Abstract
Soil Organic Carbon (SOC) plays a vital role in the global carbon cycle, affecting soil fertility and agricultural sustainability. Our study focused on areas with low SOC, where increasing its levels could enhance soil health and carbon management. We used an earth science approach to analyze SOC density and stock in different land classes of the Urmodi River watershed in Maharashtra, India. Using GIS, we estimated SOC from soil samples collected up to 20 cm depth, and produced maps of SOC, SOC density (SOCD), and SOC stock. Our results showed that natural vegetation areas, such as closed and open forests, had a higher nutritional status of SOC and SOC stock, indicating the importance of land cover history on soil health. We found the average SOC to be 1.4 g/kg−1, SOCD to be 57.36 kg/m−2, SOC stock to be 3.46 ton/ha−1. We also depicted the relationship between elevation and SOCD using a scatterplot, revealing the distribution of SOC across different elevations. Our study demonstrates the use of modern geoscientific analysis tools to understand the physical and chemical properties of soil, which can be useful in assessing soil health. Overall, our results provide valuable insights into the distribution of SOC and its relationship with other geo-chemical aspects at a regional scale.
Similar content being viewed by others
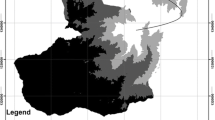
Avoid common mistakes on your manuscript.
1 Introduction
Soil plays a crucial role in the global carbon cycle, serving as a significant source of carbon to the atmosphere and storing more carbon than biomass [1]. In fact, the amount of organic carbon in soil is estimated to be twice the amount of carbon in the atmosphere [2]. Soil organic carbon (SOC) is a critical component of terrestrial ecosystems, indicating soil health, and its loss can result in land degradation, negatively impacting cultivable land cover [3]. One of the on-site impacts of accelerated soil erosion is the loss of SOC, which can have a significant impact on the carbon pool of the ecosystem [4]. SOC is the primary component of any terrestrial ecosystem, and any changes to its quantity and composition can significantly affect associated systems. Soil fertility, which is critical for cultivable land, is heavily influenced by SOC [5]. This chemical property is a vital characteristic of soil health and regeneration, and it plays a critical role in global carbon circulation, influencing soil productivity and ecosystem functions [6]. The distribution of SOC is heterogeneous, with variations depending on land cover, soil type, and climate [7]. Typically, the study of SOC in soil involves analyzing soil profiles to a depth of 0–20 or 0–30 cm. Plant residues are the primary source of SOC [8]. In tropical regions such as India, restoring SOC is a significant concern for better resource management, but inorganic agricultural practices can pose barriers to soil physical properties and biological activities [9]. It is essential to reduce deforestation, increase soil organic matter (SOM) in agricultural areas, and minimize erosion in tropical countries like India since these activities are associated with SOC [10]. The content of SOM, which acts as a binding agent for soil aggregates, plays a crucial role in achieving a stable SOC balance, and the use of compost and no-tillage practices can help achieve this goal [2, 11]. The soil organic carbon (SOC) pool is an important component of the carbon cycle and has significant implications for ecosystem services and human wellbeing. To quantify the SOC pool, various parameters such as SOC, soil organic matter (SOM), and bulk density (BD) are used, with SOM and BD being strongly related [12]. Soil organic carbon is influenced by land use, climate (including temperature and precipitation), and soil hydrology. Its significance stems from the fact that even minor changes in the soil carbon pool can have a significant impact on both agricultural output and the worldwide greenhouse gas balance [13]. Although BD has a weak relationship with SOC, it is still necessary to consider both parameters for calculating the volume-based carbon stock and potential changes over time. Modeling approaches can be used to accurately estimate the carbon stock in this case. Soil degradation is a critical issue affecting agricultural areas, leading to a decrease in soil carbon, which poses a threat to ecosystem services and human health [14]. Soil erosion caused by water is a major contributor to soil degradation in arid and semi-arid regions. SOC is a vital factor in soil processes, and its abundance is primarily determined by the decomposition of plant and animal residues by microbes, which is influenced by ambient soil conditions, moisture, and temperature [15]. Human activities such as land use changes, including the conversion of forests and grasslands to croplands, have resulted in significant alterations to land resources, necessitating an assessment of their impact on soil properties [16]. In the 1990s, quantitative methods for soil survey and mapping emerged, based on the analysis of the relationship between soil particles and soil formation factors, leading to the development of digital soil mapping techniques [17]. The main challenge in SOC mapping is the inherent spatial variability of soil carbon. Since the spatial variance of SOC is scale-dependent, it is essential to understand the contribution of various factors such as land cover, topography, and climate to its distribution [18]. A study carried out by Nandgude et al. [19], has revealed that, 6557.44 ha of Urmodi river watershed has shown low SOC (< 5000 ton/ha−1) at the central region. Approximately, in the same period i.e. 2015 lower vegetation density (0.1–0.3) map created by using Normalized Difference Vegetation Index (NDVI) has shown 92.36 km2 that could lead to land degradation activities led by rainfall erosivity [20]. Geographical Information System is a good platform to map vulnerability map and useful in crating the resource mapping [21]. Mapping terrestrial carbon stocks at the elevation layer level in larger geographical areas is useful for understanding soil properties. Digital Soil Mapping (DSM) integrates field, laboratory, and proximal soil observations with quantitative methods to infer spatial patterns of soils across different spatio-temporal scales [22]. To produce a SOCD map, the distribution of SOC, BD, and coarse soil particles was processed in a Geographic Information System (GIS) platform. The main objective of the study was to show the spatial distribution SOC, SOCD and SOC stock across the varius zones of elevation, vegetation density, Köppen-Geiger climate zone, soil type and land use land covers in the Urmodi River watershed. Such studies are crucial for understanding soil carbon dynamics and devising effective management strategies to maintain and enhance soil health. The findings of this study have significant implications for land management practices and the development of effective carbon sequestration strategies. The identification of elevation zones and their corresponding SOC, SOCD, and SOC stock provides crucial information for understanding carbon storage potential in different areas.
2 Study area
The Urmodi River watershed is located in Satara and Jaoli blocks of Satara district, Maharashtra State in India covers an area of 412.44 km2 located within longitude 73°47′37.235″E to 74°6′59.555″E and latitude 17°28′32.329″N to 17°44′34.352″N (Fig. 1). Urmodi River originated from Kaas pond which is about 36 km and meet with Krishna River near Kashil village. The river also has a waterfall named ‘Vajrai’ with a height of about 250 m, two adjacent watersheds: Venna River and Tarali River and embarked with an earthen dam named by the river itself (Urmodi). It is a major irrigation project constructed by the Government of Maharashtra to irrigate the drought-prone talukas (administrative boundary), viz. Khatav and Maan. It is a tropical watershed it mainly receives rainfall during June–September. The average annual rainfall range in the two blocks is 981.4 mm and 1661.7 mm, respectively, from 2002–2016 [23]. According to the climate classification done by [24], the watershed is divided into two zones: 2-Am: Tropical, monsoon covering 23.83 km2, and Aw: Tropical, savannah 388.60 km2. The whole Satara district lies in the Deccan peninsula of South India. The ‘Kaas Plateau’ is a world heritage site located in the same study area. It is a sub-cluster of Sahyadri (ID: 1342rev-036) that lies in this watershed. The plateau area covers 1142 hector [25]. The upper catchment of the study region predominantly has a plateau region, and one of the unique biodiversity-rich areas is present in this part of the region, which has conservation, aesthetic value, and tourist importance [26]. The entire watershed falls into the ninth agro-climatic zone: India's western plateau and hilly region [27]. Paddy cultivation occurs at the upper catchment, and Rabi and Kharif crops are cultivated in the lower watershed. Geology is one of the main components of terrain. In the study area we can find four types of rock namely-15aa pahoehoe mixed flows, 9aa simple flows (350–400 m), Megacryst flows M4, 8aa simple flows, Laterite [28]. From the lower catchment, there passes the National Highway 4 (NH-4), with a length of 20 km. Based on a visual interpretation of the World Reference Base (2006) raster data obtained from the website (https://soilgrids.org/), the study area was found to have soil groups such as leptosols, cambisols, regosols, luvisols, and vertisols. Among these soil groups, the undulating geographic areas were observed to be covered by leptosols, while vertisols were found to be present in the downslope areas, ranging from the middle to the mouth of the Urmodi river. There is also classication of soil type map prepared by National Bureau of Soil Survey and Land Use Planning [29], the details of characteristic is provided in supplementary material file.
3 Material and methods
From a scientific and sustainability perspective of land management, it is crucial to assess soil organic carbon (SOC) at the watershed level, considering the existing bio-physical spatial entities. This assessment is essential for effective land resource management and maximizing carbon sequestration potential. So, there were conducted such studies to assess the SOC dynamic in their study regions. The SOC is driver has been used to demonstrate the elevation, vegetation types to show distribution of SOC stock using spatial modeling and mapping framework; such analysis is helpful for carbon accounting [30]. The soil nutrient and the other bio-physical factor has relationship with the ecosystem where is gets form. The SOC stock has crucial role in assessing the variation in carbon pool in various land cover [31].
3.1 Remote sensing and thematic data
Remote Sensing (RS) based Cartosat Digital Elevation Model (DEM) of 30 m spatial resoulution data to understand the study region′s topography. We also processed the data already georectified with datum WGS 84 it for assignment in the UTM zone 43 N. The DEM further reclassified using natural jenk method in five classes and showed in Fig. 1. The data provided by Copernicus Global Land Service with 100 m spatial resolution has been used to investigate the type of land covers. This layer comes with 23 classes. The accuracy of the thematic raster grid is 80.2% ± 0.7% [32]. The data represents the land cover condition of the year 2015. Since soil sampling was carried out in the year 2015–16, we have used this dataset. Tile no. of the data is E060N20 accessed on 19 April 2020 (source link: https://lcviewer.vito.be, https://doi.org/10.5281/zenodo.3939038). The file format of the layer is GeoTIFF. In order to examine the correlation between soil organic carbon (SOC) and soil organic carbon density (SOCD) with vegetation, imagery from Landsat 8's remote sensing (RS) technology was obtained from Earth Explorer with a path of 147 and a row of 48, captured on November 11, 2015. Additionally, thematic data from Beck et al. [24] was used to classify the climate of the study area under the Köppen-Geiger climate classication system. This provided a description of the present climatic conditions in the area.
3.2 Field soil sampling and preparation
Unbiased soil sampling with a random approach was adopted, ensuring the inclusion of all land cover classes and maintaining the representativeness of the collected soil samples. For this, the predetermined stratification of different soil types are taken into consideration based on maps of National Bureau of Soil Survey and Land Use Planning (NBSS and LUP); and random samples are collected over these types. Sixty one (61) soil samples were collected within and around the watershed. At the time of sampling (November 2015–March 2016) the centroid point of the study area has shown relative humidy about 51.5% (Location 17.6310 N, 73.9494 E) (Source: https://power.larc.nasa.gov/data-access-viewer/). Stratified random sampling method was adopted for the collection of samples. This method has advantages over simple random sampling and gives better results [33]. The same approach was used by Sreenivas et al. [6] for different soil types and various land cover. The predefined strata of various soil types are taken into consideration based on maps of NBSS and LUP and soil samples were collected after the monsoon at the depth of 0–20 cm. Soil samples were passed through a sieve of 2 mm for determination of some parameters for SOCD.
3.3 Estimation of bulk density
Bulk density (BD) is a physical parameter of soil. It reflects the total porosity of the soil. It also describes soil quality and ecosystem functions. High BD indicates the poorer conditions for plant root growth, low aeration, and adverse changes in hydrologic functions such as compact water infiltration [34]. For mapping the purpose of this parameter, we collected the secondary data of physical soil characteristics of bulk density from the District soil survey and soil testing lab (Government of Maharashtra) at Hamdabaj in Satara. It includes soil samples from 27 locations, which includes sampling locations inside and surrounding to Urmodi River watershed.
3.4 Walkley and black method for estimation of SOC
Laboratory analysis by wet digestion method for all samples for estimation of soil organic carbon content was carried out using [35] method. It is the most widely and commonly used method for SOC. The method can be used to finding the sequester capacity of soil [13].
3.5 IDW Interpolation and scatterplot of raster datasets
The inverse distance weighted (IDW) is one of the commonly used interpolation methods which are used in soil science [36].
where Z(X0) is the resultant interpolated value, n is the number of samples used as a spatial entity, Xi is the ith data value, hij is the separation distance between interpolated values and sample data value, β shows the weighting power. In this case, it is by default value is 2.
Sometimes, there is needed to show the relationship between two raster grids to understand the pattern of accumulation of data. In this case, scatterplot contributes a great help. It is simply a statistical graph that use the point to depict a corresponding value in the Cartesian plane. With the help of two graphic data, a point cloud, and local/global fitted lines, a scatterplot reflects different aspects between the variables [37]. To obtain the same we have used System for Automated Geoscientific Analyses SAGA version 7.2.0 by importing GeoTIFF file into the software and by performing scatterplot of two raster datasets [38].
3.6 Calculation of soil organic carbon density and SOC stock
Soil Organic Carbon Density (SOCD) refers to SOC reserve or storage per unit area in a soil profile with specific depth. While calculating the SOCD, bulk density map shown in Fig. 2 used as input to estimate the SOC density. It is one of the essential indexes for the evaluation and measurement of SOC reserve in an area [39]. The formula mentioned by Batjes [40] has been employed for the estimation of SOCD (kilogram/m−2). The equation has been previously used by Sreenivas et al. [6].
where, SOCD is soil organic carbon density expressed in kg/m−2 for each sampling profile, SOC is estimated soil organic content of soil particle (< 2 mm) in (g/kg−1), BD is bulk density expressed in (g/cm−3), Ti is soil sampling depth which was constant for all the soil sample i.e. 20 cm, CS shows volumetric percent of 2 mm soil fragments (coarse soil). Moreover, we have estimated SOC stock (ton/ha−1) in the soil using the equation mentioned by Guo and Gifford, Yigini and Panagos [41, 42].
3.7 Computation of normalized difference vegetation index
This is one of the widely used indices based on band rationing (unsupervised classication method) to reflect the vegetation condition status captured by satellite imageries. The values of this indices always lie between −1 to 1. Where, higher values represents healthy vegetation and low values depit absence or lower vegetation condition. In the present study vegetation density classes were prepared based on NDVI values. A classification of NDVI was mentioned by Zheng and Yu [43] vegetation density classes such as No vegetation cover (< 0.1), Low vegetation cover (0.1–0.3),Moderate vegetation cover (0.3–0.4), High vegetation cover (> 0.4) are prepared and shows the status of vegetation canopy.
where, NIR is Landsat 8 band-5, Red is Landsat 8 band-4.
4 Results and discussion
4.1 Bulk density and coarse soil fragments
Bulk density and coarse soil fragments maps of the study region was portrayed in Figs. 2 and 3 respectively. It was evident from the map that the upper catchment area shows lower soil density because it is covered with forests.
This phenomenon can be attributed to the prevalence of laterite rock type and soil in the study area, with a lesser occurrence of basaltic rock and black sandy loam soil in the lower catchment. Hydrologically, the higher bulk density (BD) of soil in the lower catchment as compared to the upper catchment can also be attributed to surface runoff. It has been also documented by [16] that, cultivable land and grassland have a quite high bulk density as compared to forest soils. And, the lower catchment shows high bulk density. It was found that the average bulk density of soil 1.24 g/cm−3. Interestingly, the red soil (formed from laterite rock) shows lower values of bulk density. The soils formed from the pahoehoe mixed-flow shows higher values of bulk density. The southwest part of the watershed shows the highest value which also depicts that, this region was quite unsuitable for plant root growth. It was also evident from the elevation data that, due to gravitational rolling of soil particles and surface runoff the low elevated areas were observed high bulk density. It has been observed from the collected soil samples that, the majority of the soil lies in the sandy loam, loamy sand, and loamy type of soil texture. At the center of the watershed (at a lower elevation area) a large amount of coarse soil was observed. Because during soil erosion coarse fraction of soil deposited at the lower end of the slope [44]. The higher upland areas show less coarse soils. These areas are covered with open or closed types of forest. The high elevated area with shrubs (basically barren) also has coarse soil texture. Hence, vegetation cover and elevation also has a role in the definition of soil physical properties. From LULC spatial distribution point, shrubs and herbaceous vegetation exhibit moderately compacted soils with bulk density values of 1.25 g/cm−3 and 1.19 g/cm−3, respectively. Cultivated and managed vegetation/agriculture, as well as urban/built-up areas, have slightly higher bulk density values of 1.27 g/cm−3. Permanent water bodies have lower values at 1.22 g/cm−3 due to sediments and organic matter. Closed forests, including evergreen needle leaf, evergreen broadleaf, and deciduous broadleaf, generally display lower soil bulk densities ranging from 1.12–1.21 g/cm−3, while open forests show similar values with slightly lower density at 1.09–1.21 g/cm−3. These interpretations indicate variations in soil compaction and structure across different land cover types, influenced by factors such as vegetation, land management practices, and urbanization.
4.2 Soil organic carbon and SOC density of the Urmodi River watershed across various LULC
According to Fig. 4, the SOC content in the soil at 0–20 cm depth. It has been revealed from the analysis that, high values of SOC in soil were present at the higher altitude of the Urmodi River catchment. The area was predominantly covered with forest canopies. The similar SOC condition in the forests of south-west India having dry-deciduous forest cover [45]. At the high elevation area, it shows a value of more than 1.5 g/kg−1. One of the evidence for high SOC was recorded by Bhunia, et al. (2018) they also found the SOC content was high in the soils of the forest. Besides, the lower part of the catchment i.e. the left bank of the Urmodi River and closer to the joining of Krishna river it showed the highest SOC content in the soil. The mean SOC in the soil was found to be 1.4 g/kg−1. Table 1 shows the descriptive statistics of the SOC. After the integration of the SOC, BD, and coarse soil fragments maps as mentioned in Eq. (2), SOCD maps were produced. It is displayed in Fig. 5. The average SOCD of the watershed was 57.36 kg/m−2. By observing Fig. 4, high coarse soil areas have shown more SOCD values. The areas like open and closed forest zones show low values of SOCD as the soils of such LULC classes have lower bulk density. The pattern of SOCD was found to be different at the topsoil of different land covers too. By using the above layers and with the help of Eq. (3), SOC stock of the Urmodi river watershed is framed in Fig. 6. From the visual GIS map-based interpretation it was revealed that,the agriculture zone of the catchment showed high values of SOCD. The lowest values are observed at barren land or shrub land as less vegetation cover. These results match with the study done by Sreenivas et al. [6], they have found the low values of SOCD in such areas. The SOCD in the whole watershed from low to high values was loamy, loamy sand, and sandy loam soils. There has found patterns of distribution across various land cover type. The LULC map was displayed in Fig. 7 and detail are provided in Table 2 which describes the area of each land cover. The zonal statistics as table tool was used to analyze the relationship between SOC and SOCD across various land use/land cover categories, as shown in Table 3. The results reveal that the highest SOC values were observed in agriculture land situated in the upper catchment and covered by closed forest, with an average value of 3.67 g/kg−1. Closed forests (both deciduous and evergreen) were found to be the best reservoirs of SOC, with an average value of 3.67 g/kg−1, followed by open forests (both deciduous and evergreen) with an average value of 3.47 g/kg−1. Herbaceous and shrub lands showed an average SOC value of 3.43 g/kg−1, while cultivable/agriculture lands had an average value of 3.46 g/kg−1. SOCD values were also calculated for each LULC category, with closed forests having the highest SOCD value of 57.10 kg/m−2, followed by herbaceous vegetation and shrub lands with a value of 57.22 kg/m−2, open forests with a value of 53.28 kg/m−2, and agriculture lands with a value of 59.22 kg/m−2. Permanent water bodies and settlements/built-up areas were found to have no future significance, as there are less chances of their alteration. Moreover, the shrub land and herbaceous vegetation cover land surface has also showed low SOC content. In the higher elevated area due to steeper slope, there occurs loss of SOC which causes depletion in SOC [46].
4.3 Regional pattern of SOC stock
From the analysis of the soil samples and through the analysis the SOC stock map as shown in Fig. 6 the closed and open forest soils showed a high SOC stock. Increment in SOC has positive effects on agriculture crop yield and key component in promotion of ecosystem services associated with air and water quality [47]. According to the classification done by the Copernicus Global Land Service (CGLS), Fig. 7 portyras the Land Use Land Cover (LULC) observed in the Urmodi river watershed. There were 14 land covers (Table 2) in the Urmodi River watershed. There were four types of closed forest and four types of open forests along with agricultural land (cropland), permanent water body, shrub land, herbaceous shrubs, and a very small patch of wetland and urban settlement (built-up). Land use sustainability can be assessed by measuring the changes in SOC; decline in soil carbon stock can lead to poor plant performance, soil erosion, and the ever-increasing need for other inputs to stabilize the requirement of nutrients to stabilize the soil health, poor soil condition for the plant to root and soil biodiversity [48]. As stated in Table 3, the statistics reveal interesting insights into carbon storage across different ecosystems. Closed forests demonstrate relatively higher carbon stocks, with a mean value of 3.69 ton/ha−1, while cultivated and managed vegetation/agriculture exhibit a wider range and lower mean of 3.46 ton/ha−1. Herbaceous vegetation and wetlands display similar mean carbon stocks of 3.46 ton/ha−1. Open forests and shrublands also exhibit moderate carbon stocks with mean values of 3.42 ton/ha−1 and 3.39 ton/ha−1, respectively. Permanent water bodies and urban/built-up areas have the lowest mean carbon stocks at 3.14 ton/ha−1 and 3.51 ton/ha−1, respectively.
The regional pattern has various dynamic and a study conducted by Rajapaksh et al. [30] revealed that vegetation type and DEM were the main factors influencing SOC stocks across two depth intervals. These findings emphasize the significant role of vegetation cover and topographic characteristics in shaping SOC levels. Generally in these areas, we have found that they lie in the range of 3.64–5.29 ton/ha−1 of SOC. Land use alteration affects the soil carbon accumulation and interlinked with vegetation cover alteration. [49]. In addition to this, we have also found the high values of SOC in the lower catchment where this area is primarily used for agricultural activities. The lowest value is found at the hilltops with less vegetation cover such as shrub land or land with herbaceous vegetation. The average value of the SOC stock in the watershed was 3.45 ton/ha−1. From the field observation, it was also clear that the left side of the watershed was extensively covered with eucalyptus plantation and lower values of SOC were observed over there. In general, differences in soil quality, the amount of leaf litter that falls, and the rate of decomposition are reflected in the soil's organic carbon content [50]. Eucalyptus spp. litter takes a very long time to break down, and flood pulses sweep it away before nutrients are released [51]. These results suggest that natural ecosystems such as closed forests and wetlands have higher carbon sequestration potential compared to human-modified landscapes like cultivated areas and urban environments. The findings emphasize the importance of preserving and restoring natural ecosystems to mitigate carbon emissions and enhance carbon storage for climate change mitigation and ecosystem resilience.
4.4 Spatial zonation of SOC, SOCD and SOC stock with elevation, climate zone, soil types and vegetation density
4.4.1 Climate zone condition and SOC derived parameters
There are two climate zones observed in the Urmodi river watershed. Table 4 describes Köppen-Geiger climateclassification of Urmodi River watershed with respective area contributing to SOC, SOCD and SOC stock with statistics. Figure 8 shows that, the Köppen-Geiger climate classification system the Urmodi River watershed is divided into two climate zones, namely the Am: Tropical monsoon spreading across high altitude area with geographicl area 23.83 km2 (5.78%)zone and Aw: Tropical savannah zone covers an area of 388.60 km2 (94.22%). Temperature and precipitation are the two main climatic factors that influence SOC storage globally as well as at large (sub)regional scales, affecting both the amount of carbon that enters the soil and the rate at which SOC decomposes [52]. Zonal statistical analysis performed using GIS revealed that the average SOC value in the tropical monsoon zone was 1.67 g/kg−1, which was higher than the tropical savannah zone with an average SOC value of 1.39 g/kg−1. Similarly, in the case of SOCD, the average value in the tropical monsoon zone was 58.23 kg/m−2, slightly higher than the tropical savannah zone, which showed an average SOCD value of 57.15 kg/m−2. In the tropical monsoon climate zone, the SOC stock ranges from 3.08–4.34 ton/ha−1, with an average of 3.76 ton/ha−1. This zone exhibits relatively consistent carbon stocks, indicated by the low standard deviation of 0.26. On the other hand, the tropical savannah climate zone shows a wider range of carbon stocks, varying from 1.40 to 5.09 ton/ha−1, with a mean of 3.43 ton/ha−1. The larger standard deviation of 0.60 in this zone suggests greater variability in carbon stocks. These results provide valuable insights into the carbon storage potential of soils in these specific climate zones. Understanding the variations in soil organic carbon stocks is crucial for effective carbon management and devising strategies to enhance carbon sequestration. Soil type and clay content impact organic carbon characteristics.
4.4.2 Vegetation density condition and SOC parameters
According to Table 5, and Landsat 8 based NDVI image as displayed in Fig. 9 the zonal statistics of SOC, SOCD and SOC stock was computed. The analysis showing relationship of the combination of the above computed factors with NDVI, and the average values of SOC was observed in high vegetation density area with score 1.52 g/kg−1, and high SOCD values with mean 54.96 kg/m−2 in low vegetation cover with value 57.96 kg/m−2 as this value is affected due to bulk density, and SOC stock in the study region was found highest in high vegetatop density with value 3.54 ton/ha−1 and lower in no vegetation area with value 3.25 ton/ha−1. The statistics reveal important insights into the SOC variations across different vegetation density categories. The results demonstrate that as vegetation density increases, there is a general trend of increasing SOC content. This indicates a positive correlation between vegetation density and carbon storage in the soil. The mean SOC values for each vegetation density class support this finding, showing higher values for classes with greater vegetation cover. Additionally, the standard deviation values suggest varying degrees of variability in SOC within each vegetation density class. This indicates that factors beyond vegetation density, such as soil characteristics and management practices, may influence the exact SOC content within a particular class. These findings contribute to our understanding of the complex relationship between vegetation density and SOC and emphasize the role of vegetation in carbon sequestration. Low SOC was found in the middle part of the study area, as well as areas with less vegetation cover or areas with no vegetation cover. The present study has also found that the previous vegetation cover history has a significant contribution in enhancing the soil SOC content in the soil.
4.4.3 Elevation zonation and SOC based parameters
The DEM is categorized into five classes (Fig. 1) as very low (503–607 m), low elevated (608–703 m), moderate elevated (704–841 m), high elevated (842–992 m), and very high elevated zone (993–1185 m). Table 6 shows the altitude zonation with its area and respective proportion. The minimum SOC content ranges from 0.51–0.86 g/kg−1, while the maximum SOC content ranges from 1.98–2.00 g/kg−1. The mean SOC content ranges from 1.35–1.54 g/kg−1. The standard deviation (SD) indicates the variability in SOC content within each elevation zone and ranges from 0.19–0.26 g/kg−1. The SOC values for each elevation zone provide insights into the distribution and variation of SOC content across different altitude. The range between the minimum and maximum values within each zone indicates the potential differences in SOC content based on elevation. The mean and standard deviation values provide information on the average and variability of SOC content within each elevation zone. In the same way, elevation zone wise SOCD is mapped, the minimum SOCD ranges from 12.10–0.05 kg/m−2, while the maximum SOCD ranges from 106.79–144.88 kg/m−2. The mean SOCD ranges from 52.86–58.70 kg/m−2. The standard deviation (SD) indicates the variability in SOCD within each elevation zone and ranges from 13.15–19.95 kg/m−2. Also, the minimum SOCS ranges from 1.29–2.08 ton/ha−1, while the maximum SOCS ranges from 5.29–4.48 ton/ha−1. The mean SOCS ranges from 3.40–3.59 ton/ha−1. The SOCS values for each elevation zone provide insights into the distribution and variation of soil organic carbon stock across different elevations. The standard deviation (SD) indicates the variability in SOCS within each elevation zone and ranges from 0.38–0.68 ton/ha−1. Moreover, a GIS basel pixel correlation between SOCD and elevation is also plotted in SAGA GIS platform and shown in Fig. 10. Interestingly, we have found that the range of SOCD varies with different elevation range of 520–720 m high values of SOCD in the range of 30–90 kg/m−2 were present. The advantage of this plot has crucial role in portraying the spatial distribution of any spatial raster layer.
5 Conclusions
The study showcases the effectiveness of GIS technique when integrated with Digital Soil Mapping to map SOC in the Urmodi River watershed, a tropical area where soil nutrients such as SOC play a crucial role in the terrestrial ecosystem. The physical properties of soil and nutrient values can change with shifting land-use patterns, which is why we examined how changes in elevation and land-use history affect SOCD and SOC stock values. The research plays a pivotal role in soil carbon management, contributing to the goal of sustainable agriculture. RS-based datasets, such as land cover layer and DEM, are crucial in understanding the impact of regional entities on soil's physical and chemical properties. In addition, scatterplots can identify SOC sink or deposition. By using RS-based data, such as elevation and land cover layers derived from satellite data, we can analyze soil properties at different spatial locations, helping us identify areas where SOC depletion is occurring so that we can take appropriate measures to prevent soil degradation. This study used a DSM technique to explore the spatial distribution of SOC in the hilly terrain of the Urmodi River watershed in Maharashtra State, India. Furthermore, this study emphasizes the need for future research and monitoring efforts. It is recommended that further investigations explore the specific factors influencing SOC dynamics in different elevation zones and assess the effectiveness of different land management approaches in enhancing carbon sequestration.
6 Recommendation and outlook
Multiple factors are involved in the formation of organic matter in the soil through soil degradation activities. The biophysical factors and their alterations are closely linked to both natural and anthropogenic activities. From a sustainable soil nutrient management viewpoint, it is crucial to closely examine the human impact within the Urmodi river catchment. Notably, as the watershed encompasses diverse landforms and exhibits high slope gradients, it has a detrimental impact on soil health. Water-induced soil loss leads to the washout of soil nutrients and negatively affects soil fertility. Agricultural areas situated in elevated regions show clear signs of gully formation and limited soil depth for plant roots, rendering them unsuitable for future farming.Low-lying areas with lower soil organic carbon (SOC) stocks can be prioritized for the application of naturally available cow dung as part of Nature-based Solutions (NbS) to enhance carbon stock. Planting indigenous species that decompose their plant litter relatively quickly should be promoted in upper catchment areas, plateaus, and reserved forests, while avoiding the use of Eucalyptus spp. A substantial amount of SOC in the soil not only regulates microbial activities but also serves as a binding agent among soil particles, protecting the surface soil from splash erosion.Additionally, extensive plantation efforts should target barren lands and areas with low vegetation, collaborating with non-governmental organizations to raise awareness about sustainable land management practices. From the future research vision, the leaching of SOC could be an influencial area. This can be assist the soil scientist to find out the rate of leaching. There is need to find out the electric resistivity tomography profile and induced polarization IP profile as done by Brahmi et al. [53] for picturizing in in-depth analysis of SOC, SOCD and SOC stock to conserve the nutrient balance and sustainable utilization of carbon pool in the Urmodi river watershed. Moreover, continuous monitoring of SOC levels and land use changes is crucial for evaluating the long-term impact of conservation and restoration measures and refining land management strategies to optimize carbon storage and ecosystem health.
Availability of data and material
Not Applicable.
Abbreviations
- BD:
-
Bulk density
- CGLS:
-
Copernicus global land service
- CS:
-
Coarse soil
- DEM:
-
Digital elevation model
- DSM:
-
Digital soil mapping
- ENVIS:
-
Environmental information system
- FAO:
-
Food Agriculture Organization
- GIS:
-
Geographical information system
- IDW:
-
Inverse distance weighted
- LULC:
-
Land use land cover
- NBSS and LUP:
-
National Bureau of Soil Survey and Land Use Planning
- SAGA:
-
System for automated geoscientific analyses
- SOC:
-
Soil organic carbon
- SOCD:
-
Soil organic carbon density
- SOM:
-
Soil organic matter
- UNESCO:
-
United Nations Educational Scientific and Cultural Organization
- UTM:
-
Universal transverse mercator
- WGS:
-
World geodetic system
References
Lugo AE, Brown S (1993) Management of tropical soils as sinks or sources of atmospheric carbon. Plant Soil 149:27–41. https://doi.org/10.1007/BF00010760
Berihu T, Girmay G, Sebhatleab M, Berhane E, Zenebe A, Sigua GC (2017) Soil carbon and nitrogen losses following deforestation in Ethiopia. Agron Sustain Dev 37:1. https://doi.org/10.1007/s13593-016-0408-4
Winowiecki L, Vågen T-G, Massawe B, Jelinski NA, Lyamchai C, Sayula G, Msoka E (2016) Landscape-scale variability of soil health indicators: effects of cultivation on soil organic carbon in the Usambara Mountains of Tanzania. Nutr Cycl Agroecosyst 105:263–274. https://doi.org/10.1007/s10705-015-9750-1
Lal R (2014) Soil conservation and ecosystem services. Int Soil Water Conserv Res 2:36–47. https://doi.org/10.1016/S2095-6339(15)30021-6
Singh A, Santra P, Kumar M, Panwar N, Meghwal PR (2016) Spatial assessment of soil organic carbon and physicochemical properties in a horticultural orchard at arid zone of India using geostatistical approaches. Environ Monit Assess 188:529. https://doi.org/10.1007/s10661-016-5522-x
Sreenivas K, Sujatha G, Sudhir K, Kiran DV, Fyzee MA, Ravisankar T, Dadhwal VK (2014) Spatial assessment of soil organic carbon density through random forests based imputation. J Indian Soc Remote Sens 42:577–587. https://doi.org/10.1007/s12524-013-0332-x
FAO (2017) Soil organic carbon the hidden potential. https://www.fao.org/3/i6937e/i6937e.pdf
Lei Z, Yu D, Zhou F, Zhang Y, Yu D, Zhou Y, Han Y (2019) Changes in soil organic carbon and its influencing factors in the growth of Pinus sylvestris var. mongolica plantation in Horqin Sandy Land, Northeast China. Sci Rep 9:16453. https://doi.org/10.1038/s41598-019-52945-5
Bhunia GS, Shit PK, Maiti R (2016) Spatial variability of soil organic carbon under different land use using radial basis function (RBF). Model Earth Syst Environ 2:17. https://doi.org/10.1007/s40808-015-0070-x
Feller C, Albrecht A, Blanchart E et al (2001) Soil organic carbon sequestration in tropical areas. General considerations and analysis of some edaphic determinants for Lesser Antilles soils. Nutr Cycl Agroecosystems 61:19–31. https://doi.org/10.1023/A:1013359319380
Adhikari G, Bhattacharyya KG (2015) Correlation of soil organic carbon and nutrients (NPK) to soil mineralogy, texture, aggregation, and land use pattern. Environ Monit Assess 187:735. https://doi.org/10.1007/s10661-015-4932-5
Périé C, Ouimet R (2008) Organic carbon, organic matter and bulk density relationships in boreal forest soils. Can J Soil Sci 88:315–325. https://doi.org/10.4141/CJSS06008
Bahri H, Annabi M, Saoueb A, M’Hamed HC, Souissi A, Chibani R, Bahri BA (2018) Can conservation agriculture sequester soil carbon in Northern Tunisia in the long run? In: Kallel A, Ksibi M, Ben Dhia H, Khélifi N (eds) Recent advances in environmental science from the Euro-Mediterranean and surrounding regions. Springer, Cham, pp 85–87
Céspedes-Payret C, Bazzoni B, Gutiérrez O, Panario D (2017) Soil organic carbon vs. bulk density following temperate grassland afforestation. Environ Process 4:75–92. https://doi.org/10.1007/s40710-016-0197-4
Imamoglu A, Dengiz O (2017) Determination of soil erosion risk using RUSLE model and soil organic carbon loss in Alaca catchment (Central Black Sea region, Turkey). Rendiconti Lincei 28:11–23. https://doi.org/10.1007/s12210-016-0556-0
Göl C (2017) Effects of aspect and changes in land use on organic carbon and soil properties in uludere catchment, semi-arid region: Turkey. Rendiconti Lincei 28:463–469. https://doi.org/10.1007/s12210-017-0619-x
Zhang G, Liu F, Song X (2017) Recent progress and future prospect of digital soil mapping: a review. J Integr Agric 16:2871–2885. https://doi.org/10.1016/S2095-3119(17)61762-3
Adhikari K, Owens PR, Libohova Z, Miller DM, Wills SA, Nemecek J (2019) Assessing soil organic carbon stock of Wisconsin, USA and its fate under future land use and climate change. Sci Total Environ 667:833–845. https://doi.org/10.1016/j.scitotenv.2019.02.420
Nandgude S, Shinde S, Mahale D, Nagarkar S (2015) Assessment of soil organic carbon storage in urmodi basin of Krishna River using geographic information system. Agropedology 25:147–153
Bagwan WA, Gavali RS (2019) Image processing based vegetation cover monitoring and its categorization using differential satellite imageries for Urmodi River watershed in Satara District, Maharashtra, India. In: Santosh KC, Hegadi RS (eds) Recent trends in image processing and pattern recognition. Springer, Singapore, pp 329–341
Nekkoub A, Baali F, Hadji R, Hamed Y (2020) The EPIK multi-attribute method for intrinsic vulnerability assessment of karstic aquifer under semi-arid climatic conditions, case of Cheria Plateau. NE Algeria Arab J Geosci 13:709. https://doi.org/10.1007/s12517-020-05704-0
Grunwald S (2010) Current state of digital soil mapping and what is next. In: Boettinger JL, Howell DW, Moore AC, Hartemink AE, Kienast-Brown S (eds) Digital soil mapping. Progress in soil science, vol 2. Springer, Dordrecht. https://doi.org/10.1007/978-90-481-8863-5_1
Govt. of Satara. (2017) Govt. of Maharashtra Satara District official website: Government of Maharashtra
Beck HE, Zimmermann NE, McVicar TR, Vergopolan N, Berg A, Wood EF (2018) Present and future Köppen-Geiger climate classification maps at 1-km resolution. Scientific Data 5:180214. https://doi.org/10.1038/sdata.2018.214
UNESCO (2017) World heritage centre. http://whc.unesco.org/en/list/1342/multiple=1&unique_number=1921. Accessed 24 Nov 2017
ENVIS (2013) ENVIS Newsletter June–September, Mumbai
Gajbhiye KSMC (2000) Agro-ecological zones, their soil resource and cropping systems. Status of farm mechanization in India. https://krishi.icar.gov.in/jspui/bitstream/123456789/32452/1/Tree-based%20farming%20systems%20for%20different%20agro-eco-sub%20regions%20of%20Andhra%20Pradesh.pdf
GSI (2000) District resource map-Satara District, Maharashtra, Geological survey of India, 1:2,50,000
NBSS & LUP (1996) Soil map of Maharashtra state, scale 1:500,000. National Bureau of Soil Survey and Land Use Planning (NBSS & LUP, Nagpur)
Rajapaksha RPSK, Karunaratne SB, Biswas A, Paul K, Madawala HMSP, Gunathilake SK, Ratnayake RR (2020) Identifying the spatial drivers and scale-specific variations of soil organic carbon in tropical ecosystems: a case study from Knuckles Forest Reserve in Sri Lanka. For Ecol Manag 474:118285. https://doi.org/10.1016/j.foreco.2020.118285
Chandra Nath P, Nath AJ, Reang D, Lal R, Das AK (2021) Tree diversity, soil organic carbon lability and ecosystem carbon storage under a fallow age chronosequence in North East India. Environ Sustain Indic 10:100122. https://doi.org/10.1016/j.indic.2021.100122
Buchhorn M , Smets B, Bertels L, De Roo B, Lesiv M, Tsendbazar, N-E, Herold M, Fritz S (2020) Copernicus global land service: land cover 100 m: collection 3: epoch 2019: Globe 2020. 10.5281/zenodo.3939050
Pennock D, Yates TBJ (2007) Soil sampling and methods of analysis. Second, Boca Raton
FAO (2006) Guidelines for soil description, 4th edn. https://www.fao.org/3/a0541e/a0541e.pdf
Walkley A, Black IA (1934) An examination of the degtjareff method for determining soil organic matter, and a proposed modification of the chromic acid titration method. Soil Sci 37:29–38. https://doi.org/10.1097/00010694-193401000-00003
Bhunia GS, Shit PK, Maiti R (2018) Comparison of GIS-based interpolation methods for spatial distribution of soil organic carbon (SOC). J Saudi Soc Agric Sci 17:114–126. https://doi.org/10.1016/j.jssas.2016.02.001
Li B, Griffith DA, Becker B (2016) Spatially simplified scatterplots for large raster datasets. Geo-spatial Inf Sci 19:81–93. https://doi.org/10.1080/10095020.2016.1179441
Conrad O, Bechtel B, Bock M, Dietrich H, Fischer E, Gerlitz L, Wehberg J, Wichmann V, Böhner J (2015) System for automated geoscientific analyses (SAGA) v. 2.1.4. Geosci Model Develop 8:1991–2007. https://doi.org/10.5194/gmd-8-1991-2015
Yang Y, Chen Y, Li W, Chen Y (2010) Distribution of soil organic carbon under different vegetation zones in the Ili River Valley Xinjiang. J Geogr Sci. https://doi.org/10.1007/s11442-010-0807-4
Batjes NH (1996) Total carbon and nitrogen in the soils of the world. Eur J Soil Sci. https://doi.org/10.1111/j.1365-2389.1996.tb01386.x
Guo LB, Gifford RM (2002) Soil carbon stocks and land use change: a meta analysis. Glob Change Biol 8:345–360. https://doi.org/10.1046/j.1354-1013.2002.00486.x
Yigini Y, Panagos P (2016) Assessment of soil organic carbon stocks under future climate and land cover changes in Europe. Sci Total Environ 557–558:838–850. https://doi.org/10.1016/j.scitotenv.2016.03.085
Zheng Y, Yu G (2017) Spatio-temporal distribution of vegetation index and its influencing factors—a case study of the Jiaozhou Bay, China. Chin J Ocean Limnol 35:1398–1408. https://doi.org/10.1007/s00343-017-6062-0
Environment Agency (2015) Factors that influence erosion and runoff. https://projectblue.blob.core.windows.net/media/Default/Imported%20Publication%20Docs/ThinkSoils.pdf
Bellè S-L, Riotte J, Sekhar M, Ruiz L, Schiedung M, Abiven S (2022) Soil organic carbon stocks and quality in small-scale tropical, sub-humid and semi-arid watersheds under shrubland and dry deciduous forest in southwestern India. Geoderma 409:115606. https://doi.org/10.1016/j.geoderma.2021.115606
Gebrehiwot K, Desalegn T, Woldu Z, Demissew S, Teferi E (2018) Soil organic carbon stock in Abune Yosef afroalpine and sub-afroalpine vegetation, northern Ethiopia. Ecol Process 7:6. https://doi.org/10.1186/s13717-018-0117-9
Horwath WR, Kuzyakov Y (2018) Chapter Three - The potential for soils to mitigate climate change through carbon sequestration. In: Horwath WR, Kuzyakov Y (eds) Climate change impacts on soil processes and ecosystem properties. Elsevier, London, pp 61–92
Franzluebbers AJ (2013) Pursuing robust agroecosystem functioning through effective soil organic carbon management. Carbon Manag 4:43–56. https://doi.org/10.4155/cmt.12.78
Sharma G, Sharma LK, Sharma KC (2019) Assessment of land use change and its effect on soil carbon stock using multitemporal satellite data in semiarid region of Rajasthan. India Ecol Process 8:42. https://doi.org/10.1186/s13717-019-0193-5
Ravula R, Chiranjeeva Reddy M, Mhaiskar P (2022) Effect of eucalyptus plantations on soil properties in different land use patterns in central Telangana zone. Pharma Innov J 11:16–20
Tererai F, Gaertner M, Jacobs SM, Richardson DM (2015) Eucalyptus Camaldulensis invasion in riparian zones reveals few significant effects on soil physico-chemical properties: effects of invasive eucalyptus on soil properties. River Res Appl 31:590–601. https://doi.org/10.1002/rra.2762
Wiesmeier M, Urbanski L, Hobley E, Lang B, Von Lützow M, Marin-Spiotta E, Van Wesemael B, Rabot E, Ließ M, Garcia-Franco N, Wollschläger U, Vogel H-J, Kögel-Knabner I (2019) Soil organic carbon storage as a key function of soils—a review of drivers and indicators at various scales. Geoderma 333:149–162. https://doi.org/10.1016/j.geoderma.2018.07.026
Brahmi S, Baali F, Hadji R, Brahmi S, Hamad A, Rahal O, Zerrouki H, Saadali B, Hamed Y (2021) Assessment of groundwater and soil pollution by leachate using electrical resistivity and induced polarization imaging survey, case of Tebessa municipal landfill. NE Algeria Arab J Geosci 14:249. https://doi.org/10.1007/s12517-021-06571-z
Acknowledgements
We are thankful to the Copernicus Global Land Service portal for providing land cover data and Bhuvan portal for providing Cartosat DEM. All authors are thankful to anonymous reviewers and editor for their constructive comments on the submitted manuscript, which helped in the improvement of the current version of the revised manuscript.
Funding
Not received.
Author information
Authors and Affiliations
Contributions
Wasim Ayub Bagwan: Conceived and designed the research theme, carried out soil sampling, laboratory experiment, statistical analysis, GIS mapping and wrote manuscript, revision, Ravindra Sopan Gavali: supervised the design and suggested in writing of manuscript. Ashis Maity: provided soil laboratory facility at National Research Centre on Pomegranate, Solapur and has observed the reading of soil organic matter experimentation. All authors discussed the results and commented on the manuscript. All authors read and approved the final manuscript.
Corresponding author
Ethics declarations
Conflict of interest
The authors declare that they have no known competing financial interests or personal relationships that could have appeared to influence the work carried out in this research.
Ethics approval and consent to participate
Not applicable.
Consent for publication
All authors declare that they are consent for publication in the Journal of Umm Al-Qura University for Applied Sciences.
Additional information
Publisher's Note
Springer Nature remains neutral with regard to jurisdictional claims in published maps and institutional affiliations.
Supplementary Information
Below is the link to the electronic supplementary material.
Rights and permissions
Open Access This article is licensed under a Creative Commons Attribution 4.0 International License, which permits use, sharing, adaptation, distribution and reproduction in any medium or format, as long as you give appropriate credit to the original author(s) and the source, provide a link to the Creative Commons licence, and indicate if changes were made. The images or other third party material in this article are included in the article's Creative Commons licence, unless indicated otherwise in a credit line to the material. If material is not included in the article's Creative Commons licence and your intended use is not permitted by statutory regulation or exceeds the permitted use, you will need to obtain permission directly from the copyright holder. To view a copy of this licence, visit http://creativecommons.org/licenses/by/4.0/.
About this article
Cite this article
Bagwan, W.A., Gavali, R.S. & Maity, A. Quantifying soil organic carbon (SOC) density and stock in the Urmodi River watershed of Maharashtra, India: implications for sustainable land management. J.Umm Al-Qura Univ. Appll. Sci. 9, 548–564 (2023). https://doi.org/10.1007/s43994-023-00064-3
Received:
Accepted:
Published:
Issue Date:
DOI: https://doi.org/10.1007/s43994-023-00064-3