Abstract
Isorhamnetin (ISO) is a methylated flavonol present in the leaves, flowers, and fruits of many plants with antitumour, anti-inflammatory, antioxidant, and anti-apoptotic properties. ISO has been suggested as the active substance in Vernonia anthelmintica (L.) to treat vitiligo. However, the mechanisms underlying its effects remain unclear. In this study, human keratinocytes (HaCaT cells) were pre-treated with or without ISO and then stimulated with hydrogen peroxide (H2O2) to generate oxidative damage. Pre-treatment with ISO increased HaCaT cell viability, reduced malondialdehyde content, and enhanced superoxide dismutase activity, resulting in a reduction in the loss of mitochondrial membrane potential, improved cell morphological damage, and apoptosis inhibition. Furthermore, we identified 51 significantly dysregulated differentially expressed genes (DEGs) of HaCaT cells treated with ISO using RNA-sequencing. Enrichment analysis using Gene Ontology and Kyoto Encyclopedia of Genes and Genomes databases indicated that the protective effect of ISO could be related to its effects on the Wnt signalling pathway. Our study provides novel insights into key gene regulation in the progression of oxidative damage and the mechanisms of action of ISO.
Similar content being viewed by others
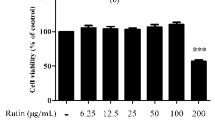
Introduction
Vitiligo is a common depigmentation disorder, clinically characterised by melanocyte destruction and melanosome loss, with a prevalence of 0.5–2% worldwide1. Vitiligo is often accompanied by various autoimmune diseases such as hyperthyroidism, diabetes, alopecia areata, psoriasis, and asthma2,3. It can occur in any part of the body, especially the exposed and wrinkled parts that are prone to friction and exposed to sunlight, and the palm plantar, mucosa, and retina can also be involved. Vitiligo affects a person’s appearance and can seriously impact their work and personal relationships; patients with vitiligo often suffer from social discrimination, causing anxiety or depression and other mental disorders, as the disfiguring spots hamper their social interactions2. Moreover, the disease is persistent and readily relapses; further, the treatment of this condition is difficult4. Although the exact cause of, or mechanism underlying, vitiligo remains mostly unknown, many theories have been proposed to explain the mechanism of pigmentation loss, such as autoimmunity, neural and genetic disease, impaired melanocyte migration and/or proliferation, and oxidative stress5. Oxidative stress is considered one of the most crucial initiators of vitiligo; it is involved in the development of vitiligo and is one of the factors responsible for the induction of the autoimmune response associated with the condition6,7.
Oxidative stress disturbs redox homeostasis and causes an imbalance between pro-oxidants and antioxidants8. Oxidative stress in tissues and cells results from excessive levels of reactive oxygen species (ROS). Oxidative stress-induced damage causes excessive aggregation of hydrogen peroxide (H2O2) in the epidermis of vitiligo patients, and a large increase in intracellular ROS levels. Oxidative stress can further cause oxidative damage to nucleic acids, proteins, and lipids, subsequently resulting in local melanocyte damage, apoptosis, and induction of an autoimmune response to attack more melanocytes, which causes progressive skin pigment loss9. Accumulating evidence indicates that keratinocytes are vital for the progression of vitiligo10. Although melanocytes are the main target cells of vitiligo, keratinocytes are also affected by the melanocyte destruction, which interrupts a vital nexus in the mechanisms underlying vitiligo development and progression11. Keratinocytes are mediators of detrimental oxidative stress, numerically dominate the cell composition of the epidermis, and directly interact with melanocytes. They can also transfer H2O2 to neighbouring melanocytes, which aggravates ROS accumulation and induces melanocyte destruction, thereby promoting vitiligo12. Oxidative stress has also been shown to induce damage to keratinocytes. Melanocytes and keratinocytes constitute the epidermal melanin units, which are closely related in structure and function, and play an important role in the transmission of melanosomes13. Keratinocytes, as mediators of oxidative stress, secrete cytokines to recruit autoreactive T cells that interfere with signal transduction in melanocytes, subsequently inducing melanocyte destruction10,11,12.
Isorhamnetin (ISO, 3-methyl quercetin; molecular formula: C16H12O7) is a methylated flavonol present in the leaves, flowers, and fruits of many plants14,15. ISO has numerous biological activities, including antitumour, anti-inflammatory, antioxidant, and anti-apoptotic properties16,17,18. Increasing evidence indicates that ISO from the plant material of Schisandra rubriflora, and its phenolic and anthocyanin compounds, has high antioxidant potential19,20. Moreover, the presence of phenolic compounds in bee bread significantly reduced the levels of oxidised-low-density lipoprotein (oxLDL) and malondialdehyde (MDA). It also significantly increased aortic antioxidant activities such as those of superoxide dismutase (SOD) and glutathione peroxidase (GPx)21. In addition, recent findings indicate that ISO is the main active substance in Vernonia anthelmintica (L.), and significantly increases the expression of melanin-biosynthetic genes (MC1R, MITF, TYR, TYRP1, and DCT) and tyrosinase activity in B16F10 cells22. Vernonia anthelmintica (L.), a traditional herbal medicine that is only grown in Kashgar, Aksu, and other areas in Xinjiang and India, is the most common herbal medicine for the treatment of vitiligo23. However, its mechanism of action remains unclear owing to the complexity of multiple ingredients and the lack of research on its chemical composition. In our previous study24, we identified 38 flavonoids from the seeds of V. anthelmintica (L.) using selective response/multiple response monitoring technology, and the content of total flavonoids was 0.97 g/100 g, among which ISO was present at a concentration of 93.23 ± 9.55 ng/g.
Currently, the pathogenesis of vitiligo is not well understood, treatment is difficult, and relapse is common25. Several treatment options aim to restore pigmentation, including excimer lasers, vitamin D analogues, and steroid therapy26. Unfortunately, these treatments are not widely employed because they induce long-term side effects27. Thus, several studies have focused on the identification of novel therapeutic medicines and on the assessment of multiple targets to explain the complex network of vitiligo-associated mechanisms. The aim of this study was to investigate the antioxidant activity and identify key genes to help reduce H2O2-induced oxidative damage in HaCaT cells (a human keratinocyte cell line) after ISO treatment. The results of the current study are expected to contribute to the development of ISO as a source of antioxidants and provide a theoretical basis for the targeted therapy of vitiligo.
Results
ISO protects HaCaT cells from H2O2-triggered injury
The effects of different concentrations of H2O2 on the viability, MDA content, and SOD enzyme activity in HaCaT cells are shown in Fig. 1. The MDA content (Fig. 1b) was increased in a dose-dependent manner and the SOD level (Fig. 1c) was reduced significantly as the concentration of H2O2 increased. Cell viability (Fig. 1a) in the H2O2-alone group (300, 600, 900, or 1200 μM H2O2) was significantly reduced compared with that of the control group (no H2O2), indicating that H2O2 induced cytotoxic damage to HaCaT cells (p < 0.05). The half-maximal inhibitory concentration (IC50) value of H2O2 was approximately 600 μM. Based on the above results, 600 μM H2O2 treatment was selected to establish the oxidative-stress model of HaCaT cells.
Effect of ISO on the cell viability, MDA content, and SOD enzyme activity after H2O2-treatment. The effect of H2O2 on (a) the cell viability and (b) MDA content and (c) SOD enzyme activity in HaCaT cells. Control, untreated HaCaT cells; The experimental groups, cells were treated with a concentration 300, 600, 900, 1200 μmol/L of the experimental sample for 24 h. The effect of ISO on (d) the cell viability, (e) MDA content, and (f) SOD enzyme activity in HaCaT cells. Control, untreated HaCaT cells; H2O2-alone group, HaCaT cells treated with 600 μM H2O2; all H2O2 + cells were treated with ISO 600 μM H2O2. Graphs show the mean ± SD values of three replication. One-way analysis of variance (ANOVA) followed by Tukey’s post-hoc test. # indicates significant differences (p < 0.05) from the control group. * indicates significant differences (p < 0.05) from the treatment of HaCaT cells with 600 μM H2O2 group.
The protective effect of ISO on the viability of HaCaT cells treated with H2O2 was detected using the CCK-8 assay. As shown in Fig. 1, as the concentration of ISO increased, the cell viability (Fig. 1d) in the experimental group (20, 40, or 60 μM ISO) became significantly higher than that in the H2O2-alone group. The expression levels of MDA (Fig. 1e) were higher in the H2O2-alone group than in the control group. Compared with the H2O2-alone group, the level of MDA in the samples pre-treated with ISO was significantly lower. The expression level of SOD (Fig. 1f) in the H2O2-alone group was significantly reduced compared with that in the control group, whereas the SOD level in cells pre-treated with ISO was significantly increased compared with that of the H2O2-alone group. These results indicate that ISO pre-treatment can significantly reduce the inhibitory effect of H2O2 on antioxidant enzymes.
ISO protects HaCaT cells from H2O2-induced apoptosis
The effect of ISO on the morphology of HaCaT cells injured by H2O2 is shown in Fig. 2. Compared with the control group (Fig. 2a), treatment of HaCaT cells with 600 μM H2O2 (Fig. 2b) induced apoptosis; compared with the H2O2-alone group, cells pre-treated with 40 μM ISO (Fig. 2c) revealed improved cell morphology and a reduction in the number of apoptotic cells. Apoptosis is mediated via mitochondrial membrane permeabilisation events18. To investigate whether ISO can inhibit H2O2-induced apoptosis stemming from mitochondrial dysfunction, the effect of ISO on the mitochondrial membrane potential of HaCaT cells after H2O2-triggered injury was assessed. Confocal microscopy showed that the mitochondria of H2O2-induced cells emitted weak red fluorescence and strong green fluorescence (Fig. 2d), indicating that the mitochondrial membrane potential of cells was disrupted. However, after ISO pre-treatment, the red fluorescence was significantly higher than that in the H2O2-alone group (Fig. 2e), indicating that the increase in green fluorescence intensity was almost completely prevented by ISO. These results showed that ISO could reduce the loss of mitochondrial membrane potential in HaCaT cells with H2O2-triggered injury.
The effect of isorhamnetin (ISO) on the morphology of HaCaT cells injured by H2O2 (40 × magnification). (a) Untreated HaCaT cells showing a normal cell morphology. (b) HaCaT cells stimulated with 600 μM H2O2 showed increased apoptosis. (c) HaCaT cells treated with 600 μM H2O2 + 40 μM ISO group, compared with H2O2-only treatment, showing improvement in cell morphology and a reduction in the number of apoptotic cells. (d) Confocal microscopy images of HaCaT cells were treated with 600 μM H2O2 to detect mitochondrial permeability; weak red fluorescence and strong green fluorescence indicates disruption of mitochondrial membrane potential. (e) Confocal microscopy of cells treated with 600 μM H2O2 + 40 μM ISO treatment, showing stronger red fluorescence. (f) Apoptosis rate of cells treated with hydrogen peroxide as assessed by PI staining and flow cytometry. Control, untreated HaCaT cells; H2O2-alone group, HaCaT cells treated with 600 μM H2O2; all H2O2 + ISO were 600 μM H2O2. Graphs show the mean ± SD values of three replication. One-way ANOVA followed by Tukey’s post-hoc test. # indicates significant differences (p < 0.05) from the control group. * indicates significant differences (p < 0.05) from the treatment of HaCaT cells with 600 μM H2O2 group.
To investigate the effects of ISO on the H2O2-induced apoptosis of HaCaT cells, propidium iodide (PI) staining was performed, as shown in Fig. 2f. The apoptosis rates of the control group, H2O2-alone group, H2O2 + 20 μM ISO, H2O2 + 40 μM ISO and H2O2 + 60 μM ISO were 4.13 ± 0.21%, 20.07 ± 0.85%, 15.17 ± 0.55%, 8.07 ± 0.15% and 7.13 ± 0.21%, respectively. Thus, compared with the H2O2-alone group, cell apoptosis was alleviated in the groups pre-treated with ISO, in a dose-dependent manner.
Differentially expressed genes (DEGs) and functional enrichment analysis
We selected three groups of HaCaT cells (control, H2O2-alone, and H2O2 + 40 μM ISO), and used three biological replicates for RNA sequencing (RNA-seq). DEGs were identified according to the criteria of p < 0.05 and |log2 FC| > 1 as a measure of significance for DEGs. Volcano plots (Fig. 3a,b) show the DEG expression profiles of the H2O2-alone vs. control groups and, the ISO group vs. H2O2-alone group. A total of 2316 DEGs (906 upregulated and 1410 downregulated) in the H2O2-alone vs. control groups, and 262 DEGs (147 upregulated and 115 downregulated) in the ISO vs. H2O2 groups were identified.
Differentially expressed genes (DEGs). (a,b) Volcano plot of gene expression profiles in the (a) control vs. H2O2 and (b) ISO vs. H2O2 comparisons. (c–e) Venn diagrams showing (c) unique or shared DEGs for those (d) upregulated in H2O2 vs. control and downregulated in H2O2 + ISO and genes that are (e) downregulated in H2O2 vs. control and upregulated in H2O2 + ISO. H2O2 hydrogen peroxide, ISO isorhamnetin, control group untreated HaCaT cells, H2O2 group HaCaT cells treated with 600 μM H2O2 for 12 h, ISO group 600 μM H2O2 + 40 μM ISO.
A Venn diagram of the identified DEGs is shown in Fig. 3c–e. There were 51 common DEGs between the H2O2 vs. control and H2O2 vs. ISO comparison groups (Fig. 3c). Twenty-three genes were upregulated by H2O2 and downregulated by H2O2 + ISO (Fig. 3d), including follistatin and glycerol-3-phosphate acyltransferase 3 (Fig. 4a). In addition, 28 genes were downregulated by H2O2 and upregulated by H2O2 + ISO (Fig. 3e), including keratin 3 (KRT3) and Wnt family member 4 (WNT4) (Fig. 4b). Treatment with ISO reversed the effect of H2O2, producing gene expression profiles similar to those observed in the control group. We subsequently performed a functional enrichment analysis using these 51 DEGs. A heat map (Fig. 4c,d) depicts selected DEGs.
Functional analysis. (a,b) Volcano plot and (c,d) Heatmaps of the (a,c) 23 DEGs that were upregulated by H2O2 and downregulated by ISO and the (b,d) 28 DEGs that were downregulated by H2O2 and upregulated by ISO. The heatmap was generated by TB tools software. H2O2 hydrogen peroxide, ISO isorhamnetin, control group untreated HaCaT cells, H2O2 group HaCaT cells treated with 600 μM H2O2 for 12 h, ISO group 600 μM H2O2 + 40 μM ISO.
To gain a better understanding of the potential relationships among these 51 DEGs, we conducted a functional enrichment analysis. Gene Ontology (GO) analysis (Fig. 4a) revealed that the biological processes of the top 30 DEGs, as shown in Fig. 5a, and the most enriched biological processes (BP) were blood vessel development, vasculature development, and tertiary alcohol metabolic process. The most enriched cellular component (CC) terms were receptor regulator activity, receptor ligand activity, and phenanthrene 9,10-monooxygenase activity, and the most enriched molecular function (MF) terms were extracellular region, Golgi lumen, and extracellular region. The top enriched KEGG pathways are shown in Fig. 5b, with the bubble diagram illustrating the top 20 enriched pathways, suggesting that the Wnt signalling pathway; steroid hormone biosynthesis; and neomycin, kanamycin, and gentamicin biosynthesis may be involved in the protective effect of ISO against oxidative stress in HaCaT cells.
(a) Gene Ontology analysis of differentially expressed genes (DEGs) altered by isorhamnetin (ISO). The y-axis shows the GO term, and the x-axis shows the − log10 (p value). (b) Kyoto Encyclopedia of Genes and Genomes (KEGG) enrichment bubble map of DEGs altered by ISO. The y-axis shows the pathway name, and the x-axis shows the corresponding p value of the pathway. The rich factor is the number of DEGs/the total number of genes detected in the pathway.
DEG interaction network analysis
We applied the interaction relations in the STRING protein interaction database (http://string-db.org/), and directly extracted the interaction relations of target DEG sets from the database to construct the network in the database. The interaction network diagram of DEGs shows that for the control vs. H2O2-alone group comparison, the upregulated DEGs protein phosphatase 2 scaffold subunit A beta, signal transducer and activator of transcription 1, and cytoplasmic linker associated protein 2, and the downregulated DEGs mitogen-activated protein kinase 3, adenylate cyclase 6, and histone deacetylase 5 were located in the centre of the network, suggesting that these may be the key genes involved in the oxidative stress-induced damage of HaCaT cells.
Discussion
Vernonia anthelmintica (L.) Willd has been demonstrated to possess several pharmacological properties, including anti-inflammatory, antibacterial, antioxidant, hypoglycaemic, and antithrombotic activities28. Its seeds are used in traditional therapy for the treatment of skin diseases, including vitiligo29, and its chemical composition is complex; the effective components for vitiligo treatment are mainly flavonoids and caffeic acids30. However, current research on vitiligo treatment with Vernonia anthelmintica (L.) Willd is mostly limited to the total flavonoid extraction level, and there are few studies on flavonoid monomers. In our previous study, we identified 38 flavonoids from the seeds of V. anthelmintica (L.) that contain ISO, a bioflavonoid with potential antioxidant properties15. To the best of our knowledge, this is the first comprehensive study to reveal the key genes involved in the protective effect of ISO against H2O2-induced oxidative damage in vitro. Our findings indicated that pre-treatment with ISO markedly increased cell viability in a dose-dependent manner, more than was observed for cells treated with H2O2 alone. We also found that pre-treatment with ISO reduced the MDA content, enhanced SOD activity, and inhibited apoptosis. Mitochondrial dysfunction is considered an important contributing factor to a variety of pathophysiological situations including aging, heart ischemia/reperfusion injury, diabetes, and cardiovascular diseases, all of which are associated with cell death31,32. Mitochondrial dysfunction results in the collapse of the mitochondrial membrane potential, and it has also been shown that the mitochondrial membrane potential decreases in the early stage when stimulated by oxidative stress, and the damage can be reversed by antioxidant treatment33. In our study, we found that pre-treatment with ISO reduced the loss of mitochondrial membrane potential, improved cell morphological damage, and inhibited apoptosis in H2O2-treated HaCaT cells. This indicates that ISO reverses the mitochondrial damage to some extent and sheds new light on the potential of ISO to serve as an antioxidant therapy for vitiligo.
We focused on the DEGs affected in both H2O2-induced HaCaT cells and those pre-treated with ISO. The DEGs observed in our model system of oxidative stress could be helpful for identifying specific biomarkers of oxidative stress-related diseases. In our study, a total of 2316 H2O2-responsive genes (906 upregulated and 1410 downregulated), and 262 ISO-responsive genes (147 upregulated and 115 downregulated) were detected, and a large number of DEGs were functionally characterised. By comparing the gene expression of the three groups, we determined that 51 DEGs were potentially related to the protective effect of ISO against oxidative stress in HaCaT cells. Of these, three genes were newly discovered, thus, further follow-up studies are warranted for their functional annotation. Regarding the other genes that have been previously annotated, some are involved in oxidative stress: follistatin protects against oxidative stress and apoptosis both in vitro and in vivo in chronic kidney disease34. Moreover, interleukin-1 receptor antagonist (IL-1RA) was shown to ameliorate pain hypersensitivity, spinal inflammation, and oxidative stress induced by systemic lipopolysaccharides in neonatal rats35. CTRP3 ameliorates fructose-induced metabolic-associated fatty liver disease by inhibiting xanthine oxidase-associated oxidative stress36. Some of the identified genes are also involved in apoptosis. Radiation-induced apoptosis enhancing nuclease (AEN) cleaves DNA in concert with other apoptotic nucleases, thereby enhancing apoptosis following ionising irradiation37, and knockdown of the MET transcriptional regulator MACC1 sensitises cancer cells to death receptor-mediated apoptosis in solid cancers38. Similar results were observed in the present study. Elevated levels of FST, IL1RL1, XDH, AEN, and MACC1 were identified as DEGs in the H2O2-treated group compared to the control group. Notably, the expression of these genes decreased after pre-treatment with ISO. However, there is limited knowledge regarding the potential functions of these dysregulated DEGs. Future studies will contribute to identifying the precise mechanism of these DEGs in the protective effects of ISO against oxidative injury.
Moreover, 51 DEGs were identified as being possibly related to the antioxidant activities of ISO, and the KEGG enrichment function analysis suggested that the protective effect of ISO may be related to the Wnt signalling pathway and steroid hormone biosynthesis. Wnt signalling plays a crucial role in melanocyte stem cell differentiation39. Moreover, it has been shown that Wnt/β-catenin signalling plays a pivotal role in cell proliferation, migration, and differentiation in vitiligo pigmentation systems40. Regazzetti et al.41 demonstrated that in lesional vitiligo, oxidative stress decreases Wnt expression in melanocytes and keratinocytes. They further demonstrated that pharmacological agents that activate the Wnt pathway successfully induced the differentiation of resident stem cells into pre-melanocytes, suggesting that stimulation of Wnt signalling may serve as an adjunct to current therapies for vitiligo42. Mei et al. found that the Wnt5a gene in the canonical Wnt/β-catenin pathway can promote melanocyte differentiation and proliferation43. The present study showed that ISO might exert an antioxidant effect by influencing the Wnt pathway, with involvement of Wnt family DEGs (WNT4 and WNT5B). These observations may support further clinical exploration of Wnt agonists to repigment vitiligo lesions. Based on data from public databases, the DEG network was visualised for a comprehensive analysis of key genes and gene pathways involved in the counteraction of H2O2-induced oxidative damage in HaCaT cells by ISO. This approach could suggest new targeted therapies for vitiligo. Furthermore, GO analysis revealed that the most enriched biological process, molecular function, and cellular component terms of these DEGs were the tertiary alcohol metabolic process, receptor regulator activity, and extracellular region, respectively. DEG interaction network analysis suggested that the key genes were closely involved in the oxidative stress damage in HaCaT cells. Future studies are likely to characterise the mechanisms of action of new transcripts and genes in the protective effects of ISO against oxidative injury.
The present investigation had some limitations. First, validation experiments should be conducted to determine whether manipulating the expression of the top candidate DEGs can affect the various functional readouts of ISO-treated and H2O2-induced HaCaT cells., Further research is necessary to observe potential changes in the apoptotic rate of H2O2-induced HaCaT cells by manipulating the expression of top DEG candidates. Additionally, DEG changes in animal models of oxidative damage should also be examined. Second, to address the lack of research on the effect of ISO-involved antioxidant pathways, future studies should aim to more accurately demonstrate the antioxidant stress mechanism of ISO. Third, although the protective effect of ISO against H2O2-induced oxidative damage in HaCaT cells was investigated in the present study, further experiments and collaborative discussions should be held to identify the effect of ISO on H2O2-induced melanocytes, which would improve the development of ISO as a source of antioxidants and provide a theoretical basis for the targeted therapy of vitiligo.
Conclusions
We demonstrated the protective effect of ISO against H2O2-induced oxidative damage in HaCaT cells, including increased cell viability, reduced MDA content, and enhanced SOD activity compared with cells treated with H2O2 alone. In addition, we found that pre-treatment with ISO reduced the loss of mitochondrial membrane potential, improved cell morphological damage, and inhibited apoptosis in H2O2-induced HaCaT cells. In addition, changes in the key genes of untreated HaCaT cells vs. H2O2-induced HaCaT cells with and without ISO intervention were identified. By combining the functional results with those obtained from the bioinformatics analysis, this study highlighted some aberrantly expressed DEGs and gene pathways that might be related to oxidative stress and the antioxidant effect of ISO. One pathway of note was the Wnt signalling pathway.
Methods
Chemicals and reagents
H2O2 solution, RIPA lysis buffer, 3,5-dimethylphenol (DMSO), and phosphate-buffered saline (PBS) were obtained from Sigma (Shanghai, China). ISO (C16H12O7), SOD, MDA, and proliferation and cytotoxicity assay (CCK-8) kits were obtained from Solarbio (Beijing, China). Dulbecco’s modified Eagle medium (DMEM) and foetal bovine serum (FBS) were purchased from BI Company (Ridgefield CT, USA). Penicillin–streptomycin solution was obtained from Hyclone (Logan, UT, USA). Trypticase was purchased from Gibco (Waltham, MA, USA). Trizol was purchased from Magen; trichloromethane and isopropanol were purchased from Sinopharm; and the RNA Nano 6000 Assay Kit, NEBNext Ultra™ RNA Library Prep Kit for Illumina, and AMPure XP system were from Agilent Technologies (Santa Clara, CA, USA), ABclonal (Woburn, MA, USA), and Beckman Coulter (Brea, CA, USA), respectively. The BCA Protein Quantitation Kit was obtained from Thermo Fisher Scientific (Waltham, MA, USA).
Cell culture and treatment
Human keratinocytes (HaCaT; Kunming Cell Bank, Chinese Academy of Sciences) were cultured in DMEM supplemented with 10% FBS and a 1% antibiotic mixture containing penicillin and streptomycin. The cells were grown at 37 °C in a humidified atmosphere containing 5% CO2. The experiments were performed 24 h after the cells were seeded. HaCaT cells (8 × 104 cells/well) were seeded in 96-well plates for 12 h and then treated with various concentrations (300, 600, 900, or 1200 μM) of H2O2. Similarly, HaCaT cells were pre-treated with various concentrations (20, 40, 60, or 80 μM) of ISO in the medium prepared above for 12 h to determine the optimal treatment concentration. Subsequently, the pre-treated cells were cultured for an additional 12 h with 600 μM H2O2 to stimulate oxidative damage. Three replicates per group were used for all experiments. Untreated cells and cells treated with H2O2 alone were used as controls.
Cell viability assay
The cellular toxicity of H2O2 and the protective effect of ISO were measured in HaCaT cells using the CCK-8 assay. After treatment with the indicated concentrations of H2O2 or ISO, 10 μL of the CCK-8 kit solution was added, and the cells were incubated for 2 h at 37 °C. Subsequently, the absorbance was measured using a microplate reader (Thermo Fisher Scientific).
Biochemical analysis
Cold PBS was used to collect treated and untreated HaCaT cell samples after centrifugation at 157×g for 3 min according to the instructions provided in each enzyme kit. The cells were homogenised with cold PBS, and commercial kits were used to obtain the supernatant for the measurement of MDA and SOD activities.
Cell apoptosis assay
The protective effect of ISO on the oxidative stress-induced apoptosis of HaCaT cells was determined using the Hoechst assay. Cells pre-treated with 20, 40, and 60 μM ISO were used as the experimental group. After treatment of the cells, 5 μg/mL Hoechst staining solution was added, and the cells were incubated for 10–15 min at 25 °C in the dark. Subsequently, 400 μL of 1 × binding buffer was added, mixed, and placed on ice, and the images of the cells were captured within 1 h under an Olympus fluorescence microscope. This experiment was repeated three times.
SOD activity
SOD activity in HaCaT cells was measured using the nitroblue tetrazolium method. HaCaT cells at the logarithmic growth stage were digested and washed. The cell suspension was centrifuged at 157×g for 5 min. HaCaT cells (1 × 106 cells/well) were seeded in 6-well plates for 12 h and grown at 37 °C in a humidified atmosphere containing 5% CO2. When the cells adhered to the wells and the density was approximately 80%, the cells were treated with various concentrations (20, 40, or 60 μM) of ISO for 12 h. Subsequently, the pre-treated cells were cultured for an additional 12 h with 600 μM H2O2 for 12 h. The cell suspension was then mixed thoroughly with a vortex mixer (ice bath; power 20% or 200 W; ultrasound 3-s, 10-s intervals, 30 repeats) and centrifuged at 8000×g at 4 °C for 10 min. The reagents were added to the cell suspension, according to the manufacturer’s instructions. Finally, the mixture was thoroughly mixed and cultured at 37 °C for 30 min, placed in a 1-mL glass cuvette, and the maximal absorbance of the mixture was measured at 560 nm using a spectrophotometer. One unit of SOD was defined as the amount of enzyme that caused 50% inhibition.
MDA assay
The MDA concentration was used to determine lipid peroxidation. The cell suspension was mixed thoroughly with a vortex mixer (ice bath, power 20% or 200 W; ultrasound 3-s, 10-s intervals, 30 repeats) and centrifuged at 8000×g at 4 °C for 10 min. The reagents were added to the cell suspension, according to the manufacturer’s instructions. Finally, the mixture was thoroughly mixed and incubated at 100 °C for 60 min, cooled in an ice bath, and centrifuged at 10,000×g for 10 min. Two hundred microliters of supernatant were transferred to a microglass cuvette or 96-well plate, and the maximal absorbance was determined at 450 nm, 532 nm, and 600 nm using a spectrophotometer. The MDA content was calculated according to the protein concentration.
Mitochondrial ultrastructure observation
HaCaT cells were inoculated into 25-cm2 culture flasks and cultured to approximately 80% confluency for fusion. Experimental groups were divided into four groups: blank, control, H2O2-alone, and ISO + H2O2. The treatment methods for each group were the same as those described above. Cells were collected into 10-mL centrifuge tubes 24 h after routine digestion and centrifuged at 1000×g for 5 min. The supernatant was discarded and 300 µL of high-sugar complete medium was added to the cell precipitate for resuspension. The cell suspension was transferred into a 0.5-mL EP tube, centrifuged at 2000×g for 8 min, and the supernatant was carefully removed. The EP tube was tilted at 45°, and 2.5% glutaraldehyde solution was slowly added to the tube wall with a pipette tip until the entire EP tube was filled, followed by fixation at 4 °C for 24 h. The fixed specimens were sent to the Electron Microscopy Center of the Basic Laboratory of Xinjiang Medical University for transmission electron microscopy.
RNA extraction and monitoring of quality
We selected three groups of HaCaT cells for RNA sequencing: untreated cells, 600 μM H2O2-induced cells, and H2O2-induced cells pre-treated with 40 μM ISO (pre-treatment with ISO markedly increased cell viability in a dose-dependent manner versus that observed for cells treated with H2O2 alone. 40 μM ISO was significantly different to 20 μM but there was no difference with 60 μM. The cells pre-treated with 40 μM ISO were used as the protection group). Total RNA was extracted from the cells using TRIzol reagent according to the manufacturer’s instructions. The absorbance ratio at 260–280 nm of the RNA samples was measured with a Nanodrop ND-2000 system (Thermo Fisher Scientific) and the RNA integrity number was determined using an Agilent Bioanalyzer 4150 system (Agilent Technologies). Only high-quality samples according to assessments of RNA purity, concentration, and integrity were used for library construction.
Library preparation and sequencing
Paired-end libraries were prepared using ABclonal mRNA-seq Lib Prep Kit (ABclonal) following the manufacturer’s instructions. The mRNA was purified from 1 mg total RNA using oligo (dT) magnetic beads, followed by fragmentation using divalent cations at elevated temperatures in ABclonal First Strand Synthesis Reaction Buffer. Subsequently, first-strand cDNA was synthesised with random hexamer primers and reverse transcriptase using mRNA fragments as templates, followed by second-strand cDNA synthesis using DNA polymerase I, RNase H, and dNTPs. The synthesised double-stranded cDNA fragments were then adapter-ligated to prepare the paired-end library. Adaptor-ligated cDNA was used for polymerase chain reaction (PCR) amplification. PCR products were purified (AMPure XP system) and library quality was assessed using an Agilent Bioanalyzer 4150 system. Sequencing was performed using an Illumina Novaseq 6000/MGISEQ-T7 instrument.
Data analysis
The data generated from the Illumina/BGI platform were used for the bioinformatic analysis. All analyses were performed using an in-house pipeline from Shanghai Applied Protein Technology.
Quality control
Raw data (or raw reads) of FASTQ format were first processed using in-house Perl scripts. In this step, the adapter sequence was removed, and low-quality reads [the number of lines with a string quality value less than or equal to 25 accounting for more than 60% of the entire read, or greater than 5% Ns (unknown base)] were filtered out to obtain clean reads that could be used for subsequent analysis.
Quantification of gene expression level and differential expression analysis
Clean reads were separately aligned to the reference genome in orientation mode using HISAT2 software (http://daehwankimlab.github.io/hisat2/) to obtain mapped reads, which were then spliced with Stringtie software (http://ccb.jhu.edu/software/stringtie/). Gffcompare software (http://ccb.jhu.edu/software/stringtie/gffcompare.shtml) was used for comparing the obtained sequences with the reference genome GTF/GFF file to find the original unannotated transcription region, and to discover new transcripts and new genes. FeatureCounts (http://subread.sourceforge.net/) was used to count the read numbers mapped to each gene. The expression level in FPKM of each gene was calculated based on the length of the gene and read counts mapped to the gene. Differential expression analysis was performed using the DESeq2 package (http://bioconductor.org/packages/release/bioc/html/DESeq2.html); differentially expressed genes (DEGs) with |log2 fold change (FC)| > 1 and adjusted p value (Padj) < 0.05 were considered significant DEGs.
Enrichment analysis
The Gene Ontology (GO) and Kyoto Encyclopedia of Genes and Genomes (KEGG) pathway analyses (http://www.kegg.jpkegg/kegg1.html) can explain the functional enrichment of DEGs and clarify the differences between samples at the gene function level44. We used the clusterProfiler R software package for GO function enrichment and KEGG pathway enrichment analysis. When p < 0.05, it was considered that the GO or KEGG function was significantly enriched. Transcription factors of the DEGs were extracted directly from the AnimalTFDB (http://bioinfo.life.hust.edu.cn/AnimalTFDB/)/PlantTFDB/)/PlantTFDB (http://planttfdb.cbi.pku.edu.cn/) databases.
Protein–protein interaction (PPI) analysis
PPI analysis was used to evaluate whether there was an interaction between gene products and other proteins. The analysis was based on protein information corresponding to genes. The known and predicted PPIs were obtained from the STRING database (https://www.string-db.org/). For the gene sequences existing in the database, the networks were constructed by extracting the target gene list from the database. For gene sequences not included in the database, the target gene set sequence was first aligned with the reference sample protein sequence contained in the STRING protein interaction database with BLASTx, and then the protein interaction relationship of the reference gene sequences was used to establish an interaction network. rMATS (http://rnaseq-mats.sourceforge.net/index.html), a variable splicing analysis software suitable for RNA-sequencing data, was used for analysis.
Statistical analysis
SPSS (version 22.0; IBM Corp., Armonk, NY, USA) was used for statistical analysis. Statistical significance was set at p < 0.05. DEseq software was used to process the sequencing data. DEGs were identified according to the criteria of p < 0.01 and |log2FC)| > 2.
Data availability
The data used to support the findings of this study are available from the corresponding author upon request.
References
Singh, M., Vaishnav, J., Shah, A. & Begum, R. Expression analysis of candidate genes in vitiligo patients and effect of oxidative stress on melanocytes. Gene Rep. 25, 101389 (2021).
Dabas, G., Vinay, K., Parsad, D., Kumar, A. & Kumaran, M. S. Psychological disturbances in patients with pigmentary disorders: A cross-sectional study. J. Eur. Acad. Dermatol. Venereol. 34(2), 392–399 (2020).
Laberge, G. et al. Early disease onset and increased risk of other autoimmune diseases in familial generalized vitiligo. Pigment Cell Res. 18(4), 300–305 (2005).
Zhang, J. et al. Research progress on targeted antioxidant therapy and vitiligo. Oxid. Med. Cell. Longev. 2022, 1821780 (2022).
Laddha, N. et al. Vitiligo: Interplay between oxidative stress and immune system. Exp. Dermatol. 22(4), 245–250 (2013).
Li, S. et al. Oxidative stress drives CD8(+) T-cell skin trafficking in patients with vitiligo through CXCL16 upregulation by activating the unfolded protein response in keratinocytes. J. Allergy Clin. Immunol. 140(1), 177-189.e179 (2017).
Zhang, Y. et al. Oxidative stress-induced calreticulin expression and translocation: New insights into the destruction of melanocytes. J. Investig. Dermatol. 134(1), 183–191 (2014).
Janjetovic, Z. et al. Melatonin and its metabolites protect human melanocytes against UVB-induced damage: Involvement of NRF2-mediated pathways. Sci. Rep. 7(1), 1274 (2017).
Xie, H. et al. Vitiligo: How do oxidative stress-induced autoantigens trigger autoimmunity?. J. Dermatol. Sci. 81(1), 3–9 (2016).
Chen, X. et al. Oxidative stress-induced IL-15 trans-presentation in keratinocytes contributes to CD8+ T cells activation via JAK-STAT pathway in vitiligo. Free Radic. Biol. Med. 139, 80–91 (2019).
Chen, J., Li, S. & Li, C. Mechanisms of melanocyte death in vitiligo. Med. Res. Rev. 41(2), 1138–1166 (2021).
Qiao, Z., Wang, X., Xiang, L. & Zhang, C. Dysfunction of autophagy: A possible mechanism involved in the pathogenesis of vitiligo by breaking the redox balance of melanocytes. Oxid. Med. Cell. Longev. 2016, 3401570–3401577 (2016).
Delmas, V. & Larue, L. Molecular and cellular basis of depigmentation in vitiligo patients. Exp. Dermatol. 28(6), 662–666 (2019).
Skalski, B. et al. Isorhamnetin and its new derivatives isolated from sea buckthorn berries prevent H2O2/Fe-Induced oxidative stress and changes in hemostasis. Food Chem. Toxicol. 125, 614–620 (2019).
Gong, G. et al. Isorhamnetin: A review of pharmacological effects. Biomed. Pharmacother. 128, 110301 (2020).
Yang, J. H. et al. O-Methylated flavonol isorhamnetin prevents acute inflammation through blocking of NF-kappaB activation. Food Chem. Toxicol. 59, 362–372 (2013).
Yang, J. H. et al. Isorhamnetin protects against oxidative stress by activating Nrf2 and inducing the expression of its target genes. Toxicol. Appl. Pharmacol. 274(2), 293–301 (2014).
Han, X. et al. Isorhamnetin protects human keratinocytes against ultraviolet B-induced cell damage. Biomol. Ther. 23(4), 357–366 (2015).
Szopa, A. et al. Schisandra rubriflora Plant material and in vitro microshoot cultures as rich sources of natural phenolic antioxidants. Antioxidants 9(6), 488 (2020).
Diep, T., Pook, C. & Yoo, M. Phenolic and anthocyanin compounds and antioxidant activity of tamarillo (Solanum betaceum Cav.). Antioxidants 9(2), 169 (2020).
Othman, Z. A., Ghazali, W. S. W., Noordin, L., Yusof, N. A. M. & Mohamed, M. Phenolic compounds and the anti-atherogenic effect of bee bread in high-fat diet-induced obese rats. Antioxidants 9(1), 33 (2020).
Wang, J. Y. et al. Network pharmacological mechanisms of Vernonia anthelmintica (L.) in the treatment of vitiligo: Isorhamnetin induction of melanogenesis via up-regulation of melanin-biosynthetic genes. BMC Syst. Biol. 11(1), 103 (2017).
Zhou, J., Shang, J., Ping, F. & Zhao, G. Alcohol extract from Vernonia anthelmintica (L.) willd seed enhances melanin synthesis through activation of the p38 MAPK signaling pathway in B16F10 cells and primary melanocytes. J. Ethnopharmacol. 143(2), 639–647 (2012).
Hu, W., Wang, H. & Kang, X. Analysis of the flavonoid constituents of Vernonia anthelmintica (L.) Willd. by SRM/MRM. J. Xinjiang Med. Univ. 44, 731–736 (2021).
Birlea, S. A., Goldstein, N. B. & Norris, D. A. Repigmentation through melanocyte regeneration in vitiligo. Dermatol. Clin. 35(2), 205–218 (2017).
Li, K. et al. The association of vitamin D receptor gene polymorphisms and serum 25-hydroxyvitamin D levels with generalized vitiligo. Br. J. Dermatol. 167(4), 815–821 (2012).
Picardo, M. et al. Vitiligo. Nat. Rev. Dis. Primers 1, 15011 (2015).
Jamil, S., Khan, R. A., Ahmed, S. & Fatima, S. Evaluation of anti-inflammatory and anti-oxidant potential of seed extracts of Vernonia anthelmintica. Pak. J. Pharm. Sci. 30, 755–760 (2017).
Tuerxuntayi, A. et al. Kaliziri extract upregulates tyrosinase, TRP-1, TRP-2 and MITF expression in murine B16 melanoma cells. BMC Complement. Altern. Med. 14, 166 (2014).
Orhan, I. E. & Khan, M. T. H. Flavonoid derivatives as potent tyrosinase inhibitors—A survey of recent findings between 2008–2013. Curr. Top. Med. Chem. 14(12), 1486–1493 (2014).
Blanch, M., Mosquera, J. L., Ansoleaga, B., Ferrer, I. & Barrachina, M. Altered mitochondrial DNA methylation pattern in Alzheimer disease related pathology and in Parkinson disease. Am. J. Pathol. 186, 385–397 (2016).
Kleszczyński, K., Zillikens, D. & Fischer, T. W. Melatonin enhances mitochondrial ATP synthesis, reduces reactive oxygen species formation, and mediates translocation of the nuclear erythroid 2-related factor 2 resulting in activation of phase-2 antioxidant enzymes (γ-GCS, HO-1, NQO1) in ultraviolet rad. J. Pineal Res. 61(2), 187–197 (2016).
Umbaugh, D., Nguyen, N., Jaeschke, H. & Ramachandran, A. Mitochondrial membrane potential drives early change in mitochondrial morphology after acetaminophen exposure. Toxicol. Sci. 180(1), 186–195 (2021).
Mehta, N., Gava, A., Zhang, D., Gao, B. & Krpeinsky, K. Sun-189 Follistatin protects against ER stress-induced oxidative stress and apoptosis both in vitro and in vivo in CKD. Kidney Int. Rep. 4, S237 (2019).
Hsieh, C. T. et al. Interleukin-1 receptor antagonist ameliorates the pain hypersensitivity, spinal inflammation and oxidative stress induced by systemic lipopolysaccharide in neonatal rats. Neurochem. Int. 135, 104686 (2020).
Zhang, J., Xu, J., Lin, X., Tang, F. & Tan, L. CTRP3 ameliorates fructose-induced metabolic associated fatty liver disease via inhibition of xanthine oxidase-associated oxidative stress. Tissue Cell 72, 101595 (2021).
Lee, J. H. et al. Identification of a novel ionizing radiation-induced nuclease, AEN, and its functional characterization in apoptosis. Biochem. Biophys. Res. Commun. 337, 39–47 (2005).
Radhakrishnan, H. et al. MACC1 regulates Fas mediated apoptosis through STAT1/3-Mcl-1 signaling in solid cancers. Cancer Lett. 403, 231–245 (2017).
Yamada, T. et al. Wnt/beta-catenin and kit signaling sequentially regulate melanocyte stem cell differentiation in UVB-induced epidermal pigmentation. J. Investig. 133, 2753–2762 (2013).
Birlea, S. A., Costin, G. E., Roop, D. R. & Norris, D. A. Trends in regenerative medicine: Repigmentation in vitiligo through melanocyte stem cell mobilization. Med. Res. Rev. 37(4), 907–935 (2017).
Regazzetti, C. et al. Transcriptional analysis of vitiligo skin reveals the alteration of Wnt pathway: A promising target for repigmenting vitiligo patients. J. Investig. Dermatol. 135(12), 3105–3114 (2015).
Zou, D. P. et al. SFRP5 inhibits melanin synthesis of melanocytes in vitiligo by suppressing the Wnt/β-catenin signaling. Genes Dis. 8, 677–688 (2021).
Mei, X., Wu, Z., Huang, J., Sun, Y. & Shi, W. Screening and analysis of differentially expressed genes of human melanocytes in skin cells mixed culture. Am. J. Transl. Res. 11(5), 2657–2667 (2019).
Kanehisa, M., Furumichi, M., Sato, Y., Ishiguro-Watanabe, M. & Tanabe, M. KEGG: Integrating viruses and cellular organisms. Nucleic Acids Res. 49(D1), D545–D551 (2021).
Acknowledgements
This study was funded by the National Natural Science Foundation of China (82173406), the authors gratefully acknowledge the support of all partners involved in the study, and the support from the Dermatology Institute of the People’s Hospital of Xinjiang Uygur Autonomous Region. We would like to thank Shanghai Applied Protein Technology for the measurements and basic analysis of RNA-seq, and Editage (http://www.editage.cn) for their assistance with English language editing.
Author information
Authors and Affiliations
Contributions
K.X.J. conceived, designed, and supervised the research project. H.W. and Z.J.Z. performed the experiments and prepared the manuscript. W.H.J., D.L.H. and L.J. performed the cell experiments. H.W. and G.M.M. provided comments and suggestions regarding the preparation of the manuscript. K.X.J. supervised the research project and edited the manuscript. All authors have read and agreed to the published version of the manuscript.
Corresponding author
Ethics declarations
Competing interests
The authors declare no competing interests.
Additional information
Publisher's note
Springer Nature remains neutral with regard to jurisdictional claims in published maps and institutional affiliations.
Rights and permissions
Open Access This article is licensed under a Creative Commons Attribution 4.0 International License, which permits use, sharing, adaptation, distribution and reproduction in any medium or format, as long as you give appropriate credit to the original author(s) and the source, provide a link to the Creative Commons licence, and indicate if changes were made. The images or other third party material in this article are included in the article's Creative Commons licence, unless indicated otherwise in a credit line to the material. If material is not included in the article's Creative Commons licence and your intended use is not permitted by statutory regulation or exceeds the permitted use, you will need to obtain permission directly from the copyright holder. To view a copy of this licence, visit http://creativecommons.org/licenses/by/4.0/.
About this article
Cite this article
Hu, W., Zhang, J., Wang, H. et al. Protective effects of isorhamnetin against H2O2-induced oxidative damage in HaCaT cells and comprehensive analysis of key genes. Sci Rep 13, 2498 (2023). https://doi.org/10.1038/s41598-023-27575-7
Received:
Accepted:
Published:
DOI: https://doi.org/10.1038/s41598-023-27575-7
- Springer Nature Limited