., Leurs R. 2007. Pharmacogenomic and structural analysis of constitutive g protein-coupled receptor activity. Ann. Rev. Pharmacol Toxicol. 47, 53–87.
Violin J.D., DiPilato L.M., Yildirim N., Elston T.C., Zhang J., Lefkowitz R.J. 2008. β2-Adrenergic receptor signaling and desensitization elucidated by quantitative modeling of real time cAMP dynamics. J. Biol. Chem. 283, 2949–2961.
Kenakin T.P. 1997. Competitive antagonism. In: Pharmacologic Analysis of Drug-Receptor Interaction. Philadelphia: Lippincott-Raven, pp. 331–373.
Rajagopal S., Ahn S., Rominger D.H., Gowen-Mac-Donald W., Lam., DeWire S.M., Violin J.D., Lefkowitz R.J. 2011. Quantifying ligand bias at seven-transmembrane receptors. Mol. Pharmacol. 80, 367–377.
Rosenbaum D.M., Zhang C., Lyons J.A., Holl R., Aragao D., Arlow D.H., Rasmussen S.G.F., Choi H.J., DeVree B.T., Sunahara R.K. 2011. Structure and function of an irreversible agonist-[bgr] 2 adrenoceptor complex. Nature. 469, 236–240.
Scarselli M., Li B., Kim S.K., Wess J. 2007. Multiple residues in the second extracellular loop are critical for M3 muscarinic acetylcholine receptor activation. J. Biol. Chem. 282, 7385–7396.
Wheatley M., Simms J., Hawtin S., Wesley V., Wootten D., Conner M., Lawson Z., Conner A., Baker A., Cashmore Y. 2007. Extracellular loops and ligand binding to a subfamily of Family A G-protein-coupled receptors. Biochem. Soc. Trans. 35, 717.
Bokoch M.P., Zou Y., Rasmussen S.G., Liu C.W., Nygaard R., Rosenbaum D.M., Fung J J., Choi H.J., Thian F.S., Kobilka T.S., Puglisi J.D., Weis W.I., Pardo L., Prosser R.S., Mueller L., Kobilka B.K. 2010. Ligand-specific regulation of the extracellular surface of a G-protein-coupled receptor. Nature. 463, 108–112.
Peeters M., Van Westen G., Li Q., Ijzerman A. 2011. Importance of the extracellular loops in G protein-coupled receptors for ligand recognition and receptor activation. Trends Pharmacol. 32, 35–42.
Nygaard R., Frimurer T.M., Hoist B., Rosenkilde M.M., Schwartz T.W. 2009. Ligand binding and micro-switches in 7TM receptor structures. Trends Pharmacol. Sci. 30, 249–259.
Ballesteros J., Weinstein H. 1995. Integrated methods for modeling G-protetn coupled receptors. Methods Neurosci. 25, 366–428.
Ghanouni P., Schambye H., Seifert R., Lee T.W., Rasmussen S.G., Gether U., Kobilka B.K. 2000. The effect of pH on β2 adrenoceptor function: Evidence for protonation-dependent activation. J. Biol. Chem. 275, 3121–3127.
Dror R.O., Arlow D.H., Maragakis P., Mildorf T.J., Pan A.C., Xu H., Borhani D.W., Shaw D.E. 2011. Activation mechanism of the beta2-adrenergic receptor. Proc. Natl. Acad. Sci. U. S. A. 108, 18684–18689.
Bahar I. 2010. On the functional significance of soft modes predicted by coarse-grained models for membrane proteins. J.Gen. Phys. 135, 563–573.
Nygaard R., Zou Y., Dror R.O., Mildorf T.J., Arlow D.H., Manglik A., Pan A.C., Liu C.W., Fung J.J., Bokoch M.P. 2013. The dynamic process of β2-adrenergic receptor activation. Cell. 152, 532–542.
Author information
Authors and Affiliations
Corresponding author
Additional information
Original Russian Text © G.V. Novikov, V.S. Sivozhelezov, K.V. Shaitan, 2014, published in Molekulyarnaya Biologiya, 2014, Vol. 48, No. 3, pp. 463–479.
Rights and permissions
About this article
Cite this article
Novikov, G.V., Sivozhelezov, V.S. & Shaitan, K.V. Influence of orthosteric ligand binding on the conformational dynamics of the β-2-adrenergic receptor via essential dynamics sampling simulation. Mol Biol 48, 399–413 (2014). https://doi.org/10.1134/S0026893314030157
Received:
Accepted:
Published:
Issue Date:
DOI: https://doi.org/10.1134/S0026893314030157