Abstract
Some of the anatomical and functional basis of cognitive impairment in multiple sclerosis (MS) currently remains unknown. In particular, there is scarce knowledge about modulations in induced EEG (nonphase activity) for diverse frequency bands related to attentional deficits in this pathology. The present study analyzes phase and nonphase alpha and gamma modulations in 26 remitting-relapsing multiple sclerosis patients during their participation in the attention network test compared with twenty-six healthy controls (HCs) matched in sociodemographic variables. Behavioral results showed that the MS group exhibited general slowing, suggesting impairment in alerting and orienting networks, as has been previously described in other studies. Time–frequency analysis of EEG revealed that the gamma band was related to the spatial translation of the attentional focus, and the alpha band seemed to be related to the expectancy mechanisms and cognitive processing of the target. Moreover, phase and nonphase modulations differed in their psychophysiological roles and were affected differently in the MS and HC groups. In summary, nonphase modulations can unveil hidden cognitive mechanisms for phase analysis and complete our knowledge of the neural basis of cognitive impairment in multiple sclerosis pathology.
Similar content being viewed by others
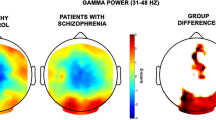
Introduction
Multiple sclerosis (MS) is a chronic degenerative neurological disease of unknown etiology that causes mainly neurological damage including demyelination and inflammation of the central nervous system (CNS)1. The onset of MS occurs over a wide age range (mainly between 20 and 40 years), and MS predominantly affects women. Approximately 40–70% of patients with the disease show diverse cognitive deficits1,2,3. Therefore, different neuropsychological profiles can be found during clinical assessments. Attention, processing speed and memory are the cognitive domains most frequently affected4,5,6,7. To understand the neural basis of these cognitive alterations in MS, different cognitive paradigms and techniques have been used8,9,10.
One of the cognitive paradigms that has often been used to evaluate attention is the “Attention Network Test” (ANT). The ANT was developed to assess three attentional networks (alerting, orienting and executive systems) and has been performed with different versions and for diverse pathologies11,12,13,14.
Although the ANT was developed in 200115, it did not begin to be applied in patients with multiple sclerosis until 201016. These authors found an alteration in patients’ alerting network with respect to healthy controls (HCs). The authors suggested that the deficit could be due to lower response readiness or a reduced ability of patients to maintain alertness after presentation of the cue. Other authors have confirmed the alteration in the alerting network in patients with multiple sclerosis even in early stages of the disease17. Moreover, a study18 found deficits in the alerting and orienting networks in patients based not only on behavioral responses but also on specific psychophysiological variables (e.g., changes in the amplitude of the contingent negative variation).
One way to improve our knowledge of the neural basis of cognitive impairment in MS pathology is to analyze the frequency domain of the EEG signal. In its origins, the EEG signal was largely studied using fast Fourier transformation (FFT), but this approach has the disadvantage of renouncing the high EEG time resolution19,20. Time–frequency EEG methods such as temporal spectral evolution (TSE) can overcome this limit21. TSE shows EEG time–frequency information that includes both phase (evoked) and nonphase (induced) activity related to stimulus presentation (for a detailed description of the TSE method22,23).
Through TSE and other time–frequency techniques, numerous studies have related frequency bands of EEG to specific cognitive mechanisms. In the case of the alpha band, originally, it was identified as an indicator of a neural area at rest24. However, some authors have argued that it may not exclusively reflect an idling status but can also play an active role in inhibitory control and timing of sensory processing25. Along this line, some authors have suggested that an increase in alpha activity in regions irrelevant to the task may reflect inhibition processes26. Specifically, for attentional visual processing, some studies have observed higher alpha activity in those regions of the occipital cortex where unattended locations are retinotopically represented27,28,29.
For decades, it has been suggested that there are two types of alpha bands, namely, low alpha (8–10.5 Hz) and upper alpha (10.5–13 Hz) bands, with different psychophysiological meanings30. Low alpha bands may be related to a general attention, while upper alpha bands are proposed to be linked to more specific processes such as semantic processing31,32. In fact, the topographical distribution of low alpha bands is usually larger and less specific than that of upper alpha bands, which tends to be more localized in scalp regions33,34.
Another neurophysiological correlate that has been linked to cognitive functioning is the gamma band35,36. The functional roles assigned to this band are more than the visual grouping, as has been reviewed by some authors37. In particular, this neural activity has been related to arousal or attention in humans38,39,40. One of the reasons that this band is suitable for attentional mechanisms is its fine temporal tuning for neuronal firing (10–30 ms time precision)41. Some studies have described the specific involvement of the gamma band in visual attentional tasks41,42. In the study by Gruber et al.41, the authors displayed colored rectangles in the left or right half of the screen, and a cue indicated to the subjects in which visual hemifield they had to shift their attention to detect target stimuli. These authors found an increase in the gamma activity (35–51 Hz) when the attended stimuli were rotating. In the study by Fries et al.42, neurons from cortical area V4 in macaque monkeys exhibited an increase in the gamma band (35–90 Hz) when the experimental subjects were attending to relevant stimuli and ignoring distracters.
With regard to these spectral bands and their relation to cognitive impairment in MS, some studies have described a higher decrement of the alpha band in MS patients reflecting a recruitment of more cognitive resources to perform the task43. A more recent study44 showed that the alpha band is related to attentional mechanisms and processing speed; a smaller decrease in the resting-state alpha band in specific experimental conditions was associated with a poorer behavioral performance.
With regard to the gamma band, a few studies have described alterations in this band in MS patients related to diverse phenomena, such as alterations in cognitive processing in a visual attention task45, a neurophysiological correlate of compensatory mechanisms or cortical excitatory states representative of some phases during the MS course45, or gray matter dysfunction, possibly mediated by GABAergic changes46.
Finally, there is scarce knowledge about the meaning of the spectral modulations observed during the execution of the ANT47,48,49. Authors have described a decrease in the alpha band after the warning signal (alerting effect) and an increase in the gamma content at 200 ms after target onset related to the orienting network47. However, this study did not consider if the modulation was in phase or nonphase to the onset of the stimuli47. In the last years, neuroscientists have focused on this difference and found that both domains (evoked and induced) could represent diverse processes in cognition50,51,52.
Considering all these premises, the main goal of the present study was to analyze the modulations in the alpha and gamma bands (and in both evoked and induced modalities) related to attentional impairment in an MS group assessed by the ANT. Our hypothesis was that behavioral data would show a general slowing in the MS group compared to the healthy group that would be corroborated by the neuropsychological assessment. Alpha and gamma modulations related to attentional mechanisms would show alterations in the MS group during the execution of the ANT. An open question in the present study was which components of the alpha and gamma modulations (evoked, induced or both) would be related to attentional impairment.
Results
Behavioral data
The HCs obtained a significantly shorter reaction time than the MS group [F (1,50) = 26,55; p < 0.001; η2 = 0.438]. In addition, the cue factor revealed differences between cues, with the no cue (NC) condition showing larger reaction times followed by the central cue (CC) condition and finally the spatial cue (SC) condition, which was the fastest [F (2,100) = 287.04; p < 0.001; η2 = 0.930] (Table 1and supplementary Table 1 for statistical results). Post hoc Bonferroni comparisons confirmed the differences between all cue levels (p < 0.001).
After applying the correction of Fernández-Duque and Black53, differences in the NC condition were observed between groups [t = 2.186; p = 0.03], and patients were faster than HCs. This value does not indicate that MS patients were faster than HC subjects in this condition; it shows that MS patients were relatively faster for this condition than in all conditions in the MS group in the same comparison for HCs.
With regard to precision, there were no significant differences between groups or other factors because of a similar high percentage of accuracy for all conditions in both experimental groups (Table 1).
Neuropsychological and depression assessments
In terms of the number of hits on the PASAT-3s and the overall hit rate on the symbol digit modality test (SDMT), the latter showed a value of 2 SD under the cutoff scores defined by Sepulcre54, indicating a moderate attentional impairment in our sample of MS patients (SDMT score = 44 ± 13.82). Last, Beck depression scores (BDI-II) indicated that there were not severe signs of depression in the MS group (BDI-II score = 7.44 ± 6.3).
Gamma activity
Expectancy interval
The evoked gamma activity showed no differences in latency after presentation of an SC between both groups (Fig. 1).There were no appreciable modulations for the NC and CC conditions to calculate the latency. With regard to the amplitude, the evoked gamma activity showed no differences after presentation of any type of cue (NC, CC or SC) or between both groups (Fig. 1 and Table 2).
(a) Evoked and induced waves for the gamma band (30–45 Hz) in the expectancy and target response intervals. (b) 2-D head maps for the peak and valley latencies of evoked and induced activity. Abbreviations: MS: multiple sclerosis; HC: healthy controls; CC: central cue; SC: spatial cue; EXP: expectancy interval; ms: milliseconds.
With regard to the induced activity, peak latency analysis of the SC conditions showed no differences between groups (Table 2).In the case of the amplitude analysis, the cue factor was statistically significant (F(2,100) = 53.89, p < 0.001, ƞ2: 0.666). However, a post hoc comparison showed no significant differences between the levels of this main factor. Nevertheless, a post hoc comparison for the following interaction “cue × anterior–posterior location and medial–lateral location” showed that the SC was higher in amplitude than theNC and CC conditions for all derivations (p < 0.001 in all cases) (Fig. 1 and and supplementary Table 2 for amplitude values).
Group factor also showed a significant result (F(1,50) = 4.51, p < 0.038, ƞ2: 0.136) that was caused by a higher amplitude of the gamma modulation in the MS group than in the HC group (Fig. 1 and Table 2). No significant differences were found for the group factor in terms of its interaction with location factors.
Target interval
In contrast to the almost nonexistent response for the cue, the evoked gamma activity for target stimuli was mainly observed for the NC and CC conditions. The latency variable was not different between cue conditions; however, the amplitude exhibited a statistically significant result for the cue factor (F(2,100) = 6.42, p = 0.002, ƞ2: 0.204) (Fig. 1 and Table 2). In a post hoc analysis, no differences were found between the levels of this main factor; however, a post hoc comparison for the interaction “cue × anterior–posterior × lateral-medial” factors revealed that all derivations exhibited a lower amplitude for the SC than for the NC or CC conditions. No significant differences were found between the NC and CC conditions (Fig. 1). Neither group nor an interaction of this factor with location factors resulted in a statistically significant difference.
With regard to the induced activity, latency did not show differences for cue or group factors. For the amplitude variable, ANOVA showed that the “cue × anterior–posterior × lateral-medial” interaction was statistically significant (F(24,1200) = 1.80, p < 0.009, ƞ2: 0.068). Post hoc comparisons showed that all derivations were higher in amplitude for the NC and CC conditions than for the SC condition (p < 0.001 in all cases).
Group factor also showed a statistically significant result (F(1,50) = 6.24, p = 0.015, ƞ2: 0.177), with MS patients exhibiting a higher amplitude than the healthy group (Fig. 1 and Table 2). No significant differences were found for the group factor in its interaction with other location factors.
Low (8–10.5 Hz) and upper (10.5–13 Hz) alpha bands
Expectancy interval
In the evoked activity latency analysis, no significant differences between groups were present either in the low or upper alpha bands (Fig. 2, 3). For the amplitude variable, HCs showed larger values than patients in both the low alpha [F (1,50) = 7.1205; p = 0.010; η2 = 0.237] and upper alpha bands [F (1,50) = 4.4794; p = 0.039; η2 = 0.164] (Figs. 2, 3) (Tables 3, 4 for amplitude and latency values). Despite the differences in the amplitude in both bands, there were no differences in their topographical distributions between groups (interactions for group and location factors).
(a) Evoked and induced waves for the low alpha band (8–10.5 Hz) in the expectancy and target response intervals. (b) 2-D head maps for the peak and valley latencies of evoked and induced activity.Abbreviations: MS: multiple sclerosis; HC: healthy controls; NC: no cue; CC: Central cue; SC: spatial cue; ms: milliseconds.
(a) Evoked and induced waves for the upper alpha band (10.5–13 Hz) in the expectancy and target response intervals. (b) 2-D head maps for the peak and valley latencies of evoked and induced activity. Abbreviations: MS: multiple sclerosis; HC: healthy controls; NC: no cue; CC: central cue; SC: spatial cue; ms: milliseconds.
Regarding induced activity, the latency in which the maximum amplitude was reached was different for each cue condition (Figs. 2, 3). This difference for the cue factor was observed both in the low alpha [F (1.50) = 0.77980; p < 0.001; η2 = 0.714] and upper alpha bands [F (1.50) = 6.3649; p = 0.014; η2 = 0.773]. A post-hoc Bonferroni comparison showed that the SC condition reached the maximum amplitude later than the CC condition (in both the low and upper alpha bands, p < 0.001) (Tables 3, 4 for latency values).
Despite the larger amplitude observed in the control group (Figs. 2, 3), no statistically significant differences for group factor were observed throughout the expectancy interval divided into two time intervals (0–350 ms and 350–700 ms) or in its interaction with other location factors (Tables 3, 4 for mean amplitude values).
Target interval
Evoked latency showed no significant differences between the two groups in the low alpha and upper alpha subbands (Figs. 2, 3). HCs showed a larger amplitude than MS patients in both the low alpha [F (1.50) = 8.4645; p = 0.005; η2 = 0.242] and upper alpha bands [F (1.50) = 4.5503; p = 0.037; η2 = 0.125] (Figs. 2, 3 and Tables 3, 4 for amplitude and latency values). There were no differences in topographical distribution between groups.
In the induced latency analysis, the interaction of group and cue factors was significant in both the low and upper alpha bands [F (2.204) = 81.212; p < 0.001; η2 = 0.599] [F (2.204) = 87.305; p < 0.001; η2 = 0.644] (Figs. 2, 3).A post-hoc Bonferroni comparison showed that MS patients in the NC and CC conditions reached the maximum amplitude later than controls in both alpha subbands (p < 0.001). However, in the SC condition, there were no differences between groups in the latency in the low alpha or upper alpha bands (Figs. 2, 3).
With regard to the amplitude variable for induced modulation, no differences in topographical distribution were found for the group factor or its interaction with other location factors in either low alpha or upper alpha bands or in any time interval analyzed (0–350 ms and 350–700 ms) (Figs. 2, 3 and Tables 3, 4 for mean amplitude and latency values).
Phase analysis for induced and evoked activity
After phase analyses were made for the evoked and induced activities in the frequency bands (low and upper alpha and gamma bands) and for both groups, it was possible to conclude that the potential contribution of the evoked activity over induced activity could be discarded in all bands, as has been described in previous studies52,55. Figure 4 shows that the phase values of induced activity for HCs in the lower and upper alpha subbands and gamma band for the target stimuli following a central cue showed random values that were not concentrated in the phase value of the evoked activity. Similar results were observed in other cue conditions and for the MS group (see Figs. 1–7 in supplementary material).
Plotting of phase values in the lower and upper alpha and gamma bands for evoked and induced activities and in the target response interval following the presentation of a central cue and for each healthy control subject (26 subjects). Evoked activity is represented by red, yellow and green dots, and induced activity is displayed with green, blue and red crosses for lower alpha (8–10.5 Hz), upper alpha (10.5–13 Hz) and gamma (30–45 Hz) bands, respectively.
Discussion
The behavioral results suggested an attentional impairment in the MS group. A general slowing was observed (approximately 100 ms) for MS patients in all cue conditions. Moreover, the delay in reaction times was not caused by a speed-accuracy tradeoff between experimental conditions for the patient group compared with the HC group. In addition, the neuropsychological assessment revealed an attentional impairment that was not caused by severe signs of depression (low scores on the BDI).
A delay in the reaction time for attentional tasks in MS patients has been described in previous studies18,56,57,58. With regard to the neural networks, the slower reaction times in the NC and CC conditions suggest that alerting mechanisms (phasic or tonic) could be impaired, as has been suggested by other studies16,17,18. Moreover, a longer reaction time in the SC condition also indicates that the orienting mechanism could also be affected, as previously described18.
However, it seems unreasonable that such a common delay in all cue conditions (100 ms) could be due to multiple and diverse impairments such as the phasic and tonic alerting or orientation mechanisms. It is not possible with the current results to discard that a common mechanism operating in all conditions could be the reason for the general slowing in the MS sample.
A relevant result to better understand the attention impairment in the MS group comes from the data after the correction of Fernandez-Duque and Black53. A statistical t test group comparison in all cue conditions (e.g., NC MS vs NC HC) showed that the NC condition was responded to faster in the internal comparisons for MS patients than the same comparison in the HC group. This result suggests that MS patients try to be more ready for uncued targets that are more demanding in terms of response time. This approach could be a strategic compensation from MS patients to reduce the average reaction time for all the experimental conditions. However, a more intricate interpretation is suggested from neurophysiological measures, which will be described in the following paragraphs.
Regarding the induced activity, the gamma band seems to be related to the translation of the attentional focus towards the cued visual spatial locations. The only condition in the expectancy interval that showed an increase in the gamma amplitude was the SC condition during which subjects were required to move their attentional focus to specific spatial regions indicated by cues (100% valid). On the other hand, during the NC or CC conditions, the attentional focus is maintained around a fixation point; therefore, no increase in the gamma activity is observed.
In the case of the induced gamma response for target stimuli, the NC and CC conditions need an orienting response provoked by the onset of the stimuli and consequently a shifting of the attention focus towards it. This shift is translated as an increase in the amplitude of the gamma activity as it occurred in the SC condition for the cue onset. In the case of the target preceded by the SC condition, a small increase in the gamma response could represent a lower need to readjust the attentional focus precisely over the central arrow to avoid competition from distractor stimuli.
These results are in accordance with those of a previous study that showed that the orientation mechanism modulates gamma activity (without a specific definition of evoked or induced activity) in the first 200 ms after the onset of the target47. Gamma band activity has been associated with an attentional mechanism involved in optimal performance in cognitive tasks37, 39.
With regard to the group variable, a first consideration is that the peak latencies of the evoked or induced gamma activities were not different between both groups. It is well known that MS pathology usually implies delays in the timing of cognitive processes caused by the demyelination process59,60,61. However, there have been diverse studies that have not found latency delays in behavioral or EEG parameters in MS patients during the execution of cognitive tasks20,62.
At the same time, the amplitude for induced gamma activity was higher for MS subjects than for the HC group. It is counterintuitive that the MS group exhibited a higher amplitude than and a similar latency to the HC group. However, if the functional meaning of the induced gamma modulation represents the translation of the attentional focus in the visual field, a possible interpretation is that the ability of the patients to adequately keep this focus in an appropriate location (e.g., on the fixation point) is impaired, and constant reorienting processes are needed with a consequent increase in the gamma amplitude. In this case, the gamma band reflects a compensatory mechanism rather than an impaired one.
A corollary of this interpretation is that MS patients probably need to increase their engagement of alerting and orienting mechanisms to improve their reaction in each trial to relocate their attentional focus as fast as possible. The maintenance of this strategy to compensate for the deficit is probably the cause of cognitive fatigue observed in some cases among MS patients during sustained attention tasks63,64.
Finally, it is necessary to comment about the difference between evoked and induced gamma activity. Notably, evoked gamma modulation was mainly present for the target stimuli, and induced activity was linked to both stimuli (cue and target). It could be suggested that evoked activity is only related to the relevant stimuli that require a motor response; in contrast, induced activity does not depend on that feature. Unfortunately, the present experiment does not allow further assessment of this issue, and future studies are necessary to disentangle the precise functional role of both gamma modulations in this respect.
With regard to evoked activity that may mainly represent the alpha content of the early ERPs (P1 and N1), there was no difference between groups for the latency parameter. Therefore, it could be suggested that the described slowing in the latency of early ERPsin MS patients18,58,65,66 due to the demyelination was not observed in our data. However, other studies did not find differences in early visual ERP latency between HCs and patients with MS67.
On the other hand, with regard to the amplitude parameter, previous studies have found lower amplitudes in the P1 component68 and N1-P2 complex69,70among MS patients. Our results are in agreement with these studies in terms of both the cue and target early evoked responses. This decrement in the amplitude could reflect that a lower number of neurons were synchronized to elicit the evoked response but at the same time preserved their connectivity, which maintained the appropriate latency. Other authors have interpreted similar results as an impaired mechanism of synchrony in the neural generators of the early visual processing in MS group71.
With regard to the induced alpha activity, a clear negative trend was observed in the CC and SC conditions in the expectancy interval. It has been suggested that the onset of alpha desynchronization starting at approximately 300 ms is a sign that the influence of the alpha band on cortical activity was disappearing and that other task-relevant processes/bands took place25. This reduction of alpha synchronization observed in our study could also be in accordance with the findings of previous studies in which a decreased alpha band was linked to anticipatory attention (orienting network) with respect to the arrival of the target in a cue paradigm26.
In the particular case of alpha modulations during the ANT, a study found a decrease in alpha activity (not distinguished between phase or nonphase) related to the alerting network from 200 to 450 ms after the onset of the cue47. These authors indicated that this desynchronization could be related to the abrupt onset of the cue that engages an alerting response. However, in our study, the cue factor results from ANOVA indicated that the CC condition reached its highest value before the SC condition. We suggest that the reason for this difference may be the different information content involved in the two cues. The CC condition provides timing information, while the SC condition adds spatial information to the timing expectancy. The more information is added, the more latency is necessary to reach the maximum desynchronization. Previous studies have demonstrated in the time domain of EEG [contingent negative variation (CNV)] that the more spatial and timing information was given in the cue, the higher the amplitude of the CNV72. However, the CNV trend is maintained until the target stimuli are presented (at least in a one-second SOA), but induced alpha modulation reaches maximum negativity and starts recovering to the baseline level before the target appears. This last modulation seems to be more related to a specific mechanism in a determined time interval than to a sustained preparatory state for incoming stimuli.
This difference between the CC and SC conditions observed in both alpha bands (low and upper) could indicate that both general (alerting) and specific (orienting) attentional mechanisms are recruited in the expectancy interval. In particular, some authors have suggested that the decrease in the upper alpha band may be related to anticipatory attention26or maintaining attentional focus73.
With regard to the comparison between both experimental groups, no differences were found in the latency or amplitude. These results suggest that expectancy mechanisms indexed by alpha-induced activity seem preserved in MS patients. A previous study in our laboratory with the same cognitive task demonstrates that the expectancy mechanisms related to the CNV showed alterations in MS patients18, which supports that evoked activity and induced activities, can be disentangled and represent different cognitive mechanisms. Moreover, phase analyses showed that single trial-induced phases displayed random values that were not concentrated in the evoked phase values (Fig. 4 and supplementary material Fig. 1). These results suggest that it is possible to discard the potential contribution of the evoked activity over the induced activity in terms of amplitude modulation. Therefore, the induced activity is truly non-phase-locked activity and is not caused by the jittering of the evoked activity. A similar result was obtained in our laboratory during the performance of an oddball task52.
In the target response interval, alpha-evoked activity showed no differences in the latencies of the responses for the target in all cue conditions (NC, CC and SC). On the other hand, the amplitude showed a reduction in the MS group suggesting an impaired processing of the target stimuli. Some studies have reported that the early components (P1/N1) in a cueing paradigm are enhanced for the target stimuli as the result of sensory preparation74,75,76. However, in the present study, it is not possible to determine if the decrement was caused by an impaired preparatory mechanisms in MS patients (not shown in the current experiment but demonstrated in previous studies18) or was due to a reduced number of neurons operating as it was suggested for the cue processing. Further research is needed to clarify the precise factors involved in the decrement of early evoked activity in MS pathology.
With regard to the induced activity, different modulations were found for target stimuli depending on the preceding cue. In the NC and CC conditions, there was no difference in the amplitude between experimental groups. However, an increase in the latency of the maximum negativity for both cue conditions was observed in the MS group. It seems that patients performed a first alpha desynchronization in the first valley (0–350 ms) but not well enough in terms of efficient cognitive processing (lower amplitude) and rebounded with a second valley that reached a better level of alpha desynchronization.
Moreover, the SC condition did not show a statistically significant delay for MS patients, with a slightly higher amplitude in the first than in the second valley. However, the presence of a second valley can be observed in the SC condition for MS patients compared with controls, suggesting that a rebound could be present in some trials. These rebounds in the decrease in the alpha modulation could reflect an effort from MS patients to compensate for cognitive impairment, as has been suggested in previous studies43.
In summary, the current behavioral results supported previous studies16,17,18 that suggested an impairment in the alerting and orienting networks in MS patients. However, diverse neurophysiological parameters suggest a more complex interpretation of the causes for cognitive impairment in this MS sample.
Our hypothesis after the analysis of the current experiment is that MS patients exhibited an impairment to maintain attentional focus during the experiment, as has been suggested in previous studies73. First, this hypothesis could explain why the evoked activity for cue and target stimuli is reduced considering that MS patients are not placing their attentional focus precisely in the relevant locations for the experiment. Second, an increased induced gamma modulation in MS patients could reflect a higher need of reorienting in both cue and target presentations. However, the latency of the gamma peak was not delayed in the MS group, which suggests that the relocation of the spatial attentional focus is not enough to justify the delay in behavioral responses.
This last evidence leads us to consider that the impairment in the maintenance of attentional focus not only involves its spatial properties but also involves its object properties. Multiple studies have analyzed the interaction between both determinants of the stimuli (spatial and object features) and described its neural sources77,78,79. Our conclusion is that MS patients have difficulties in maintaining an appropriate “spatial-object” attentional focus. As it has been pointed out in a study with ADHD children80, an impaired attentional performance is not caused necessarily by the orienting process itself but by the difficulty in maintaining the relevant information demanded for the task (spatial and object properties) during the preparation interval and until the target processing stage. The maintenance of an attentional focus to perform the ANT could also be related to working memory mechanisms81,82,83. Considering this link between the two processes, diverse studies have described working memory impairments in cognitive tasks in patients with MS83,84,85.
The correlate for the difficulty in the processing of object properties of the target could be represented by the rebounds in the induced alpha modulation. However, this impaired mechanism is a consequence of the poor maintenance of the spatial-object attentional focus in MS patients. The precise neurophysiological parameter for the specific impairment in the maintenance of the spatial-object attentional focus has not been found in the present experiment and future studies are needed for its identification.
In any case, the study of the nonphase activity in EEG signals has exhibited hidden cognitive mechanisms (compensatory and impaired) for the traditional “evoked studies” that can complete our knowledge of the neural basis of cognitive impairment in MS patients and other pathologies.
Methods
Participants
Twenty-six patients with a relapsing–remitting MS (RRMS) form were recruited in the Multiple Sclerosis Unit of Hospital Universitario Virgen Macarena (Seville, Spain). The definitive diagnosis was made by a neurologist, according to McDonald's criteria85. To be eligible for inclusion in the study, patients were required to be under an EDSS of 6 (mean 2.4, SD 1.5) and avoid exclusion conditions: clinical relapses in the last month; presence of comorbid neurodegenerative or psychiatric disorders; severe signs of depression; history of substance abuse; head trauma; vascular diseases and seizures; significant upper limb impairment; or visual acuity or field deficits.
The MS sample was formed by 16 women and 10 men, with an age range of 29–45 years (mean 34.42, SD 7.07); one of the patients was left-handed. An HC group was selected with sociodemographic variables matched to those off the MS group (11 women and 15 men who were between the ages of 22–52 years (mean 30.30, SD 9.30); one of the HCs was left-handed. All the healthy subjects were declared to be free of neurological conditions.
This study was carried out in compliance with the Helsinki Declaration. All participants signed informed consent before their inclusion, and the protocol was approved by the Ethics Committee of the University of Seville (project code: PSI2016-78,133-P).
Neuropsychological and depression assessments
Neuropsychological and depression assessments of the patients were performed by well-trained psychologists blinded to the study goals. The attention and speed of information processing were evaluated through the Paced Auditory Serial Addition Test, 3s (PASAT-3s)86,87 and the Symbol Digit Modality Test (SDMT)88,89. Cognitive impairment was identified using the normative scores developed by Sepulcre54. The Beck Depression Inventory (BDI-II)90,91 was used to assess symptoms of depression.
Cognitive task
Participants were seated in a sound-attenuated room in front of a computer monitor. Stimuli were created by E-prime 2.0 (Psychology Software Tools, Inc. Pittsburgh, PA) and presented on an LCD screen. An adaptation of the ANT was used for this study11 (Fig. 5A).Both cue and target stimuli were presented on a black background on which a fixation cross was projected in the middle of the screen throughout the experiment. The task consisted of two intervals (defined by the onset of the cue and target), and the subject only had to respond to the target stimuli. During the expectancy interval (between the cue and target), there were 3 possible cue conditions. The no cue condition (NC) consisted only of the presentation of the fixation cross in the middle of the screen and a delayed presentation of the target stimulus. The central cue (CC) condition involved an asterisk (cue) being presented in the middle of the screen and providing the subject with a temporal clue about the timing (because of a fixed SOA, 1 s) of the onset of the target. The last condition was the spatial cue (SC) condition, in which the asterisk appeared above or below the fixation cross, providing both clues about the timing of the target stimulus presentation and spatial location (cues were 100% valid). All cue conditions appeared in the same proportion. The cues were presented with a visual angle of 0.41 on the × axis and 0.41 on the Y axis.
In the target response interval, the participant had to respond to stimuli formed by 5 arrows pointing to the left or right side. The presented stimuli could be congruent (C) when the central arrow (target) was oriented in the same direction as the flankers or incongruent (I) when the flanker arrows pointed to the opposite direction of the central arrow. The target stimulus was presented at a total visual angle of 3.28 on the X-axis and 0.41 on the Y-axis and 0.86 above or below the fixation cross. Congruent and incongruent stimuli were presented in equal proportions. Furthermore, half of the targets pointed to the right and half to the left. The subject was required to respond according to the direction of the central arrow by pressing the left button with the left thumb when the central arrow pointed to the left or by pressing the right button with the right thumb when the central arrow pointed to the right.
The timing for the presentation of stimuli was modified from the original ones11 to adapt it for MS patients. The cue duration was 150 ms, and the target stimulation presentation lasted 350 ms. The time between the end of the cue and onset of the target was 1000 ms. Participants had 1000 ms to respond from the onset of the target stimulus. The duration between the end of one trial and the next one was variable (1000, 1500 or 2000 ms). The experiment was divided into two blocks of 144 tests each, for 288 in total. A pause between blocks was proportionate to avoid fatigue in participants. The duration of this pause was defined by the need of the participant but usually did not last for more than two minutes. The total duration of the experimental procedure with an average pause block of two minutes was approximately of 15 min. The presentation of the trials (cue and target pairs) was set in a pseudorandom order.
Reaction times and precision percentages were calculated for all conditions. All participants were instructed to respond as quickly and accurately as possible. To analyze differences between groups in specific conditions, a potential general slowing for reaction time in MS patients was corrected following the recommendations of Fernandez-Duque and Black53. No network effects were calculated because diverse studies have concluded that thesevalues can induce erroneous interpretations of the data72,92.
EEG recording and analyses
EEG data were recorded from 58 electrodes (Ag/AgCl) in standard locations of a 10–10 system93and amplified with BrainAmp amplifiers (Brain Products GmbH, Germany) (Fig. 5B, 5C for detailed positions of electrodes). The EEG signal was filtered online with a bandpass of 0.01 to 100 Hz, digitized with a sampling rate of 500 Hz and stored using Brain Vision Recorder software (Brain Products GmbH, Germany). These continuous data were referenced online to the linked auricular lobes and offline to a common averaged reference. Impedance was kept below 5 kOhm during the experiment. Vertical and horizontal electro-oculograms (VEOG and HEOG) were also recorded with a bipolar montage. Trials with an HEOG signal outside the ± 50 µV range were rejected. For blinking artifacts, ocular correction was performed in the scalp electrodes using the algorithm developed by Gratton et al.94. A continuous signal was epoched in segments of − 200 to 1000 ms, with zero being the onset of the cue or target stimuli. A baseline correction (− 100 to 0 ms) was also applied to all conditions.
After all this processing, two possible analyses were made for the EEG signal: to obtain evoked activity, all conditions (NC, CC and SC, for both cue and target stimuli) were averaged independently, filtered for low (8–10.5 Hz) and upper (10.5–13 Hz) alpha and gamma bands (30–45 Hz) (in all cases, 48 dB/octave, Butterworth) and rectified52.In the case of the induced activity, a temporal spectral evolution (TSE) was calculated with the following steps: identical bandpass filtering in previously defined low and upper alpha and gamma bands performed over EEG epochs, rectifying the signal and finally averaging for all stimuli conditions. After this analysis, a subtraction of evoked activity from the TSE was subsequently performed to calculate the induced response (nonphase activity)51 (see Fig. 6).
To analyze the latency of all band modulations as suggested by Keil95, latency was defined at the electrode with the maximum amplitude observed in a grand average of all experimental conditions and performed for each group. Following these criteria, the evoked gamma activity in the expectancy interval was almost nonexistent in all cue conditions, and induced activity was mainly present in the SC condition and for the interval of 210–225 ms. In the case of the target stimuli, the peaks of the evoked and induced gamma activity occurred in the interval of 175 to 245 ms. Once the intervals were set, calculations of gamma peak latencies were made for each participant. Moreover, the mean amplitude value was exported for the entire intervals defined previously in a matrix of 3 × 7 electrodes that covered the posterior area of the scalp where gamma activity was prominent (Fig. 5C).
With respect to the low and upper alpha subbands, the evoked activity was analyzed in both expectancy and target response intervals for the 0–350-ms interval. The latency peak was determined in this interval individually for each participant. Amplitude analyses were performed with mean values in the 0–350 ms and for a matrix of 6 × 7 electrodes in the expectancy interval and a 3 × 7 matrix in the target response interval.
With regard to the induced activity, the valley latency value was identified as the maximum negativity present in any of the intervals analyzed (0–350 ms; 350–700 ms). Individual values were calculated for each subject of both experimental groups. Amplitude studies were performed as for the evoked activity but in two different time intervals (0–350 ms; 350–700 ms).
Phase analysis for evoked and induced activity
Because of a potential contribution of evoked over induced activity could occur (especially in the target response), the induced modulation was checked to ensure that it was truly nonphase locked activity for all bands studied (low/upper alpha and gamma bands). To achieve this goal, the evoked response was estimated through averaging over trials and then subtracted from each of the individual trials55,96. To do so, the trials were filtered in the desired bands (low alpha: 8–10.5 Hz; upper alpha: 10.5–13 Hz; gamma: 30–45 Hz, in all cases, 48 dB/octave, Butterworth), and the Hilbert transform was applied to calculate instantaneous phase.
The phases of gamma activity were measured at the interval of 210–225 ms (expectancy interval) and 175–245 ms (target response interval) in single trials, which corresponds to the estimated grand average peak interval of the induced response. In the case of the alpha bands, phase calculations were performed in 0–350 ms for low and upper alpha subbands following the same protocol described for the gamma band. Last, the phases of the evoked responses (alpha and gamma) were calculated by following the same protocol performed for the induced activities and in the same time intervals.
Statistical analyses
Behavioral responses
A previous exploratory analysis of the data revealed that there were no differences by the “congruency” variable or in its interaction with the experimental group for alpha or gamma modulations. For this reason and to facilitate the following analyses, we collapsed congruent and incongruent values for each cue condition.
By means of the Shapiro–Wilk test (p > 0.05), all variables were checked for normality. Parametric (ANOVA) or nonparametric tests (Kruskal–Wallis) were performed to study possible differences in behavioral and neurophysiological variables where appropriate.
The analysis of the reaction times was carried out with a two-factor ANOVA: group factor (levels: HC and MS patients) and a cue factor (levels: no cue, central cue and spatial cue). After correction suggested by Fernández-Duque and Black53, the t test was performed to check whether both groups responded differently to the cues (paired comparisons for each cue condition value). The accuracy of the subjects' responses was analyzed applying the Kruskal–Wallis test with the same factors as for the reaction time ANOVA.
Gamma band
To analyze the latency of evoked and induced gamma activities in the expectancy interval, we performed two independents ANOVAs to compare both experimental groups only for the SC condition. For the amplitude variable, we performed two ANOVAs (evoked and induced) with the following factors and levels: group factor (levels: HC and MS patients); cue factor (levels: NC, CC and SC); anterior–posterior location factor (levels: mid-parietal, parietal and parietal-posterior); and medial–lateral location factor (levels: line 5, line 3, line 1, central, line 2, line 4, line 6).
In the target response interval, evoked and induced gamma latencies were analyzed separately by ANOVAs including all cue conditions (NC, CC and SC) and for both experimental groups. The amplitude variable was studied with the same factors as described for the expectancy interval.
Low and upper alpha subbands
In the case of the analysis of alpha activity (both subbands), in the expectancy interval, evoked and induced latencies were analyzed separately. The two ANOVAs performed had two main factors: “group” (levels: HC and MS patients) and “cue” (levels: CC and SC). The NC condition was not introduced because of a null response of evoked and induced activity due to the absence of cue stimulation. With regard to topographic study, the evoked amplitude was analyzed with an ANOVA with the following factors: group (levels: HC and MS patients); cue factor (levels: NC, CC and SC); anterior–posterior factor (levels: frontal, frontocentral, central, mid-parietal, parietal and parietal-posterior); and lateral-medial factor (line 5, line 3, line 1, central, line 2, line 4, line 6). In the case of induced activity, two independent ANOVAs were performed for each time interval (0–350; 350–700) with the previously described factors for evoked activity.
In the target response interval, evoked and induced latencies were analyzed separately with the same ANOVA factors as in the expectancy interval, but in this case, all the cues were included in the cue factor (levels: NC, CC and SC). On the other hand, amplitude analysis of evoked and induced modulations was performed with the same factors for the ANOVAs in the expectancy interval but with fewer levels for the anterior–posterior factor (levels: mid-parietal, parietal and parietal-posterior) (matrix 3 × 7).
In all the analyses described above, sphericity was corrected with Greenhouse–Geisser, and a statistically significant result was considered from p < 0.05. A post hoc analysis of all the possible comparisons was carried out using Bonferroni correction.
Data availability
The datasets generated during and/or analyzed during the current study are available from the corresponding author on reasonable request.
References
Calabresi, P.A. In Multiple Sclerosis and Demyelinating Conditions of the Central Nervous System (eds Goldman, L. & Schafer, A.) Cap 11 (Elsevier Saunders, 2016).
Chiaravalloti, N. D. & DeLuca, J. Cognitive impairment in multiple sclerosis. Lancet Neurol. 7(12), 1139–1151 (2008).
Fabian, M.T., Krieger, S.C. & Lublin, F.D. In Multiple Sclerosis and Other Inflammatory Demyelinating Diseases of the Central Nervous System (eds. Daroff, R.B., Jankovic, J., Mazziotta, J.C. & Pomeroy, S.L.) Cap. 80 (Elsevier, 2016).
Paul, R. H., Beatty, W. W., Schneider, R., Blanco, C. & Hames, K. Impairments of attention in individuals with multiple sclerosis. Mult. Scler. 4(5), 433–439 (1998).
Prakash, R. S., Snook, E. M., Lewis, J. M., Motl, R. W. & Kramer, A. F. Cognitive impairments in relapsing-remitting multiple sclerosis: A meta-analysis. Mult. Scler. 14(9), 1250–1261 (2008).
Benedict, R. H. & Zivadinov, R. Risk factors for and management of cognitive dysfunction in multiple sclerosis. Nat. Rev. Neurol. 7(6), 332–342 (2011).
Vázquez-Marrufo, M., Borges, M. & González-Rosa, J.J. Neuropsicología del deterioro cognitivo en esclerosis múltiple (Síntesis, 2018).
Leocani, L. et al. Electroencephalography coherence analysis in multiple sclerosis: Correlation with clinical, neuropsychological and MRI findings. J. Neurol. Neurosurg. Psychiatry. 69(2), 192–198 (2000).
Leocani, L. & Comi, G. Neurophysiological markers. Neurol Sci. https://doi.org/10.1007/s10072-008-0942-1 (2008).
Vázquez-Marrufo, M. et al. Altered individual behavioral and EEG parameters are related to the EDSS score in relapsing-remitting multiple sclerosis patients. PLoS ONE 14(7), e0219594 (2019).
Fan, J., McCandliss, B., Sommer, T., Raz, A. & Posner, M. I. Testing the efficiency and independence of attentional networks. J. Cogn. Neurosci. 14(3), 340–347 (2002).
Neuhaus, A. H. et al. Event-related potentials associated with Attention Network Test. Int. J. Psychophysiol. 76, 72–79 (2010).
Martella, D., Casagrande, M. & Lupiáñez, J. Alerting, orienting and executive control: The effects of sleep deprivation on attentional networks. Exp Brain Res. 210, 81–89 (2011).
Spagna, A., Martella, D., Fuentes, L. J., Marotta, A. & Casagrande, M. Hemispheric modulations of the attentional networks. Brain Cogn. 108, 73–80 (2016).
Fan, J., Wu, Y., Fossella, J. A. & Posner, M. I. Assessing the heritability of attentional networks. BMC Neurosci. 2, 14 (2001).
Urbanek, C. et al. Attention Network Test reveals alerting network dysfunction in multiple sclerosis. Mult Scler. 16(1), 93–99 (2010).
Crivelli, L. et al. Alerting network dysfunction in early Multiple Sclerosis. J. Int. Neuropsychol. Soc. 18, 757–763 (2012).
Vázquez-Marrufo, M. et al. Neural correlates of alerting and orienting impairment in multiple sclerosis patients. PLoS ONE 9(5), 1–10 (2014).
Ray, W. J. & Cole, H. W. EEG activity during cognitive processing: Influence of attentional factors. Int. J. Psychophysiol. 3(1), 43–48 (1985).
Vázquez-Marrufo, M. et al. Abnormal ERPs and high frequency bands power in multiple sclerosis. Int. J. Neurosci. 118(1), 27–38 (2008).
Salmelin, R. Y. & Hari, R. Spatiotemporal characteristics of sensoriomotor neuromagnetic rhythms related to thumb movement. Neuroscience 60(2), 537–550 (1994).
Lehtela, L., Salmelin, R. & Hari, R. Evidence for reactive magnetic 10-Hz rhythm in the human auditory cortex. Neurosci. Lett. 222, 111–114 (1997).
Vázquez-Marrufo, M., Vaquero, E., Cardoso, M. J. & Gómez, C. M. Temporal spectral evolution of electroencephalogram. Methodology and applications. Rev Neurol. 33(6), 525–530 (2001).
Pfurtscheller, G., Maresh, H. & Schuy,. Inter- and intrahemispheric differences in the peak frequency of rhythmic within the alpha band. Electroencephalogr. Clin. Neurophysiol. 42(2), 77–83 (1977).
Klimesch, W., Sauseng, P. & Hanslmayr, S. EEG alpha oscillations: The inhibition-timing hypothesis. Brain Res. Rev. 53, 63–88 (2007).
Romei, V., Gross, J. & Thut, G. Sounds reset rhythms of visual cortex and corresponding human visual perception. Curr. Biol. 22, 807–813 (2012).
Worden, M. S., Foxe, J. J., Wang, N. & Simpson, C. V. Anticipatory biasing of visuospatial attention indexed by retinotopically specific alpha band electroencephalography increases over occipital cortex. J. Neurosci. 20(6), 1–6 (2000).
Vázquez-Marrufo, M., Vaquero, E., Cardoso, M. J. & Gómez, C. M. Temporal evolution of α and β bands during visual spatial attention. Brain Res. Cogn. 12, 315–320 (2001).
Capilla, A., Schoffelen, J. M., Paterson, G., Thut, G. & Gross, J. Dissociated α-band modulations in the dorsal and ventral visual pathways in visuospatial attention and perception. Cereb. Cortex. 24(2), 550–561 (2014).
Klimesch, W. EEG alpha and theta oscillations reflect cognitive and memory performance: A review and analysis. Brain Res. Rev. 29(2–3), 169–195 (1999).
Klimesch, W., Doppelmayr, M., Schimke, H. & Ripper, B. Theta synchronization and alpha desynchronization in memory task. Psychophysiology 34, 169–176 (1997).
Klimesch, W., Russegger, H., Doppelmayr, M. & Pachinger, T. A Method for the calculation of induced band power: Implications for the significance of brain oscillations. Electroencephalogr. Clin. Neurophysiol. 108(2), 123–130 (1998).
Pfurtscheller, G., Neuper, C. & Mohl, W. Event-related desynchronization (ERD) during visual processing. Int. J. Psychophysiol. 16(2–3), 147–153 (1994).
Klimesch, W., Doppelmayr, M., Pachinger, T. & Russegger, H. Event—related desynchronization in the alpha band and the processing of semantic information. Cogn. Brain Res. 6, 83–94 (1997).
Fries, P. Rhythms for cognition: Communication through coherence. Neuron 88(1), 220–235 (2015).
Mably, A. J. & Colgin, L. L. Gamma oscillations in cognitive disorders. Curr. Opin. Neurobiol. 52, 182–187 (2018).
Tallon-Baudry, C. The roles of gamma-band oscillatory synchrony in human visual cognition. Front. Biosci. 14, 321–332 (2009).
Basar, E., Basar-Eroglu, C., Karakas, S. & Schürmann, M. Brain oscillations in perception and memory. Int. J. Psychophysiol. 124, 95–124 (2000).
Gonzalez-Andino, S. L., Michel, C. M., Thut, G., Landis, T. & Grave de Peralta, R. Prediction of response speed by anticipatory high-frequency (gamma band) oscillations in the human brain. Hum. Brain Mapp. 24(1), 50–58 (2005).
Ray, S., Niebur, E., Hsiao, S. S., Sinai, A. & Crone, N. E. High-frequency gamma activity (80–150Hz) is increased in human cortex during selective attention. Clin. Neurophysiol. 119(1), 116–133 (2008).
Gruber, T., Müller, M. M., Keil, A. & Elbert, T. Selective visual-spatial attention alters induced gamma band responses in the human EEG. Clin. Neurophysiol. 110(12), 2074–2085 (1999).
Fries, P., Reynolds, J. H., Rorie, A. E. & Desimone, R. Modulation of oscillatory neuronal synchronization by selective visual attention. Science 291(5508), 1560–1563 (2001).
Kiiski, H. et al. Only low frequency event-related EEG activity is compromised in multiple sclerosis: Insights from an independent component clustering analysis. PLoS ONE 7(9), 1–12 (2012).
Keune, P. et al. Exploring resting-state EEG brain oscillatory activity in relation to cognitive functioning in Multiple Sclerosis. Clin. Neuropsysiol. 128, 1746–1754 (2017).
Vazquez-Marrufo, M. et al. Quantitative electroencephalography reveals different physiological profiles between benign and remitting-relapsing multiple sclerosis patients. BMC Neurol. 8, 44 (2008).
Stickland, R. et al. Coupling during visual stimulation in multiple sclerosis: A MEG-fMRI study. Neuroscience 403, 54–69 (2019).
Fan, J. et al. The relation of brain oscillations to attentional networks. J. Neurosci. 27(3), 6197–6206 (2007).
Deiber, M. P., Ibañez, V., Missonnier, P., Rodriguez, C. & Giannakopoulos, P. Age-associated modulations of cerebral oscillatory patterns related to attention control. Neuroimage. 82, 531–547 (2013).
Hasler, et al. Attention-related EEG markers in adult ADHD. Neuropsychologia 87, 120–133 (2016).
Pitts, M. A., Padwal, J., Fennelly, D., Martínez, A. & Hillyard, S. A. Gamma band activity and the P3 reflect post-perceptual processes, not visual awareness. Neuroimage. 101, 337–350 (2014).
Marshall, T. R., O’Shea, J., Jensen, O. & Bergmann, T. O. Frontal eye fields control attentional modulation of alpha and gamma oscillations in contralateral occipitoparietal cortex. J. Neurosci. 35(4), 1638–1647 (2015).
Vázquez-Marrufo, M., García-Valdecasas, M., Caballero-Díaz, R., Martín-Clemente, R. Y. & Galvao-Carmona, A. Multiple evoked and induced alpha modulations in a visual attention task: Latency, amplitude and topographical profiles. PLoS One 14(9), e0223055 (2019).
Fernández-Duque, D. & Black, S. E. Attentional networks in normal aging and Alzheimer’s disease. Neuropsychology. 20(2), 133–143 (2006).
Sepulcre, J. et al. Cognitive impairment in patients with multiple sclerosis using the Brief Reatable Battery-Neuropsychology test. Mult. Scler. 12(2), 187–195 (2006).
David, O., Kilner, J. M. & Friston, J. K. Mechanisms of evoked and induced responses in MEG/EEG. NeuroImage. 31(4), 1580–1591 (2006).
Jansen, D. A. & Cimprich, B. Attentional impairment in persons with multiple sclerosis. J. Neurosci. Nurs. 26(2), 95–102 (1994).
Gadea, M. et al. Spectroscopic axonal damage of the right locus coeruleus relates to selective attention impairment in early stage relapsing-remitting multiple sclerosis. Brain 127(Pt 1), 89–98 (2004).
Gonzalez-Rosa, J. J. et al. Cluster analysis of behavioural and event-related potentials during a contingent negative variation paradigm in remitting-relapsing and benign forms of multiple sclerosis. BMC Neurol. 11, 64 (2011).
Arrondo, G. et al. Abnormalities in brain synchronization are correlated with cognitive impairment in multiple sclerosis. Mult. Scler. 15(4), 509–516 (2009).
Vázquez-Marrufo, M. et al. Cognitive evoked potentials in remitting-relapsing and benign forms of multiple sclerosis. Rev. Neurol. 48(9), 453–458 (2009).
Keune, P. M. et al. Frontal brain activity and cognitive processing speed in multiple sclerosis: An exploration of EEG neurofeedback training. Neuroimage Clin. 22, 101716 (2019).
Binetruy, M. et al. Slowing of information processing speed without motor slowing in multiple sclerosis observed during two crossing-off tasks. Rev. Neurol. 172(3), 225–230 (2016).
Kluckow, S. W., Rehbein, J. G., Schwab, M., Witte, O. W. & Bublak, P. What you get from what you see: Parametric assessment of visual processing capacity in multiple sclerosis and its relation to cognitive fatigue. Cortex 83, 167–180 (2016).
Berard, J. A., Smith, A. M. & Walker, L. A. S. A Longitudinal evaluation of cognitive fatigue on a task of sustained attention in early relapsing-remitting multiple sclerosis. Int. J. MS Care. 20(2), 55–61 (2018).
Polich, J., Romine, J. S., Sipe, J. C., Aung, M. & Dalessio, D. J. P300 in multiple sclerosis: A preliminary report. Int. J. Psychophysiol. 12(2), 155–163 (1992).
Aminoff, J. C. & Goodin, D. S. Long-latency cerebral event-related potentials in multiple sclerosis. J. Clin. Neurophysiol. 18(4), 372–377 (2001).
Whelan, et al. A high-density ERP study reveals latency, amplitude, and topographical differences in multiple sclerosis patients versus controls. Clin. Neurophysiol. 121(9), 1420–1426 (2010).
Covey, T. J., Shucard, J. L. & Shucard, D. W. Event-related brain potential indices of cognitive function and brain resource reallocation during working memory in patients with multiple sclerosis. Clin. Neurophysiol. 128(4), 604–621 (2017).
Ciurleo, et al. Olfactory dysfunction as a prognostic marker for disability progression in multiple sclerosis: An olfactory event related potential study. PLoS ONE 13(4), e019600 (2018).
Jung, J., Morlet, D., Mercier, B., Confavreux, C. & Fisher, C. Mismatch negativity (MMN) in multiple sclerosis: An event-related potentials study in 46 patients. Clin. Neurophysiol. 117(1), 85–93 (2006).
Tewarie, P. et al. Disruption of structural and functional networks in long-standing Multiple Sclerosis. Hum. Brain Mapp. 35(12), 5946–5961 (2014).
Galvao-Carmona, A. et al. Disentangling the Attention network test: Behavioral, event related potentials and neural source analyses. Front. Hum. Neurosci. 8, 813 (2014).
Caravaglios, G., Muscoso, E. G., Di Maria, G. & Costanzo, E. Patients with mild cognitive impairment have an abnormal upper-alpha event-related desynchronization/synchronization (ERD/ERS) during a task of temporal attention. J. Neural. Transm. 122(3), 441–453 (2015).
Mangun, G. R. & Hillyard, S. A. Modulations of sensory-evoked brain potentials indicate changes in perceptual processing during visual-spatial priming. J. Exp. Psychol. Hum. Percept. Perform. 17(4), 1057–1074 (1991).
Mishra, J. & Hillyard, S. A. Endogenous attention selection during binocular rivalry at early stages of visual processing. Vis. Res. 49(10), 107–1080 (2009).
Mishra, J., Martínez, A., Schroeder, C. E. & Hillyard, S. A. Spatial attention boosts short-latency neural responses in human visual cortex. Neuroimage. 59(2), 1968–1978 (2012).
Gómez, C. M., Vázquez, M., Vaquero, E., López-Mendoza, D. & Cardoso, M. J. Frequency analysis of the EEG during spatial selective attention. Int. J. Neurosci. 95(1–2), 17–32 (1998).
Gómez, C. M., Flores, A., Digiacomo, M. R. & Vázquez-Marrufo, M. Sequential P3 effects in a Posner’s spatial cueing paradigm: Trial-by-trial learning of the predictive value of the cue. Acta Neurobiol. Exp. (Wars). 69(2), 155–167 (2009).
Martínez, A. et al. Objects are highlighted by spatial attention. J. Cogn. Neurosci. 18(2), 298–310 (2006).
Ortega, R., López, V., Carrasco, X., Anllo-Vento, L. & Aboitiz, F. Exogenous orienting of visual-spatial attention in ADHD children. Brain Res. 1493, 68–79 (2013).
Gómez, C. M., Vaquero, E., Vázquez, M., González-Rosa, J. J. & Cardoso, M. J. Alternate response preparation in a visuomotor serial task. J. Mot. Behav. 37(2), 127–134 (2005).
Pratt, N., Willoughby, A. & Swick, D. Effects of working memory load on visual selective attention: Behavioral and electrophysiological evidence. Front. Hum. . Neurosci. 135, 57 (2011).
Vacchi, L. et al. Working memory network dysfunction in relapse-onset multiple sclerosis phenotypes: A clinical-imaging evaluation. Mult. Scler. 23(4), 577–587 (2017).
Aguirre, N. et al. Repeated working memory training improves task performance and neural efficiency in Multiple Sclerosis patients and healthy controls. Mult. Scler. Int. 2657902, 1–13. https://doi.org/10.1155/2019/2657902(2019) (2019).
Polman, C. H. et al. Diagnostic criteria for multiple sclerosis: 2010 revisions to the McDonald criteria. Ann. Neurol. 69(2), 292–302 (2011).
Gronwall, D. M. A. Paced auditory serial-addition task: A measure of recovery from concussion. Percept Mot. Skills. 44(2), 367–373 (1977).
Tombaugh, T. N. A comprehensive review of the Paced Auditory Serial Addition Test (PASAT). Arch. Clin. Neuropsychol. 21(1), 53–76 (2006).
Smith, A. In The Symbol-Digit Modalities Test: A Neuropsychologic Test of Learning and Other Cerebral Disorders (ed. Helmuth, J.) 83–91 (Special Child Publications, 1968).
Sheridan, L. et al. Normative symbol digit modalities test performance in a community-based sample. Arch. Clin. Neuropsychol. 21(1), 23–28 (2006).
Beck, A. T., Steer, R. A., Ball, R. & Ranieri, W. Comparison of Beck Depression Inventories -IA and –II in psychiatric outpatients. J. Pers. Assess. 67(3), 588–597 (1996).
Beck, A. T., Steer, R. A. & Brown, G. K. Manual for the Beck Depression Inventory-II (Psychological Corporation, San Antonio, 1996).
McConnell, M. M. & Shore, D. I. Mixing measures: Testing an assumption of the Attention Network Test. Atten. Percept. Psychophys. 73(4), 1096–1107 (2011).
American Electroencephalographic Society. Guideline thirteen: Guidelines for standard electrode position nomenclature American Electroencephalographic Society. J. Clin. Neurophysiol. 11(1), 111–113 (1994).
Gratton, G., Coles, M. G. & Donchin, E. A new method for off-line removal of ocular artifact. Electroencephalogr. Clin. Neurophysiol. 55(4), 468–484 (1983).
Keil, A. & Müller, M. M. Feature selection in the human brain: Electrophysiological correlates of sensory enhancement and feature integration. Brain Res. 1313, 172–184 (2010).
Truccolo, W. A., Ding, M., Knuth, K. H., Nakamura, K. & Bressler, S. L. Trial-to-trial variability of cortical evoked responses: Implications for the analysis of functional connectivity. Clin. Neurophysiol. 113(2), 206–226 (2002).
Author information
Authors and Affiliations
Contributions
M. V., G.N. and G.I. designed the study. E.S. and A.G. collected the data. E.S., R.M. and A.G. ran the analyses. All authors participated in discussion to interpret the results, M.V., E.S. and G.I. wrote the paper, and all authors participated in revising it.
Corresponding author
Ethics declarations
Competing interests
The authors declare no competing interests.
Additional information
Publisher's note
Springer Nature remains neutral with regard to jurisdictional claims in published maps and institutional affiliations.
Supplementary information
Rights and permissions
Open Access This article is licensed under a Creative Commons Attribution 4.0 International License, which permits use, sharing, adaptation, distribution and reproduction in any medium or format, as long as you give appropriate credit to the original author(s) and the source, provide a link to the Creative Commons licence, and indicate if changes were made. The images or other third party material in this article are included in the article's Creative Commons licence, unless indicated otherwise in a credit line to the material. If material is not included in the article's Creative Commons licence and your intended use is not permitted by statutory regulation or exceeds the permitted use, you will need to obtain permission directly from the copyright holder. To view a copy of this licence, visit http://creativecommons.org/licenses/by/4.0/.
About this article
Cite this article
Vazquez-Marrufo, M., Sarrias-Arrabal, E., Martin-Clemente, R. et al. Altered phase and nonphase EEG activity expose impaired maintenance of a spatial-object attentional focus in multiple sclerosis patients. Sci Rep 10, 20721 (2020). https://doi.org/10.1038/s41598-020-77690-y
Received:
Accepted:
Published:
DOI: https://doi.org/10.1038/s41598-020-77690-y
- Springer Nature Limited